Chapter 11: LCA-Driven Solutions to Combat Urban Pollution PDF
Document Details
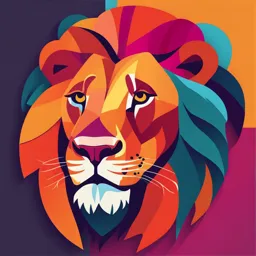
Uploaded by IngeniousCthulhu
Amir Hamzah Sharaai
Tags
Summary
This chapter examines LCA methodologies to address urban air pollution, highlighting the lifecycle impacts of pollution control measures. A framework for integrating LCA into urban air quality management and policy is proposed. The research draws upon literature, case studies, and empirical data.
Full Transcript
# Cradle to Grave ## Life Cycle Thinking for a Sustainable Future ### Amir Hamzah Sharaai # Chapter 11: Clearing the Air: LCA-Driven Solutions to Combat Urban Pollution ## Abstract This comprehensive study explores the application of Life Cycle Assessment (LCA) methodologies in developing and eval...
# Cradle to Grave ## Life Cycle Thinking for a Sustainable Future ### Amir Hamzah Sharaai # Chapter 11: Clearing the Air: LCA-Driven Solutions to Combat Urban Pollution ## Abstract This comprehensive study explores the application of Life Cycle Assessment (LCA) methodologies in developing and evaluating solutions to combat urban air pollution. As cities worldwide grapple with poor air quality, there is a growing need for holistic approaches to assess and implement effective pollution mitigation strategies. This paper examines how LCA may provide crucial insights into the full environmental implications of various air pollution control measures, enabling more informed decision-making in urban environmental management. Through an extensive review of literature, case studies, and empirical data, this research demonstrates the potential of LCA to inform more effective air quality management strategies in urban areas. The study reveals that while certain pollution control measures may have obvious local benefits, their overall environmental impact can be complex and sometimes counterintuitive when assessed from a lifecycle perspective. The article proposes a framework for integrating LCA into urban air quality management, explores applications across different sectors contributing to urban pollution, and discusses the implications for policy and urban planning. It also examines the role of emerging technologies and innovative approaches in enhancing the effectiveness of LCA-driven solutions. The paper concludes by outlining key research priorities and policy recommendations to advance the use of LCA in combating urban air pollution. ## Keywords: * Urban Air Pollution * Life Cycle Assessment (LCA) * Environmental Impact * Sustainable Cities * Air Quality Management * Green Technologies * Environmental Policy * Public Health * Climate Change Mitigation * Sustainable Urban Planning # 1. Introduction Urban air pollution remains one of the most pressing environmental challenges of the 21st century, with significant implications for public health, quality of life, and climate change. As cities continue to grow and densify, the need for effective strategies to combat air pollution becomes increasingly urgent. Life Cycle Assessment (LCA) offers a powerful tool for evaluating the comprehensive environmental impacts of various pollution control measures, enabling policymakers and urban planners to make more informed decisions. ## 1.1 Background and Context The exploration of LCA-driven solutions for urban air pollution is motivated by several factors: 1. Persistent Urban Air Quality Issues. Despite improvements in many cities, air pollution remains a significant problem, with 90% of urban dwellers breathing air that exceeds WHO guidelines (WHO, 2021). 2. Complex Pollution Sources. Urban air pollution stems from multiple sources, including transportation, industry, energy production, and residential activities (EEA, 2020). 3. Interconnected Environmental Challenges. Air pollution is closely linked to other environmental issues such as climate change and resource depletion (IPCC, 2021). 4. Technological Advancements. Emerging green technologies offer new possibilities for pollution control but require careful evaluation of their overall environmental impact (Shaikh et al., 2021). 5. Policy Integration. Growing recognition of the need to integrate air quality management with broader sustainability and climate change mitigation efforts (C40 Cities, 2020). ## 1.2 Research Objectives This study addresses several key research questions: 1. How can LCA methodologies be effectively applied to evaluate and compare different urban air pollution control strategies? 2. What are the main environmental trade-offs and synergies associated with various air quality improvement measures when analyzed from a lifecycle perspective? 3. How do the lifecycle impacts of pollution control technologies vary across different urban contexts and geographical regions? 4. What role can LCA play in informing more effective policies and strategies for urban air quality management? 5. How can LCA insights be integrated into urban planning and decision-making processes to promote sustainable air quality improvements? 6. What are the key challenges in applying LCA to complex urban systems, and how can these be addressed? 7. How do LCA-driven solutions for air pollution align with or conflict with other urban sustainability objectives? 8. What emerging technologies and innovative approaches show promise for improving urban air quality when evaluated through an LCA lens? 9. How can LCA be used to assess the co-benefits of air pollution control measures, such as climate change mitigation and public health improvements? 10. What should be the priorities for future research and technological development in this field? ## 1.3 Methodology To answer these questions, this article employs a mixed-methods approach, drawing on a wide range of sources: 1. Literature Review. An extensive review of peer-reviewed academic literature, technical reports, and policy documents on urban air pollution, LCA methodologies, and related fields. 2. Case Studies. Analysis of LCA studies conducted on various air pollution control measures and technologies across different urban contexts. 3. Data Analysis. Examination of air quality data, emissions inventories, and LCA databases relevant to urban pollution control. 4. Expert Interviews. Insights from interviews with environmental scientists, urban planners, policymakers, and LCA practitioners working on urban air quality issues. 5. Policy Analysis. Review of air quality policies and regulations at local, national, and international levels. 6. Modeling Studies. Examination of air quality modeling approaches integrated with LCA to assess the impacts of different pollution control scenarios. This comprehensive approach allows for a holistic examination of the potential for LCA to inform and shape more effective strategies for combating urban air pollution. # 2. Theoretical Foundations ## 2.1 Life Cycle Assessment (LCA) Methodology LCA is a standardized method for assessing environmental impacts associated with all stages of a product's life, from raw material extraction through materials processing, manufacture, distribution, use, repair and maintenance, and disposal or recycling (ISO 14040, 2006). Key stages in an LCA include: 1. Goal and Scope Definition. Defining the purpose of the study and system boundaries. 2. Inventory Analysis. Collecting data on inputs and outputs for all processes within the system boundaries. 3. Impact Assessment. Evaluating the potential environmental impacts associated with the identified inputs and outputs. 4. Interpretation. Analyzing results and making recommendations based on the findings. ## 2.2 Urban Air Pollution: Sources and Impacts Understanding the complex nature of urban air pollution is crucial for developing effective LCA-driven solutions: ## 2.2.1 Major Pollutants Key air pollutants in urban environments include: * Particulate Matter (PM2.5 and PM10) * Nitrogen Oxides (NOx) * Sulfur Dioxide (SO2) * Ozone (O3) * Carbon Monoxide (CO) * Volatile Organic Compounds (VOCs) ## 2.2.2 Pollution Sources Primary sources of urban air pollution: * Transportation (vehicle emissions) * Industrial activities * Energy production and consumption * Residential heating and cooking * Construction and demolition activities ## 2.2.3 Health and Environmental Impacts Consequences of urban air pollution: * Respiratory and cardiovascular diseases * Premature mortality * Reduced cognitive function * Ecosystem damage * Climate change contributions ## 2.3 LCA Applications in Urban Air Quality Management LCA can be applied to various aspects of urban air quality management: ## 2.3.1 Technology Assessment Evaluating the overall environmental impact of pollution control technologies: * End-of-pipe solutions (e.g., catalytic converters, air purifiers) * Clean energy technologies (e.g., solar panels, wind turbines) * Green infrastructure (e.g., urban forests, green roofs) ## 2.3.2 Policy Evaluation Assessing the lifecycle implications of different policy measures: * Vehicle emission standards * Low emission zones * Congestion charging schemes * Industrial emission regulations ## 2.3.3 Urban Planning Informing sustainable urban development strategies: * Transit-oriented development * Mixed-use zoning * Green space allocation * Sustainable transportation infrastructure # 3. LCA-Driven Solutions Across Urban Sectors This section explores how LCA can inform air pollution control strategies in various urban sectors, highlighting key findings and implications. ## 3.1 Transportation Sector ## 3.1.1 Electric Vehicles (EVs) LCA considerations in transitioning to electric mobility: * Benefits: Reduced tailpipe emissions, potential for lower lifecycle GHG emissions (Hawkins et al., 2013). * Challenges: Environmental impacts of battery production, electricity source dependency (Bicer & Dincer, 2017). * LCA Insights: Importance of clean electricity grids and battery recycling for maximizing EV benefits (Ellingsen et al., 2017). ## 3.1.2 Public Transportation Lifecycle assessment of public transit systems: * Benefits: Potential for significant per-passenger emission reductions (Chester et al., 2013). * Challenges: High infrastructure-related emissions, variability in ridership (Ercan & Tatari, 2015). * LCA Insights: Importance of high occupancy rates and low-carbon construction methods (Nahlik & Chester, 2014). ## 3.1.3 Active Transportation Infrastructure Evaluating cycling and walking infrastructure: * Benefits: Near-zero operational emissions, health co-benefits (Rojas-Rueda et al., 2011). * Challenges: Emissions associated with infrastructure construction (Helbig et al., 2016). * LCA Insights: Long-term benefits outweigh initial environmental costs, importance of durable materials (Mrkajic et al., 2018). ## 3.2 Energy Sector ## 3.2.1 Renewable Energy Technologies LCA of clean energy solutions for urban areas: * Solar PV: Variability in impacts based on location and technology (Louwen et al., 2016). * Wind Power: Generally favorable lifecycle impacts, concerns about resource use (Arvesen & Hertwich, 2012). * LCA Insights: Importance of recycling and circular economy approaches for renewable technologies (Hertwich et al., 2015). ## 3.2.2 District Heating and Cooling Assessing centralized energy systems: * Benefits: Potential for high efficiency and integration of renewable sources (Ghafghazi et al., 2011). * Challenges: Infrastructure-related emissions, fuel source dependency (Eriksson et al., 2007). * LCA Insights: Importance of system design and fuel mix optimization (Titus et al., 2019). ## 3.3 Buildings and Construction ## 3.3.1 Green Building Materials Lifecycle assessment of sustainable construction materials: * Benefits: Potential for reduced embodied energy and emissions (Bribián et al., 2011). * Challenges: Variability in performance, potential for unintended consequences (Ingrao et al., 2016). * LCA Insights: Importance of considering local climate and building lifespan (Säynäjoki et al., 2017). ## 3.3.2 Energy Efficiency Measures Evaluating building energy retrofits: * Benefits: Significant potential for reducing operational emissions (Famuyibo et al., 2013) * Challenges: Embodied emissions of retrofit materials, potential rebound effects (Vilches et al., 2017). * LCA Insights: Importance of holistic assessment including user behavior (Malmqvist et al., 2018). ## 3.4 Urban Greening and Nature-Based Solutions ## 3.4.1 Urban Forests and Green Spaces LCA of urban vegetation strategies: * Benefits: Air pollutant removal, carbon sequestration, cooling effects (Nowak et al., 2018). * Challenges: Water consumption, maintenance-related emissions (Strohbach et al., 2012). * LCA Insights: Long-term benefits generally outweigh costs, species selection crucial (Russo et al., 2016). ## 3.4.2 Green Infrastructure Assessing green roofs, walls, and bioswales: * Benefits: Localized air quality improvements, stormwater management, energy savings (Pugh et al., 2012). * Challenges: Material and maintenance impacts, context-dependent effectiveness (Bozorg Chenani et al., 2015). * LCA Insights: Importance of integrated design for multiple benefits (Kosareo & Ries, 2007). # 4. Challenges in Applying LCA to Urban Air Quality Solutions ## 4.1 Methodological Challenges ## 4.1.1 System Boundary Definition Defining appropriate boundaries for urban LCA studies: * Challenge: Complexity of urban systems and interconnected pollution sources. * Potential Solution: Development of standardized frameworks for urban air quality LCAs (Loiseau et al., 2018). ## 4.1.2 Data Availability and Quality Ensuring sufficient and reliable data for comprehensive assessments: * Challenge: Limited data on long-term performance and real-world emissions of new technologies. * Potential Solution: Establishment of urban environmental observatories and data sharing initiatives (Mirabella et al., 2018). ## 4.2 Temporal and Spatial Considerations ## 4.2.1 Long-term Impacts Addressing the long lifespans of urban infrastructure: * Challenge: Uncertainty in future energy mixes, technological developments, and climate conditions. * Potential Solution: Development of dynamic LCA approaches that can account for changing conditions over time (Collinge et al., 2013). ## 4.2.2 Spatial Variability Accounting for location-specific factors in urban environments: * Challenge: High variability in pollution levels, population exposure, and environmental conditions within cities. * Potential Solution: Integration of GIS and spatial analysis techniques with LCA (Maier et al., 2017). ## 4.3 Multi-criteria Decision Making ## 4.3.1 Trade-off Analysis Balancing multiple environmental and social objectives: * Challenge: Potential conflicts between air quality improvements and other sustainability goals. * Potential Solution: Development of multi-criteria decision analysis frameworks that incorporate LCA results (Zanghelini et al., 2018). ## 4.3.2 Uncertainty Management Dealing with uncertainties in LCA results: * Challenge: High uncertainty in long-term projections and complex urban systems. * Potential Solution: Use of probabilistic approaches and sensitivity analyses in urban LCAs (Gregory et al., 2016). # 5. Innovative Approaches and Future Directions ## 5.1 Technological Innovations ## 5.1.1 Internet of Things (IoT) and Big Data Leveraging advanced data collection and analysis: * Opportunity: Real-time monitoring and adaptive management of urban air quality. * Future Direction: Integration of IoT data streams into dynamic urban LCA models (Bibri & Krogstie, 2017). ## 5.1.2 Artificial Intelligence and Machine Learning Enhancing predictive capabilities and optimization: * Opportunity: Improved air quality forecasting and pollution control optimization. * Future Direction: Development of AI-powered decision support systems integrating LCA insights (Bellinger et al., 2021). ## 5.2 Policy and Governance Innovations ## 5.2.1 Integrated Urban Environmental Management Developing holistic approaches to urban sustainability: * Opportunity: Aligning air quality management with climate, energy, and health policies. * Future Direction: Creation of integrated assessment frameworks that combine LCA with other urban planning tools (Petit-Boix et al., 2017). ## 5.2.2 Participatory LCA Approaches Enhancing stakeholder engagement in decision-making: * Opportunity: Incorporating local knowledge and preferences into LCA-driven solutions. * Future Direction: Development of citizen science initiatives and participatory LCA methodologies (Mazzi et al., 2017). ## 5.3 Emerging Research Areas ## 5.3.1 Health Impact Integration Strengthening the link between LCA and health outcomes: * Opportunity: Quantifying the health benefits of air quality improvements in LCA studies. * Future Direction: Development of integrated LCA-HIA (Health Impact Assessment) frameworks (Stylianou et al., 2018). ## 5.3.2 Urban Metabolism and Circular Economy Applying systems thinking to urban air quality management: * Opportunity: Identifying synergies between air quality improvements and resource efficiency. * Future Direction: Integration of Material Flow Analysis with LCA for comprehensive urban environmental assessment (Goldstein et al., 2020). # 6. Conclusion ## 6.1 Key Findings This comprehensive review of LCA-driven solutions for urban air pollution has revealed several key insights: 1. Holistic Perspective: LCA provides a crucial systemic perspective that can enhance the effectiveness of air pollution control strategies by revealing hidden impacts and potential burden-shifting. 2. Technology Evaluation: LCA studies consistently highlight the importance of considering the full lifecycle impacts of pollution control technologies, including production, operation, and end-of-life. 3. Sector-Specific Insights: LCA reveals varying challenges and opportunities across different urban sectors, emphasizing the need for tailored approaches in transportation, energy, buildings, and urban greening. 4. Multi-dimensional Impacts: LCA-driven solutions often highlight co-benefits and trade-offs between air quality improvements and other environmental objectives, such as climate change mitigation and resource conservation. 5. Contextual Variability: The effectiveness and overall impact of air pollution control measures can vary significantly based on local conditions, underscoring the importance of city-specific assessments. 6. Technological Synergies: Emerging technologies in data analytics, IoT, and artificial intelligence offer significant potential to enhance the application and effectiveness of LCA in urban air quality management. 7. Policy Integration: LCA provides a valuable framework for integrating air quality considerations into broader urban sustainability policies and planning processes. 8. Methodological Challenges: While LCA offers powerful insights, significant challenges remain in terms of data availability, system boundary definition, and dealing with the complexity of urban systems. ## 6.2 Implications The findings of this study have significant implications for various stakeholders: 1. Urban Policymakers need to incorporate LCA thinking into air quality management strategies to ensure that pollution control measures deliver net positive environmental outcomes across their full lifecycle. 2. City Planners should use LCA insights to inform long-term urban development plans, considering the lifecycle impacts of infrastructure choices on air quality and overall urban sustainability. 3. Technology Developers can leverage LCA to guide innovation towards solutions that offer genuine environmental benefits when considering their full lifecycle impacts. 4. Environmental Agencies should consider adopting LCA-based evaluation criteria in regulatory frameworks and environmental impact assessments for urban development projects. 5. Researchers must continue to refine LCA methodologies for urban applications, addressing key challenges such as data gaps, spatial variability, and integration with other assessment tools. 6. Citizens and Community Organizations can use LCA-derived information to advocate for more effective and holistic air quality improvement strategies in their cities. ## 6.3 Future Outlook As cities worldwide continue to grapple with air pollution challenges, the role of LCA in informing effective solutions is likely to grow. Several trends are expected to shape the future of this field: 1. Integrated Assessments: Increasing integration of LCA with other urban planning and environmental assessment tools, providing more comprehensive insights for decision-makers. 2. Real-time LCA: Development of dynamic, real-time LCA capabilities enabled by IoT and big data analytics, allowing for more responsive and adaptive urban air quality management. 3. Circular Economy Integration: Greater emphasis on circular economy principles in urban air quality solutions, with LCA playing a key role in optimizing resource flows and minimizing waste. 4. Health-Centered Approaches: Stronger integration of health impact assessments with LCA, quantifying the public health benefits of air quality improvements more precisely. 5. Climate-Air Quality Nexus: Increased focus on solutions that simultaneously address air pollution and climate change, with LCA helping to identify and optimize these synergies. 6. Participatory Frameworks: Development of more inclusive, participatory LCA approaches that incorporate citizen science and local knowledge into urban air quality assessments. 7. Global-Local Connections: Enhanced understanding of the connections between local air quality actions and global environmental impacts, facilitated by advanced LCA modeling capabilities. In conclusion, LCA-driven solutions offer a promising path towards more effective and sustainable urban air quality management. By providing a comprehensive, lifecycle perspective on pollution control measures, LCA can guide cities towards strategies that deliver genuine environmental improvements without unintended consequences. As urban areas continue to grow and evolve, the application of LCA thinking will be crucial in creating cleaner, healthier, and more sustainable urban environments. The journey towards clean urban air is complex, requiring the integration of scientific insight, technological innovation, and thoughtful policy-making. LCA stands as a powerful tool in this endeavor, offering a systematic approach to evaluating and optimizing air quality solutions. Moving forward, continued research, methodological refinement, and practical application of LCA in urban contexts will be essential in our collective efforts to clear the air in cities around the world. # References * Arvesen, A., & Hertwich, E. G. (2012). Assessing the life cycle environmental impacts of wind power: A review of present knowledge and research needs. Renewable and Sustainable Energy Reviews, 16(8), 5994-6006. * Bellinger, C., et al. (2021). Artificial intelligence for environmental science: Applications and future directions. Environmental Research Letters, 16(6), 061001. * Bibri, S. E., & Krogstie, J. (2017). Smart sustainable cities of the future: An extensive interdisciplinary literature review. Sustainable Cities and Society, 31, 183-212. * Bicer, Y., & Dincer, I. (2017). Comparative life cycle assessment of hydrogen, methanol and electric vehicles from well to wheel. International Journal of Hydrogen Energy, 42(6), 3767-3777. * Bozorg Chenani, S., Lehvävirta, S., & Häkkinen, T. (2015). Life cycle assessment of layers of green roofs. Journal of Cleaner Production, 90, 153-162. * Bribián, I. Z., Capilla, A. V., & Usón, A. A. (2011). Life cycle assessment of building materials: Comparative analysis of energy and environmental impacts and evaluation of the eco-efficiency improvement potential. Building and Environment, 46(5), 1133-1140. * C40 Cities. (2020). Air Quality in Cities: C40 Cities Baseline Report. C40 Cities Climate Leadership Group. * Chester, M., et al. (2013). Infrastructure and automobile shifts: Positioning transit to reduce life-cycle environmental impacts for urban sustainability goals.. Environmental Research Letters, 8(1), 015041. * Collinge, W. O., et al. (2013). Dynamic life cycle assessment: Framework and: application to an institutional building. The International Journal of Life Cycle Assessment, 18(3), 538-552. * EEA. (2020). Air quality in Europe - 2020 report. European Environment Agency. * Ellingsen, L. A. W., et al. (2017). The size and range effect: Lifecycle greenhouse gas emissions of electric vehicles. Environmental Research Letters, 12(5), 054010. * Ercan, T., & Tatari, O. (2015). A hybrid life cycle assessment of public transportation buses with alternative fuel options. The International Journal of Life Cycle Assessment, 20(9), 1213-1231. * Eriksson, O., et al. (2007). Life cycle assessment of fuels for district heating: A comparison of waste incineration, biomass- and natural gas combustion. Energy Policy, 35(2), 1346-1362. * Famuyibo, A. A., Duffy, A., & Strachan, P. (2013). Achieving a holistic view of the life cycle performance of existing dwellings. Building and Environment, 70, 90-101. * Ghafghazi, S., et al. (2011). Life cycle assessment of base-load heat sources for district heating system options. The International Journal of Life Cycle Assessment, 16(3), 212-223. * Goldstein, B., et al. (2020). Surveying the environmental footprint of urban food consumption. Journal of Industrial Ecology, 24(3), 515-525. * Gregory, J. R., Noshadravan, A., Olivetti, E. A., & Kirchain, R. E. (2016). A methodology for robust comparative life cycle assessments incorporating uncertainty. Environmental Science & Technology, 50(12), 6397-6405. * Hawkins, T. R., et al. (2013). Comparative environmental life cycle assessment of conventional and electric vehicles. Journal of Industrial Ecology, 17(1), 53-64. * Helbig, C., et al. (2016). How to evaluate raw material vulnerability - An overview. Resources Policy, 48, 13-24. * Hertwich, E. G., et al. (2015). Integrated life-cycle assessment of electricity-supply scenarios confirms global environmental benefit of low-carbon technologies. Proceedings of the National Academy of Sciences, 112(20), 6277-6282. * Ingrao, C., et al. (2016). The potential use of life cycle assessment (LCA) in the assessment of environmental sustainability of bioplastic products: A review. Sustainability, 8(1), 118. * IPCC. (2021). Climate Change 2021: The Physical Science Basis. Contribution of Working Group I to the Sixth Assessment Report of the Intergovernmental Panel on Climate Change. Cambridge University Press. * ISO. (2006). ISO 14040:2006 Environmental management - Life cycle assessment - Principles and framework. International Organization for Standardization. * Kosareo, L., & Ries, R. (2007). Comparative environmental life cycle assessment of green roofs. Building and Environment, 42(7), 2606-2613. * Loiseau, E., et al. (2018). Green economy and related concepts: An overview. Journal of Cleaner Production, 182, 91-110. * Louwen, A., et al. (2016). Re-assessment of net energy production and greenhouse gas emissions avoidance after 40 years of photovoltaics development. Nature Communications, 7(1), 1-9. * Maier, M., Mueller, M., & Yan, X. (2017). Introducing a localised spatio-temporal LCI method with wheat production as exploratory case study. Journal of Cleaner Production, 140, 492-501. * Malmqvist, T., et al. (2018). Design and construction strategies for reducing embodied impacts from buildings - Case study analysis. Energy and Buildings, 166, 35-47. * Mazzi, A., et al. (2017). The combination of an Environmental Management System and Life Cycle Assessment at the territorial level. Environmental Impact Assessment Review, 63, 59-71. * Mirabella, N., Allacker, K., & Sala, S. (2018). Current trends and limitations of life cycle assessment applied to the urban scale: Critical analysis and review of selected literature. The International Journal of Life Cycle Assessment, 23(12), 2439-2453. * Mrkajic, V., et al. (2018). Life cycle assessment of a bicycle sharing system: Case study in Novi Sad (Serbia). Sustainable Cities and Society, 37, 611-618. * Nahlik, M. J., & Chester, M. V. (2014). Transit-oriented smart growth can reduce life-cycle environmental impacts and household costs in Los Angeles. Transport Policy, 35, 21-30. * Nowak, D. J., et al. (2018). Air pollution removal by urban forests in Canada and its effect on air quality and human health. Urban Forestry & Urban Greening, 29, 40-48. * Petit-Boix, A., et al. (2017). Application of life cycle thinking towards sustainable cities: A review. Journal of Cleaner Production, 166, 939-951. * Pugh, T. A., et al. (2012). Effectiveness of green infrastructure for improvement of air quality in urban street canyons. Environmental Science & Technology, 46(14), 7692-7699. * Rojas-Rueda, D., et al. (2011). The health risks and benefits of cycling in urban environments compared with car use: Health impact assessment study. BMJ, 343, d4521. * Russo, A., et al. (2016). Edible green infrastructure: An approach and review of provisioning ecosystem services and disservices in urban environments. Agriculture, Ecosystems & Environment, 242, 53-66. * Säynäjoki, A., et al. (2017). Can life-cycle assessment produce reliable policy guidelines in the building sector? Environmental Research Letters, 12(1), 013001. * Shaikh, P. H., et al. (2021). Building energy efficiency: A review of classification and evaluation methods. Sustainability, 13(13), 7245. * Strohbach, M. W., Arnold, E., & Haase, D. (2012). The carbon footprint of urban green space-A life cycle approach. Landscape and Urban Planning, 104(2), 220-229. * Stylianou, K. S., et al. (2018). A life cycle assessment framework combining nutritional and environmental health impacts of diet: A case study on milk. The International Journal of Life Cycle Assessment, 23(1), 136-149. * Titus, E., et al. (2019). Life cycle assessment of district heating systems in Sweden: Integration of industrial excess heat. Applied Energy, 255, 113833. * Vilches, A., Garcia-Martinez, A., & Sanchez-Montañes, B. (2017). Life cycle assessment (LCA) of building refurbishment: A literature review. Energy and Buildings, 135, 286-301. * WHO. (2021). WHO global air quality guidelines: Particulate matter (PM2.5 and: PM10), ozone, nitrogen dioxide, sulfur dioxide and carbon monoxide. World Health Organization. * Zanghelini, G. M., et al. (2018). Systematic review of life cycle assessment (LCA) for Comparative studies in the housing sector. Journal of Cleaner Production, 186, 411-422.