Biochemistry Chapter 13 Study Guide PDF
Document Details
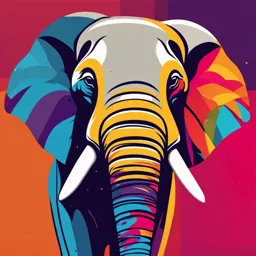
Uploaded by MatureGyrolite4296
Loyola Marymount University
Tags
Summary
This study guide provides an overview of chapter 13 in a biochemistry textbook. It covers topics such as autotrophs, heterotrophs, and an introduction to bioenergetics and thermodynamics. The guide also explains key concepts like catabolism, anabolism, and pathways in biological systems.
Full Transcript
Study Guide for Biochemistry: Chapter 13 Topics Autotrophs and Heterotrophs Autotrophs: Organisms that use atmospheric CO2 as their sole carbon source (e.g., plants). Heterotrophs: Organisms that must obtain carbon from their environment (e.g., animals, fungi). Metabolit...
Study Guide for Biochemistry: Chapter 13 Topics Autotrophs and Heterotrophs Autotrophs: Organisms that use atmospheric CO2 as their sole carbon source (e.g., plants). Heterotrophs: Organisms that must obtain carbon from their environment (e.g., animals, fungi). Metabolites and Intermediary Metabolism Metabolites: Small intermediates in pathways that convert precursors to products. Intermediary Metabolism: The network of pathways involved in metabolite interconversions. Catabolism and Anabolism Catabolism: Breakdown of molecules; releases energy (exergonic). Anabolism: Synthesis of molecules; requires energy (endergonic). Pathway dynamics: ○ Catabolic pathways converge. ○ Anabolic pathways diverge. 13.1 Bioenergetics and Thermodynamics Key Concepts Bioenergetics: Study of energy transformations in biological systems. Energy Transductions: Conversion of energy forms. Laws of Thermodynamics 1. First Law: Energy is conserved. 2. Second Law: Systems naturally progress toward increased disorder (entropy). Important Thermodynamic Terms Free Energy (G): Energy available for work. ○ ∆G < 0: Exergonic (releases energy, spontaneous). ○ ∆G > 0: Endergonic (requires energy). Enthalpy (H): Heat content. ○ ∆H < 0: Exothermic (releases heat). ○ ∆H > 0: Endothermic (absorbs heat). Entropy (S): Measure of disorder. ○ ∆S > 0: Increased disorder. ○ ∆S < 0: Decreased disorder. Relationships Free energy equation: ΔG=ΔH−TΔSΔG=ΔH−TΔS. K′eq and ∆G′°: ○ ΔG′°=−RTlnK′eqΔG′°=−RTlnK′eq ○ K′eq>1.0K′eq>1.0: Reaction proceeds forward (ΔG′°0) can occur when paired with highly favorable reactions (e.g., ATP hydrolysis). Chemical Logic in Metabolic Reactions Common Reaction Types 1. Making/Breaking Carbon–Carbon Bonds: ○ Examples: Aldol condensation, Claisen condensation, decarboxylation. 2. Internal Rearrangements, Isomerizations, and Eliminations: ○ Electron redistribution; examples include cis-trans isomerization. 3. Group Transfer Reactions: ○ Examples: Phosphorylation by kinases. 4. Oxidation-Reduction Reactions: ○ Involve transfer of electrons, often releasing energy. Key Enzyme Types Kinases: Transfer phosphate groups. Oxidases: Use oxygen as an electron acceptor. Dehydrogenases: Catalyze oxidation-reduction reactions. 13.3 Phosphoryl Group Transfers and ATP ATP as Energy Currency Links energy release from catabolism to energy requirements of anabolism. Hydrolysis of ATP: ○ Releases energy (ΔG′°=−30.5 kJ/molΔG′°=−30.5kJ/mol). ○ Reduces electrostatic repulsion in ATP. Other High-Energy Compounds Examples: ○ Phosphoenolpyruvate (ΔG′°=−61.9 kJ/molΔG′°=−61.9kJ/mol). ○ 1,3-Bisphosphoglycerate (ΔG′°=−49.3 kJ/molΔG′°=−49.3kJ/mol). Key Concepts to Focus On 1. Understand the thermodynamic principles governing metabolism. 2. Memorize relationships between K′eqK′eq, ΔG′°ΔG′°, and reaction direction. 3. Recognize the role of ATP in coupling reactions. 4. Be familiar with enzyme functions and common reaction mechanisms in metaboli Study Guide: Chapter 13 - Metabolism and Bioenergetics Cellular ATP Levels ATP Regulation: Cellular [ATP] is maintained above equilibrium to drive metabolic reactions effectively. ○ When [ATP] drops: Available fuel decreases. Fuel's effectiveness reduces. Phosphorylated Compounds and Thioesters High-Energy Compounds: Phosphorylated compounds have negative ∆G′° due to resonance stabilization of Pi. Examples of High-Energy Hydrolysis: ○ Phosphoenolpyruvate: ΔG′°=−61.9 kJ/molΔG′°=−61.9kJ/mol. ○ 1,3-Bisphosphoglycerate: ΔG′°=−49.3 kJ/molΔG′°=−49.3kJ/mol. ○ ATP (to ADP + Pi): ΔG′°=−30.5 kJ/molΔG′°=−30.5kJ/mol. Thioesters: ○ Less resonance stabilization compared to oxygen esters, yielding higher free-energy differences. ATP's Role in Metabolism 1. Energy Provider: ATP activates substrates via group transfers. 2. Muscle Contraction and Biosynthesis: ○ Drives processes like DNA/RNA/protein synthesis and ion transport. 3. Transphosphorylations: ○ ATP transfers phosphates to nucleotides (e.g., GTP, UTP) via nucleoside diphosphate kinase. 13.4 Biological Oxidation-Reduction Reactions Electron Flow in Oxidation-Reduction Purpose: Drives all biological work. Electromotive Force (emf): Proportional to the difference in electron affinity between species. Pathway: Electrons move from substrates to electron carriers, then to final acceptors like O2O2. Key Concepts Half-Reactions: Oxidation and reduction are described separately (e.g., Fe2+→Fe3++e−Fe2+→Fe3++e−). Redox Pairs: ○ Reducing Agent: Donates electrons. ○ Oxidizing Agent: Accepts electrons. Biological Redox Examples Dehydrogenation: Catalyzed by dehydrogenases, transferring electrons as: ○ Free electrons. ○ Hydrogen atoms. ○ Hydride ions (:H−:H−). Reduction Potentials (E°) Positive E°E°: High affinity for electrons (oxidizing). Negative E°E°: Donates electrons (reducing). Electron Carriers and Coenzymes 1. NAD and NADP: ○ Reversible reduction of the nicotinamide ring. ○ Example: NAD++2e−+2H+→NADH+H+NAD++2e−+2H+→NADH+H+. 2. FMN and FAD: ○ Derived from riboflavin. ○ Can accept one or two electrons during reduction. Niacin Deficiency Causes: Lack of NAD/NADP synthesis leads to pellagra (dermatitis, diarrhea, dementia). Energetics of Hydrolysis ATP Hydrolysis: Produces PiPi, PPiPPi, or AMP and releases energy. Group Transfers: ○ Coupled to anabolic reactions to overcome unfavorable ∆G′°. ○ Allows synthesis of molecules like DNA and proteins. Flavin Nucleotides in Flavoproteins Derived from riboflavin (vitamin B2). Mediate oxidation-reduction reactions as tightly bound cofactors. Essential Equations 1. Free Energy: ΔG=ΔH−TΔSΔG=ΔH−TΔS. 2. Reduction Potential (Simplified Nernst):E=E°+0.026nln[acceptor][donor].E=E°+n0.026ln[donor][acceptor]. Key Takeaways ATP and related compounds are central to cellular energy transfer. Oxidation-reduction reactions provide the energy for ATP synthesis. Cofactors like NAD, NADP, FAD, and FMN are universal electron carriers facilitating redox processes. Study Guide: Chapter 14 - Glycolysis, Gluconeogenesis, and the Pentose Phosphate Pathway 1. Major Pathways of Glucose Utilization Glycolysis: Converts glucose into pyruvate while producing ATP and NADH. Gluconeogenesis: Synthesizes glucose from non-carbohydrate precursors. Pentose Phosphate Pathway: Produces NADPH and ribose-5-phosphate for biosynthetic reactions. 2. Overview of Glycolysis Definition: A 10-step metabolic pathway that breaks down glucose into two molecules of pyruvate. Phases: 1. Preparatory Phase: Consumes 2 ATP. Converts glucose to glyceraldehyde-3-phosphate (G3P). 2. Payoff Phase: Generates 4 ATP and 2 NADH. Yields 2 pyruvate molecules. 3. Glycolysis Pathway Details Preparatory Phase Steps: 1. Phosphorylation of Glucose: ○ Enzyme: Hexokinase. ○ Produces glucose-6-phosphate (G6P); ATP is consumed. 2. Isomerization of G6P to F6P: ○ Enzyme: Phosphohexose Isomerase. 3. Phosphorylation of F6P to Fructose-1,6-bisphosphate (F1,6BP): ○ Enzyme: Phosphofructokinase-1 (PFK-1). ○ Key regulatory step (irreversible). 4. Cleavage of F1,6BP: ○ Enzyme: Aldolase. ○ Produces glyceraldehyde-3-phosphate (G3P) and dihydroxyacetone phosphate (DHAP). 5. Interconversion of DHAP to G3P: ○ Enzyme: Triose Phosphate Isomerase. Payoff Phase Steps: 6. Oxidation of G3P to 1,3-bisphosphoglycerate (1,3BPG): ○ Enzyme: G3P Dehydrogenase. ○ Produces NADH. 7. Phosphoryl Transfer from 1,3BPG to ADP: ○ Enzyme: Phosphoglycerate Kinase. ○ Produces ATP (substrate-level phosphorylation). 8. Conversion of 3-Phosphoglycerate to 2-Phosphoglycerate: ○ Enzyme: Phosphoglycerate Mutase. 9. Dehydration of 2-Phosphoglycerate to Phosphoenolpyruvate (PEP): ○ Enzyme: Enolase. 10. Transfer of Phosphoryl Group from PEP to ADP: ○ Enzyme: Pyruvate Kinase. ○ Produces ATP and pyruvate. 4. Chemical Transformations in Glycolysis 1. Degradation of Glucose to Pyruvate: ○ Carbon skeleton broken down. 2. ATP Synthesis via Substrate-Level Phosphorylation: ○ High-energy intermediates transfer phosphoryl groups to ADP. 3. NADH Formation: ○ G3P oxidation reduces NAD⁺ to NADH. 5. Energetics of Glycolysis Overall Reaction:Glucose+2 NAD++2 ADP+2 Pi→2 Pyruvate+2 NADH+2 ATP+2 H2OGlucose+2 NAD++2ADP+2Pi→2Pyruvate+2NADH+2ATP+2H2O Net ATP Gain: 2 ATP (4 produced − 2 consumed). Free Energy: ○ Overall ΔG′°=−85 kJ/molΔG′°=−85kJ/mol, making glycolysis essentially irreversible. 6. Importance of Phosphorylated Intermediates Functions: ○ Prevents intermediates from diffusing out of the cell. ○ Conserves energy via phosphoryl transfer. ○ Lowers activation energy for enzymatic reactions. 7. Pyruvate Fate Aerobic Conditions: ○ Converted to acetyl-CoA → Citric Acid Cycle. Anaerobic Conditions: ○ Reduced to lactate (in animals) or ethanol (in yeast). 8. Key Regulatory Points 1. Hexokinase: Controls glucose entry into glycolysis. 2. Phosphofructokinase-1 (PFK-1): ○ Activated by: AMP, ADP, fructose-2,6-bisphosphate. ○ Inhibited by: ATP, citrate. 3. Pyruvate Kinase: ○ Activated by: Fructose-1,6-bisphosphate. ○ Inhibited by: ATP, alanine. 9. Notable Enzymes Hexokinase: Phosphorylates glucose (requires Mg²⁺). Phosphofructokinase-1 (PFK-1): First committed step. Pyruvate Kinase: Converts PEP to pyruvate, producing ATP. 10. Additional Notes Glycolysis is highly conserved across species, emphasizing its central role in energy metabolism. The preparatory and payoff phases are energetically coupled to ensure efficiency. This guide covers glycolysis comprehensively. Let me know if you need details on gluconeogenesis or the pentose phosphate pathway!