Environmental Geology, 9th Edition (2010) - Mass Movements
Document Details
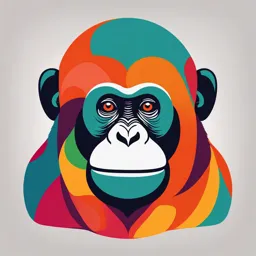
Uploaded by BelievableJubilation8063
Batangas State University - TNEU
2010
Carla W. Montgomery
Tags
Summary
This textbook chapter discusses mass movements, including landslides and other downward movements of geological materials. It examines the factors influencing slope stability, such as slope angle, material properties, and pore pressure. The chapter also touches upon the assessment of landslide risks. This includes considerations of regional topography and soil types.
Full Transcript
mon24085_ch08_171-194.indd Page 171 12/9/09 8:43:26 AM user-s176 /Volumes/204/MHDQ173/mon24085%0/0073524085/mon24085_pagefiles...
mon24085_ch08_171-194.indd Page 171 12/9/09 8:43:26 AM user-s176 /Volumes/204/MHDQ173/mon24085%0/0073524085/mon24085_pagefiles C H A P T E R Mass Movements 8 W hile the internal heat of the earth drives mountain- building processes, just as inevitably, the force of gravity acts to tear the mountains down. Gravity is the great leveler. It imagine from the usually brief coverage of landslides in the news media. In the United States alone, landslides and other mass movements cause over $3.5 billion in property damage every tugs constantly downward on every mass of material every- year, and 25 to 50 deaths. Many landslides occur quite indepen- where on earth, causing a variety of phenomena collectively dently of human activities. In some areas, active steps have been called mass wasting, or mass movements, whereby geological taken to control downslope movement or to limit its damage. materials are moved downward, commonly downslope, from On the other hand, certain human activities aggravate local one place to another. The movement can be slow, subtle, almost landslide dangers, usually as a result of failure to take those haz- undetectable on a day-to-day basis but cumulatively large over ards into account. days or years. Or the movement can be sudden, swift, and devas- Large areas of this country—and not necessarily only tating, as in a rockslide or avalanche. Mass movements need not mountainous regions—are potentially at risk from landslides (fig- even involve slipping on a slope: vertical movements include ure 8.1). It is important to realize, too, that given the area repre- surface subsidence, as from extraction of oil or ground water, or sented in that figure, it is necessarily a generalization, representing collapse into sinkholes as described further in chapter 11. regional landslide hazard levels associated with the characteristic Landslide is a general term for the results of rapid mass regional topography, soil type, etc. One can live in a high-risk spot movements. Overall, landslide damage is greater than one might within an area generally classified as low-risk on this map! This cliff beside a road in southern California looks like a slope failure waiting to happen. Note undercutting of footbridge at center and debris from sliding at lower left. At minimum, erosion by rain will continue. 171 mon24085_ch08_171-194.indd Page 172 12/29/09 3:16:27 PM user-f463 /Volumes/204/MHDQ173/mon24085%0/0073524085/mon24085_pagefiles WA ME MT ND OR VT MN NH ID WI NY MA SD RI MI CT WY IA PA NJ NE NV OH DE UT IL IN DC MD CO WV CA VA KS MO KY NC TN AZ OK AR SC NM MS AL GA TX LA EXPLANATION FL Landslide incidence Low (less than 1.5% of area involved) Moderate (15% –1.5% of area involved) High (greater than 15% of area involved) Landslide susceptibility/incidence Moderate susceptibility/low incidence Figure 8.1 High susceptibilty/low incidence High susceptibility/moderate incidence Landslide potential of regions in the United States. After U.S. Geological Survey Open-File Report 97-289 Factors Influencing Slope Stability Basically, mass movements occur whenever the downward pull of gravity overcomes the forces—usually frictional—resisting it. The downslope pull tending to cause mass movements, called the shearing stress, is related to the mass of material and the slope angle. Counteracting the shearing stress is friction, or, in a coherent solid, shear strength. When shearing stress exceeds frictional resistance or the shear strength of the material, sliding occurs. Therefore, factors that increase shearing stress, decrease friction, or decrease shear strength tend to increase the likeli- hood of sliding, and vice versa. Application of these simple principles is key to reducing landslide hazards. Figure 8.2 Effects of Slope and Materials Effects of slope geometry on slide potential. The mass of the block and thus the total downward pull of gravity are the same in both All else being equal, the steeper the slope, the greater the shear- cases, but the steeper the slope, the greater the shearing stress ing stress (figure 8.2) and therefore the greater the likelihood of component. An increase in pore pressure can decrease frictional slope failure. For dry, unconsolidated material, the angle of resistance to shearing stress. 172 Section Three Surface Processes mon24085_ch08_171-194.indd Page 173 11/24/09 9:18:08 PM user-s176 /Volumes/204/MHDQ173/mon24085/0073524085/mon24085_pagefiles A B Figure 8.3 (A) Angle of repose indicates an unconsolidated material’s resistance to sliding. Coarser and rougher particles can maintain a steeper stable slope angle. (Variation in angle of repose in natural materials is less than the range illustrated here.) (B) This California cliff holds its near-vertical slope only temporarily; eventually the weakly cohesive sediment collapses to assume its natural angle of repose, illustrated by the slope of the surface of the sediment pile at the base of the cliff. (B) Photograph by J. T. McGill, USGS Photo Library, Denver, CO. repose is the maximum slope angle at which the material is Over long periods of time, slow tectonic deformation also stable (figure 8.3). This angle varies with the material. Smooth, can alter the angles of slopes and bedding planes, making them rounded particles tend to support only very low-angle slopes steeper or shallower. This is most often a significant factor in (imagine trying to make a heap of marbles or ball bearings), young, active mountain ranges, such as the Alps or the coast while rough, sticky, or irregular particles can be piled more ranges of California. Steepening of slopes by tectonic move- steeply without becoming unstable. Other properties being ments has been suggested as the cause of a large rockslide in equal, coarse fragments can usually maintain a steeper slope Switzerland in 1806 that buried the township of Goldan under a angle than fine ones. The tendency of a given material to as- block of rock nearly 2 kilometers long, 300 meters across, and sume a constant characteristic slope can be seen in such diverse 60 to 100 meters thick. In such a case, past evidence of land- geologic forms as cinder cones (figure 8.4A) and sand dunes slides may be limited in the immediate area because the danger- (figure 8.4B). ously steep slopes have not existed long in a geologic sense. Solid rock can be perfectly stable even at a vertical slope but may lose its strength if it is broken up by weathering or fracturing. Also, in layered sedimentary rocks, there may be Effects of Fluid weakness along bedding planes, where different rock units are The role of fluid in mass movements is variable. Addition of imperfectly held together; some units may themselves be weak some moisture to dry soils may increase adhesion, helping the or even slippery (clay-rich layers, for example). Such planes of particles to stick together (it takes damp sand to make a sand weakness are potential slide or failure planes. castle). However, saturation of unconsolidated materials re- Slopes may be steepened to unstable angles by natural duces the friction between particles that otherwise provides erosion by water or ice. Erosion also can undercut rock or soil, cohesion and strength, and the reduced friction can destabilize removing the support beneath a mass of material and thus leav- a slope. As was described in chapter 4, elevated pore-fluid ing it susceptible to falling or sliding. This is a common contrib- pressure can trigger earthquakes by facilitating slip in faulted uting factor to landslides in coastal areas and along stream rocks under tectonic stress. It is also effective in promoting valleys; recall figures 7.4A and 7.21C. sliding in rocks under stress due to gravity, reducing the force Chapter Eight Mass Movements 173 mon24085_ch08_171-194.indd Page 174 11/24/09 9:18:14 PM user-s176 /Volumes/204/MHDQ173/mon24085/0073524085/mon24085_pagefiles A Figure 8.5 Instability in expansive clay seriously damaged this road near Boulder, Colorado. Photograph courtesy USGS Photo Library, Denver, CO. B Figure 8.4 (A) Angle of repose of volcanic cinders is shown not only by the Figure 8.6 smoothly sloping sides of each individual cinder cone in the summit crater of Haleakala volcano in Hawaii, but also by the Laguna Beach, CA landslide of 1 June 2005. consistent slope and shape from cone to cone. (B) The uniform Photograph by Gerald Bawdeu, U.S. Geological Survey slope on the face of this sand dune is maintained by slumping to the sand’s angle of repose. Oregon Dunes National Recreation Area. expands, loosens and displaces the soil, which may then be more susceptible to sliding when it next thaws. holding the rock to the plane below (vector perpendicular to Some soils rich in clays absorb water readily; one type of slope in figure 8.2). The very mass of water in saturated soil clay, montmorillonite, may take up twenty times its weight in adds extra weight, and thus extra downward pull. water and form a very weak gel. Such material fails easily un- Aside from its role in erosion, water can greatly increase der stress. Other clays expand when wet, contract when dry, the likelihood of mass movements in other ways. It can seep and can destabilize a slope in the process (figure 8.5; see also along bedding planes in layered rock, reducing friction and chapter 20). making sliding more likely. The expansion and contraction of Sudden, rapid landslides commonly involve a triggering water freezing and thawing in cracks in rocks or in soil can act mechanism. Heavy rainfall or rapid melting of abundant snow as a wedge to drive chunks of material apart (frost wedging). can be a very effective trigger, quickly adding weight, decreas- Frost heaving, the expansion of wet soil as it freezes and the ice ing friction, and increasing pore pressure (figure 8.6). As with 174 Section Three Surface Processes mon24085_ch08_171-194.indd Page 175 12/14/09 11:10:06 AM user-s176 /Volumes/204/MHDQ173/mon24085%0/0073524085/mon24085_pagefiles A Figure 8.7 (A) Debris flows in Deming, Washington, following two days of heavy storms. Note buildings mired in the mud near the road. More than 1500 new landslides resulted from these storms. (B) Closeup of debris flow in Whatcom County, Washington. Photographs courtesy Washington Division of Geology & Earth Resources, U.S. Geological Survey B flooding, the key is not simply how much water is involved, but shear strength. Moisture loss through the vegetation by transpi- how rapidly it is added and how close to saturated the system ration also helps to dry out sodden soil more quickly. Com- was already. The January 2009 landslides in the Pacific monly, then, vegetation tends to increase slope stability. Northwest (figure 8.7) accompanied severe flooding, both prob- However, the plants also add weight to the slope. If the added lems caused by heavy rainfall onto—and contributing to rapid weight is large and the root network of limited extent, the veg- melting of—unusually heavy snow that had fallen earlier in the etation may have a destabilizing effect instead. Also, plants that winter. Tropical regions subject to intense cloudbursts may also take up so much water that they dry out soil until it loses its be subject to frequent slides in thick tropical soils—which may adhesion can aggravate the slide hazard. tend to be clay-rich—and in severely weathered rock. A relatively recently recognized hazard is the fact that when previously forested slopes are bared by fires or by log- ging, they may become much more prone to sliding than before. Effects of Vegetation Previous illegal logging on slopes above the town of Guin- Plant roots, especially those of trees and shrubs, can provide a saugon in the Philippines was blamed for the February 2006 strong interlocking network to hold unconsolidated materials mudslide, triggered by heavy rains, that engulfed the town and together and prevent flow (figure 8.8A). In addition, vegetation killed an estimated 1800 of its 1857 residents. Wildfires in takes up moisture from the upper layers of soil and can thus California frequently strip slopes of stabilizing vegetation, and reduce the overall moisture content of the mass, increasing its deadly landslides may follow (figure 8.8B). Chapter Eight Mass Movements 175 mon24085_ch08_171-194.indd Page 176 12/10/09 10:22:41 AM user /Volumes/204/MHDQ173/mon24085%0/0073524085/mon24085_pagefiles Nevados Huascarán Avalanche Lgnas. Llanganuco source A Yungay Ranrachira ta S an Rio B Figure 8.8 Figure 8.9 (A) Examination of this Alaskan roadcut shows surface soil held in The Nevados Huascarán debris avalanche, Peru, 1970. place by grass roots, erosion and slope failure below. (B) October Photograph courtesy USGS Photo Library, Denver, CO. 2003 wildfires removed vegetation in Cable Canyon, California; rains sent this debris flow through a campground on 25 December, killing two people. shook loose a much larger debris avalanche that buried most of (B) Photograph by Sue Cannon, U.S. Geological Survey the towns of Yungay and Ranrachira and more than 18,000 peo- ple (figure 8.9). Once sliding had begun, escape was impossible: Earthquakes Some of the debris was estimated to have moved at 1000 kilo- meters per hour (about 600 miles per hour). The steep mountains Landslides are a common consequence of earthquakes in hilly of Central and South America, sitting above subduction zones, terrain, as noted in chapter 4. Seismic waves passing through continue to suffer earthquake-induced slides (figure 8.10). rock stress and fracture it. The added stress may be as much as Earthquakes in and near ocean basins may also trigger sub- half that already present due to gravity. Ground shaking also marine landslides. Sediment from these may be churned up into jars apart soil particles and rock masses, reducing the friction a denser-than-water suspension that flows downslope to the sea that holds them in place. In the 1964 Alaskan earthquake, the floor, much as pyroclastic flows flow down from a volcano. These Turnagain Heights section of Anchorage was heavily damaged turbidity currents not only carry sediment to the deep-sea floor; by landslides (recall figure 4.27C). California contains not only they are forceful enough to break cables, and they could therefore many fault zones but also many sea cliffs and hillsides prone to disrupt transatlantic communications in the days when all trans- landslides during earthquakes. atlantic telephone calls were carried by undersea cables! One of the most lethal earthquake-induced landslides oc- curred in Peru in 1970. An earlier landslide had already occurred below the steep, snowy slopes of Nevados Huascarán, the high- Quick Clays est peak in the Peruvian Andes, in 1962, without the help of an A geologic factor that contributed to the 1964 Anchorage land- earthquake. It had killed approximately 3500 people. In 1970, a slides and that continues to add to the landslide hazards in magnitude-7.7 earthquake centered 130 kilometers to the west Alaska, California, parts of northern Europe, and elsewhere is a 176 Section Three Surface Processes mon24085_ch08_171-194.indd Page 177 11/24/09 9:18:48 PM user-s176 /Volumes/204/MHDQ173/mon24085/0073524085/mon24085_pagefiles pore water. The sodium chloride in the pore water acts as a glue, holding the clay particles together. Fresh water subsequently infiltrating the clay washes out the salts, leaving a delicate, hon- eycomblike structure of particles. Seismic-wave vibrations break the structure apart, reducing the strength of the quick clay by as much as twenty to thirty times, creating a finer-grained equivalent of quicksand that is highly prone to sliding. Failure of the Bootlegger Clay, a quick clay underlying Anchorage, Alaska, was responsible for the extent of damage from the 1964 earthquake. Nor is a large earthquake necessary to trigger fail- ure; vibrations from passing vehicles can also do it. So-called sensitive clays are somewhat similar in behavior to quick clays but may be formed from different materials. Weathering of vol- canic ash, for example, can produce a sensitive-clay sediment. Such deposits are not uncommon in the western United States. Figure 8.10 Slide on Pan-American Highway east of Ropango, El Salvador, Types of Mass Wasting triggered by magnitude-7.6 earthquake in January 2001. Photograph by E. L. Harp, U.S. Geological Survey In the broadest sense, even subsidence of the ground surface is a form of mass wasting because it is gravity-driven. Subsidence includes slow downward movement as ground water or oil is extracted, and the rapid drop of sinkhole formation, described in chapter 11. Here we focus on downslope movements and displacement of distinct masses of rock and soil. When downslope movement is quite slow, even particle by particle, the motion is described as creep. Soil creep, which is often triggered by frost heaving, occurs more commonly than rock creep. Though gradual, creep may nevertheless leave tell- tale signs of its occurrence so that areas of particular risk can be avoided. For example, building foundations may gradually weaken and fail as soil shifts; roads and railway lines may be disrupted. Often, soil creep causes serious property damage, though lives are rarely threatened. A related phenomenon, solifluction, describes slow movement of wet soil over imper- meable material. It will be considered further in connection with permafrost, discussed in chapter 10. Mass movements may be subdivided on the basis of the type of material moved and the characteristic type or form of movement. The material moved can range from unconsolidated, fairly fine material (for example, soil or snow) to large, solid masses of rock. Landslide is a nonspecific term for rapid mass movements in rock or soil. There are several types of landslides, and a particular event may involve more than one type of motion. The rate of motion is commonly related to the proportion of Figure 8.11 moisture: frequently, the wetter the material, the faster the move- Example of quick clay. Turnagain Heights, Anchorage, Alaska. ment. In general, the more rapid the movement, the greater the likelihood of casualties. A few examples may clarify the different types of mass movements, which are summarized in figure 8.12. material known as “quick” or “sensitive” clay. True quick clays (figure 8.11) are most common in northern polar latitudes. The grinding and pulverizing action of massive glaciers can produce Falls a rock flour of clay-sized particles, less than 0.02 millimeter A fall is a free-falling action in which the moving material is (0.0008 inch) in diameter. When this extremely fine material is not always in contact with the ground below. Falls are most deposited in a marine environment, and the sediment is later often rockfalls (figure 8.13). They frequently occur on very uplifted above sea level by tectonic movements, it contains salty steep slopes when rocks high on the slope, weakened and Chapter Eight Mass Movements 177 mon24085_ch08_171-194.indd Page 178 11/24/09 9:18:57 PM user-s176 /Volumes/204/MHDQ173/mon24085/0073524085/mon24085_pagefiles Types of Mass Movement and Typical Rates of Movement Material moves Chaotic, as coherent incoherent unit movement Free-falling Rockfall Soil fall (rare) motion Speed: Rapid Speed: Rapid (meters/second) (meters/second) In contact with Slides: Flows: surface below rockslide snow avalanche slump (soil) debris avalanche nuée ardente debris flow earthflow mudflow lahar Speed: Highly variable Speed: Rapid (meters/year to (meters/day to meters/second) meters/second) Figure 8.12 Comparison of nature of various types of mass movements. broken up by weathering, lose support as materials under them Slumps and Slides erode away. Falls are common along rocky coastlines where In a slide, a fairly cohesive unit of rock or soil slips downward cliffs are under cut by wave action and also at roadcuts through along a clearly defined surface or plane. Rockslides most often solid rock. The coarse rubble that accumulates at the foot of a involve movement along a planar fracture or a bedding plane slope prone to rockfalls is talus (figure 8.14). As the pounding between successive layers of sedimentary rocks, or slippage water undercuts the resistant rock layer that has created Ni- where differences in original composition of the sedimentary agara Falls (figure 8.15), repeated rockfalls cause the falls to layers results in a weakened layer or a surface with little cohe- move slowly upstream, while talus accumulates at the base of sion (figure 8.16). Rockslides may be complex, with multiple the falls. rock blocks slipping along the same plane. 178 Section Three Surface Processes mon24085_ch08_171-194.indd Page 179 11/24/09 9:19:01 PM user-s176 /Volumes/204/MHDQ173/mon24085/0073524085/mon24085_pagefiles Consequences of Mass Movements Just as landslides can be a result of earthquakes, other unfortunate events, notably floods, can be produced by landslides. A stream in the process of cutting a valley may create unstable slopes. Subse- quent landslides into the valley can dam up the stream flowing through it, creating a natural reservoir. The filling of the reservoir makes the area behind the earth dam uninhabitable, though it usu- ally happens slowly enough that lives are not lost. Heavy rains and snows in Utah in 1982–1983 caused landslides in unprecedented numbers. In one case, an old 3-million-cubic-meter slide near Thistle, Utah, was reactivated. It blocked Spanish Fork Canyon, creating a large lake and cut- ting off one transcontinental railway line and a major highway route into central Utah (figure 8.20). Shipments of coal and other supplies were disrupted. Debris flows north of Salt Lake City also forced hundreds of residents to evacuate and de- stroyed some homes, and water levels in reservoirs in the Wasatch Plateau were lowered to reduce dangers of landslides into the reservoirs or breaching of the dams. A further danger is that the unplanned dam formed by a landslide will later fail. In 1925, an enormous rockslide a kilo- meter wide and over 3 kilometers (2 miles) long in the valley of the Gros Ventre River in Wyoming blocked that valley, and the resulting lake stretched to 9 kilometers long. After spring rains Figure 8.13 and snowmelt, the natural dam failed. Floodwaters swept down Rockfall in Colorado National Monument. Note accumulation of the valley below. Six people died, but the toll might have been talus at base of cliff, from past falls. far worse in a more populous area. Slumps can occur in rock or soil. In a soil slump, a rotational Impact of Human Activities movement of the soil mass typically accompanies the downslope movement. A scarp may be formed at the top of the slide. Usually, Given the factors that influence slope stability, it is possible to the surface at the top of the slide is relatively undisturbed. The see many ways in which human activities increase the risk of lower part of the slump block may end in a flow (figure 8.17). A mass movements. One way is to clear away stabilizing vegeta- rockslide that does not move very far may also be a slump. tion. As noted earlier, where clear-cutting logging operations have exposed sloping soil, landslides of soil and mud may occur far more frequently or may be more severe than before. Flows and Avalanches Many types of construction lead to oversteepening of In a flow, the material moved is not coherent but moves in a slopes. Highway roadcuts, quarrying or open-pit mining opera- more chaotic, disorganized fashion, with mixing of particles tions, and construction of stepped home-building sites on hill- within the flowing mass, as a fluid flows. Flows of unconsoli- sides are among the activities that can cause problems dated material are extremely common. They need not involve (figure 8.21). Where dipping layers of rock are present, re- only soils. Snow avalanches are one kind of flow; nuées ar- moval of material at the bottom ends of the layers may leave dentes, or pyroclastic flows (see chapter 5), are another, associ- large masses of rock unsupported, held in place only by friction ated only with volcanic activity. Where soil is the flowing between layers. Slopes cut in unconsolidated materials at angles material, these phenomena may be described as earthflows higher than the angle of repose of those materials are by nature (fairly dry soil; figure 8.18) or mudflows (if saturated with wa- unstable, especially if there is no attempt to plant stabilizing ter), which include the volcanic lahars. A flow involving a wide vegetation (figure 8.22). In addition, putting a house above a variety of materials—soil, rocks, trees, and so on—together in naturally unstable or artificially steepened slope adds weight to a single flow is a debris avalanche, or debris flow, the latter the slope, thereby increasing the shear stress acting on the slope. term more often used for water-saturated debris (figure 8.19; Other activities connected with the presence of housing devel- recall also figures 8.7 and 8.8B). Regardless of the nature of the opments on hillsides can increase the risk of landslides in more materials moved, all flows have in common the chaotic, inco- subtle ways. Watering the lawn, using a septic tank for sewage herent movement of the particles or objects in the flow. disposal, and even putting in an in-ground swimming pool from Chapter Eight Mass Movements 179 mon24085_ch08_171-194.indd Page 180 11/24/09 9:19:05 PM user-s176 /Volumes/204/MHDQ173/mon24085/0073524085/mon24085_pagefiles A B Figure 8.14 Rockfalls occur in many settings, creating talus from many different rock types: (A) the sandstones of Pictured Rocks National Lakeshore; (B) gneiss boulders that tumble down a slope crossed by a trail in the Colorado Rockies, (C) chunks of volcanic rock fallen from the columns of Devil’s Tower. Note in (B) and (C) that talus, too, tends to assume a consistent slope angle. which water can seep slowly out are all activities that increase the moisture content of the soil and render the slope more sus- ceptible to slides. On the other hand, the planting of suitable vegetation can reduce the risk of slides. Recent building-code restrictions have limited develop- ment in a few unstable hilly areas, and some measures have been taken to correct past practices that contributed to land- slides. (Chapter 19 includes an illustration of the effectiveness of legislation to restrict building practices in Los Angeles County.) However, landslides do not necessarily cease just be- cause ill-advised practices that caused or accelerated them have been stopped. Once activated or reactivated, slides may continue to slip for decades. The area of Portuguese Bend in the Palos Verdes Peninsula in Los Angeles County is a case in point. In the 1950s, housing developments were begun in Portu- guese Bend. In 1956, a 1-kilometer-square area began to slip, though the slope in the vicinity was less than 7 degrees, and within months, there had been 20 meters of movement. What activated this particular slide is unclear. Most of the homes used cesspools for sewage disposal, which added fluid to the ground, potentially increasing fluid pressure. On the other hand, the county highway department, in building a road across what be- C came the top of this slide, added a lot of fill, and thus weight. In any event, the resultant damage was extensive: cracks developed within the sliding mass; houses on and near the slide were 180 Section Three Surface Processes mon24085_ch08_171-194.indd Page 181 12/10/09 10:22:53 AM user /Volumes/204/MHDQ173/mon24085%0/0073524085/mon24085_pagefiles Figure 8.15 Niagara Falls are moving slowly upstream as a consequence of rockfalls. This is also a site at which the International Joint Commission regulates Great Lakes outflow. Photograph by Ken Winters, courtesy U.S. Army Corps of Engineers A B Figure 8.16 Figure 8.17 Rockslides occur along steeply sloping fractures in this granite in (A) Slump and flow at La Conchita, California, in spring 1995. Houses the Colorado Rocky Mountains. at the toe were destroyed by the debris flow, but no one was killed. (B) Ten years later, part of the 1995 slide remobilized after a period of heavy rain, flowing out over four blocks of the town, and ten people damaged or destroyed; a road built across the base of the slide died. What is perhaps more disturbing is that recent studies suggest had to be rebuilt repeatedly. Over $10 million in property damage that the La Conchita slides are just a small part of a much larger resulted from the slippage. Worse, the movement has continued complex slide; see scarp at upper left. for decades since, slow but unstoppable, at a rate of about 3 me- (A) Photograph by R. L. Schuster, (B) photograph by Jonathan Godt, ters per year. Some portions of the slide have moved 70 meters. both courtesy U.S. Geological Survey Chapter Eight Mass Movements 181 mon24085_ch08_171-194.indd Page 182 11/24/09 9:19:40 PM user-s176 /Volumes/204/MHDQ173/mon24085/0073524085/mon24085_pagefiles Figure 8.18 Recent earthflows in grassland, Gilroy, California. Photograph © The McGraw-Hill Companies, Inc./Doug Sherman, photographer Figure 8.20 An old, 3-million-cubic-meter slide near Thistle, Utah, was reactivated in the wet spring of 1983. It blocked Spanish Fork Canyon and cut off highway and rail routes (note where they continue up the valley near top of photo). Residents were driven from their homes as the lake behind the slide grew. Photograph courtesy USGS Photo Library, Denver, CO. the next slide: Often, the cleanup involves removing the toe of a slump or flow—for example, where it crosses a road. This removes support for the land above and may oversteepen the local slope, too. Artificial reservoirs may cause not only earth- quakes, but landslides, too. As the reservoirs fill, pore pressures in rocks along the sides of the reservoir increase, and the strength of the rocks to resist shearing stress can be correspond- ingly decreased. The case of the Vaiont reservoir disaster (Case Figure 8.19 Study 8) is a classic in this respect. Chapter 20 describes other Puget Peak debris avalanche following 1964 earthquake, Alaska. examples of ways in which engineering activities may influence Photograph by G. Plafker, USGS Photo Library, Denver, CO. land stability. Human activities can increase the hazard of landslides in A Compounding of Problems: still other ways. Irrigation and the use of septic tanks increase the Venezuelan Coast the flushing of water through soils and sediments. In areas un- On the north coast of Venezuela, the terrain is mountainous, derlain by quick or sensitive clays, these practices may hasten with steep slopes and narrow stream channels. A few people the washing-out of salty pore waters and the destabilization of settled on the slopes, risking landslides; larger towns developed the clays, which may fail after the soils are drained. Even in the only flat areas available, beside the streams or in alluvial cleanup after one slide may reduce stability and contribute to plains at the mouths of the valleys (figure 8.23). 182 Section Three Surface Processes mon24085_ch08_171-194.indd Page 183 12/21/09 7:10:26 PM user-s-179 /Volumes/204/MHDQ173/mon24085%0/0073524085/mon24085_pagefiles Fill added in roadbed; increases load on hill. Home adds weight on Original slope slope. oversteepened by cutting homesite; support for hillside above reduced. Infiltration from septic system soaks soil. Leakage from pool also adds water to soil. Figure 8.21 Effects of construction and human habitation on slope stability. Figure 8.23 Quebrada San Julián (foreground) and Caraballeda (rear) were built, Figure 8.22 respectively, in a floodplain and on an alluvial plain; but the Failure of steep slope along a roadcut now threatens the cabin mountainous terrain leaves little choice. above. Photograph by M. C. Larsen and H. T. Sierra, U.S. Geological Survey Chapter Eight Mass Movements 183 mon24085_ch08_171-194.indd Page 184 11/24/09 9:20:02 PM user-s176 /Volumes/204/MHDQ173/mon24085/0073524085/mon24085_pagefiles Case Study 8 The Vaiont Dam The Vaiont River flows through an old glacial valley in the Italian Alps. The tended to buoy up the rocks and to swell the clays of the clay-rich layers, valley is underlain by a thick sequence of sedimentary rocks, principally further decreasing their strength, making sliding easier. limestones with some clay-rich layers, that were folded and fractured during In 1960, a block of 700,000 cubic meters of rock slid from the the building of the Alps. The sedimentary units of the Vaiont Valley are in slopes of Monte Toc on the south wall into the reservoir. Creep was the form of a syncline, or trough-shaped fold, so the beds on either side of noted over a still greater area. A set of monitoring stations was the valley dip down toward the valley (figure 1). The rocks themselves are established on the slopes of Monte Toc to track any further movement. relatively weak and particularly prone to sliding along the clay-rich layers. Measurable creep continued. In 1960–1961, the rate of creep Evidence of old rockslides can be seen in the valley. Extensive solution of occasionally reached 25 to 30 centimeters (10 to 12 inches) per week, the carbonates by ground water further weakens the rocks. and the total volume of rock affected by the creep was estimated at The Vaiont Dam, built for power generation, is the highest about 200 million cubic meters. “thin-arch” dam in the world. It is made of concrete and stands over 265 Late summer and early fall of 1963 were times of heavy rainfall in meters (875 feet) high at its highest point. Modern engineering methods the Vaiont Valley. The rocks and soils were saturated. The reservoir level were used to stabilize the rocks in which it is based. The capacity of the rose by over 20 meters, and pore pressures in the rocks increased. By reservoir behind the dam was 150 million cubic meters—initially. mid-September 1963, measured creep rates were consistently about 1 The obvious history of landslides in the area originally led some to centimeter per day. What was still not realized was that the rocks were object to the dam site. Later, the very building of the dam and subsequent slipping not in a lot of small blocks, but as a single, coherent mass. filling of the reservoir aggravated an already-precarious situation. As the Animals sensed the danger before people did. On October 1, water level in the reservoir rose following completion of the dam, pore animals that had been grazing on those slopes of Monte Toc moved off pressures of ground water in the rocks of the reservoir walls rose also. This the hillside and reportedly refused to return. The rains continued, and so did the creep. Creep rates grew to 20 to 30 centimeters per day. Finally, on October 8, the engineers realized La nd that all the creep-monitoring stations were moving together. Worse yet, sur fac Slide block they also discovered that a far larger area of hillside was moving than they e Top of slide– had thought. They then tried to reduce the water level in the reservoir by after ne opening the gates of two outlet tunnels. But it was too late, and the water pla ide level continued to rise: The silently creeping mass had begun to encroach Folded limestones Sl s and shales ne on the reservoir, decreasing its capacity significantly. On the night of la Old in gp October 9, in the midst of another downpour, the disaster struck. 0 500 1000 fau dd Be A resident of the town of Casso, on the slopes above the lt meters reservoir, later reported that, at first, there was just a sound of rolling rocks, ever louder. Then a gust of wind hit his house, smashing windows Figure Figu Figure re 1 and raising the roof so that the rain poured in. The wind suddenly died; Geologic cross section of the Vaiont valley. the roof collapsed. G. A. Kiersch, “The Vaiont Reservoir Disaster,” Mineral Information Service A chunk of hillside, over 240 million cubic meters in volume, had 18(7):129–138, 1965. State of California, Division of Mines and Geology. slid into the reservoir (figure 2). The shock of the slide was detected by The first two weeks of December 1999 were hardly dry, runoff. And there are few safe sites in the region for those who with 293 millimeters (nearly a foot) of rain, but the real prob- choose to stay and rebuild. lem was the deluge of another 911 millimeters (close to 3 feet) of rain that fell December 14–16. Runoff from the steep slopes was swift, and flash floods quickly rose in the valleys. Worse Possible Preventive Measures yet, thousands of debris avalanches from the hillsides cascaded into the same valleys, mingling with floodwaters to make huge Avoiding the most landslide-prone areas altogether would debris flows that swept out into the alluvial plains and through greatly limit damages, of course, but as is true with the towns (figure 8.24). An estimated 30,000 people died from fault zones, floodplains, and other hazardous settings, the consequences of flooding and landslides combined. developments may already exist in areas at risk, and eco- Nor is the danger now past, for much rock and soil re- nomic pressure for more development can be strong. In mains in those stream channels, to be remobilized by future areas such as the Venezuelan coast, there may be little 184 Section Three Surface Processes mon24085_ch08_171-194.indd Page 185 12/14/09 11:10:31 AM user-s176 /Volumes/204/MHDQ173/mon24085%0/0073524085/mon24085_pagefiles seismometers in Rome, in Brussels, and elsewhere around Europe. That discredit of those who chose the site that the dam and reservoir were ever immense movement, occurring in less than a minute, set off the shock put in such a spot. Ample evidence of slope instability dated back long wave of wind that rattled Casso and drew the water 240 meters upslope before the conception of the dam. With more thorough study of the rocks’ out of the reservoir after it. Displaced water crashed over the dam in a properties and structure and the possible effects of changing water levels, wall 100 meters (over 325 feet) above the dam crest and rushed down the Vaiont tragedy could have been avoided. the valley below. The water wave was still over 70 meters high some History often seems destined to repeat itself. In the late 1970s, the 1.5 kilometers downstream at the mouth of the Vaiont Valley, where it Tablacha Dam on Rio Mantaro, Peru, was threatened by a similar failure flowed into the Piave River. The energy of the rushing water was such (figure 3). Foliated metamorphic rocks dip toward the dam and reservoir. A that some of it flowed upstream in the Piave for more than 2 kilometers. portion of an ancient slide was reactivated, most likely by the increasing Within about five minutes, nearly 3000 people were drowned and whole pore pressure due to reservoir filling. The situation is much like that of the towns wiped out. Vaiont Dam. In this case, however, the Peruvian government spent $40 It is a great tribute to the designer and builder of the Vaiont Dam million on stabilization efforts—building a 460,000-cubic-meter earth that the dam itself held through all of this, resisting forces far beyond buttress at the toe of the slide block, and adding drainage, rock bolts, and the design specifications; it still stands today. It is also very much to the other measures. So far, they seem to be succeeding. Figure Figu Figure re 2 Figure Fig Figur ure e3 The landslide at the Vaiont reservoir and resultant flood damage. Reactivated slide block (identified by red arrows) above Tablacha Dam G. A. Kiersch, “The Vaiont Reservoir Disaster,” Mineral Information Service and reservoir, Peru. 18(7): 129–138, 1965. State of California, Division of Mines and Geology. Photograph by J. T. McGill, USGS Photo Library, Denver, CO. buildable land not at risk. Population density, too, coupled pensive on a large scale, however, and no use at all if the base with lack of “safe” locations, may push development into on which the structure was built were sliding also. unsafe areas. There are some other steps that can be taken to reduce Sometimes, local officials just resign themselves to the actual landslide hazards. existence of a mass-movement problem and perhaps take some steps to limit the resulting damage (figure 8.25). In places where the structures to be protected are few or small, and the Slope Stabilization slide zone is narrow, it may be economically feasible to bridge If a slope is too steep to be stable under the load it carries, any of structures and simply let the slides flow over them. For exam- the following steps will reduce slide potential: (1) reduce the slope ple, this might be done to protect a railway line or road running angle, (2) place additional supporting material at the foot of the along a valley from avalanches—either snow or debris—from slope to prevent a slide or flow at the base of the slope, or (3) reduce a particularly steep slope. This solution would be far too ex- the load (weight, shearing stress) on the slope by removing some Chapter Eight Mass Movements 185 mon24085_ch08_171-194.indd Page 186 11/24/09 9:20:11 PM user-s176 /Volumes/204/MHDQ173/mon24085/0073524085/mon24085_pagefiles A B Figure 8.24 Damage in Caraballeda: (A) Overview of Los Corales sector. (B) A close look at this apartment building shows that at their peak, debris flows flowed through the lowest three floors. (C) Debris-flow deposits in channel at San José de Galipán. Sizes of largest boulders C moved—estimated to weigh up to 400 tons—confirm the force of the flow. of the rock or soil (or artificial structures) high on the slope (fig- Photographs by M. C. Larsen and H. T. Sierra, U.S. Geological Survey ure 8.26). These measures may be used in combination. Depend- ing on just how unstable the slope, they may need to be executed cautiously. If earthmoving equipment is being used to remove soil with an impermeable material and diverting surface runoff at the top of a slope, for example, the added weight of the equip- above the slope. Alternatively, subsurface drainage might be ment and vibrations from it could possibly trigger a landslide. undertaken. Systems of underground boreholes can be drilled to To stabilize exposed near-surface soil, ground covers or increase drainage, and pipelines installed to carry the water out other vegetation may be planted (preferably fast-growing mate- of the slide area (figure 8.29). All such moisture-reducing tech- rials with sturdy root systems). But sometimes plants are insuf- niques naturally have the greatest impact where rocks or soils ficient, and the other preventive measures already described are relatively permeable. Where the rock or soil is fine-grained impractical, in a particular situation. Then, retaining walls or and drains only slowly, hot air may be blown through boreholes other stabilization structures can be built against the slope to try to help dry out the ground. Such moisture reduction reduces to hold it in place (figure 8.27). Given the distribution of stresses pore pressure and increases frictional resistance to sliding. acting on retaining walls, the greatest successes of this kind Other slope-stabilization techniques that have been tried have generally been low, thick walls placed at the toe of a fairly include the driving of vertical piles into the foot of a shallow slide coherent slide to stop its movement. High, thin walls have been to hold the sliding block in place. The procedure works only less successful (figure 8.28). where the slide is comparatively solid (loose soils may simply Since water can play such a major role in mass move- flow between piles), with thin slides (so that piles can be driven ments, the other principal strategy for reducing landslide haz- deep into stable material below the sliding mass), and on low- ards is to decrease the water content or pore pressure of the rock angle slopes (otherwise, the shearing stresses may simply snap or soil. This might be done by covering the surface completely the piles). So far, this technique has not been very effective. 186 Section Three Surface Processes mon24085_ch08_171-194.indd Page 187 11/24/09 9:20:21 PM user-s176 /Volumes/204/MHDQ173/mon24085/0073524085/mon24085_pagefiles A B Figure 8.25 Measures to reduce the consequences of slope instability. (A) Chain-link fencing draped over roadcut in Hawaii to protect road from rockfall. (B) Shelter built over railroad track or road in snow avalanche area diverts snow flow. (C) Example of avalanche- protection structure in the Canadian Rockies, in Canada’s Glacier National Park. Recognizing the Hazards Mass movements occur as a consequence of the basic character- C istics of a region’s climate, topography, and geology, indepen- dent of human activities. Where mass movements are naturally common, they tend to recur repeatedly in the same places. Rec- The use of rock bolts to stabilize rocky slopes and, occa- ognition of past mass movements in a region indicates a need for sionally, rockslides has had greater success (figure 8.30). Rock caution and serious consideration of the hazard in any future bolts have long been used in tunneling and mining to stabilize development. Such recognition can also save lives. rock walls. It is sometimes also possible to anchor a rockslide Even when recent activity is limited, the potential can often with giant steel bolts driven into stable rocks below the slip be seen. The granite domes of Yosemite have been shaped by the plane. Again, this works best on thin slide blocks of very coher- flaking-off of massive curving slabs of rock (figure 8.31A), but ent rocks on low-angle slopes. these rockfalls are infrequent. A 1996 fall that killed one park Procedures occasionally used on unconsolidated materials visitor and injured 14 (figure 8.31B) has focused the attention include hardening unstable soil by drying and baking it with heat of the National Park Service on the rockfall hazards and how to (this procedure works well with clay-rich soils) or by treating identify the highest-hazard regions. with portland cement. By far the most common strategies, how- Recognizing past rockfalls can be quite simple in vegetated ever, are modification of slope geometry and load, dewatering, areas. The large chunks of rock in talus are inhospitable to most or a combination of these techniques. The more ambitious engi- vegetation, so rockfalls tend to remain barren of trees and plants. neering efforts are correspondingly expensive and usually re- The few trees that do take hold may only contribute to further served for large construction projects, not individual homesites. breakup by root action. Lack of vegetation may also mark the paths Determination of the absolute limits of slope stability is of past debris avalanches or other soil flows or slides (figure 8.32). an imprecise science. This was illustrated in Japan in 1969, These scars on the landscape point plainly to slope instability. when a test hillside being deliberately saturated with water un- Landslides are not the only kinds of mass movements that expectedly slid prematurely, killing several of the researchers. recur in the same places. Snow avalanches disappear when the The same point is made less dramatically every time a slope snow melts, of course, but historical records of past avalanches stabilization effort fails. can pinpoint particularly risky areas; or, their tracks may be Chapter Eight Mass Movements 187 mon24085_ch08_171-194.indd Page 188 11/24/09 9:20:30 PM user-s176 /Volumes/204/MHDQ173/mon24085/0073524085/mon24085_pagefiles Shear stress A B A C Figure 8.26 Slope stabilization by slope reduction and removal of unstable material along roadcut. (A) Before: Roadcut leaves steep, unsupported slope. If the shale layers (grey) are relatively impermeable, fluid may accumulate along bedding planes, B promoting sliding. (B) After: Material removed to reduce slope angle and load. (C) This roadcut near Denver, CO has been “terraced,” cut Figure 8.27 into steps to break up the long slope while reducing the overall (A) Retaining wall of concrete blocks on slope cut in old slide debris, slope angle. Roadbed for one direction of traffic appears at lower Pacific Palisades area, California. (B) Side view of retaining structure right; retaining wall for the other is two steps up. If the farther lanes formed by rock rubble encased in wire mesh, Lake Tahoe, Nevada. had been brought down to the level of the nearer ones, the slope Note that this is also stepped rather like the roadcut in figure 8.26. would have had to be even steeper, and harder to stabilize. (A) Photograph by J. T. McGill, USGS Photo Library, Denver, CO. mon24085_ch08_171-194.indd Page 189 11/24/09 9:20:47 PM user-s176 /Volumes/204/MHDQ173/mon24085/0073524085/mon24085_pagefiles A A B Figure 8.29 Improved drainage enhances slope stability by reducing pore pressure. (A) Before: Water trapped in wet soil causes movement, pushing down retaining wall. (B) After: Water drains through pipe, B allowing wall to keep slope stable. Figure 8.28 Slapping concrete sheets on a steep, rubbly hillside in Rock Creek Valley, With creep or gradual soil flow, individual movements Montana, is not altogether effective; (B) photograph, taken several years are short-distance and the whole process is slow, so vegetation after (A), shows slipping and buckling, and telltale road repair below. may continue to grow in spite of the slippage. More detailed observation, however, can reveal the movement. For example, trees are biochemically “programmed” to grow vertically up- marked by swaths of downed trees. Records of the character of ward. If the soil in which trees are growing begins to creep past volcanic activity and an examination of a volcano’s typical downslope, tree trunks may be tilted, and this indicates the soil products can similarly be used to recognize a particular volca- movement. Further tree growth will continue to be vertical. If no’s tendency to produce pyroclastic flows. slow creep is prolonged over a considerable period of time, Very large slumps and slides may be less obvious, espe- curved tree trunks may result (figure 8.33A). (Trees growing in cially when viewed from the ground. The coherent nature of slanted fractures in rock, however, may develop curved trunks rock and soil movement in many slides means that vegetation through similar growth tendencies in the absence of creep; this growing atop the slide may not be greatly disturbed by the move- is a better indicator of movement in unconsolidated material.) ment. Aerial photography or high-quality topographic maps can Inanimate objects can reflect soil creep, too. Slanted utility be helpful here. In a regional overview, the mass movement of- poles and fences and the tilting-over of once-vertical grave- ten shows up very clearly, revealed by a scarp at the head of a stones or other monuments also indicate that the soil is moving slump or an area of hummocky, disrupted topography relative to (figure 8.33B). The ground surface itself may show cracks par- surrounding, more-stable areas (recall figure 8.17). allel to (across) the slope. Chapter Eight Mass Movements 189 mon24085_ch08_171-194.indd Page 190 11/24/09 9:20:56 PM user-s176 /Volumes/204/MHDQ173/mon24085/0073524085/mon24085_pagefiles Cables tightened by nuts at surface Unstable layers Cables anchored in stable rocks A A B Figure 8.30 B (A) Installation of rock bolts to stabilize a slope. The bolts are steel cables anchored in cement. Tightening nuts at the surface pulls Figure 8.31 unstable layers together and anchors them to the bedrock below. (A) A close look at the granite domes of Yosemite shows curved (B) Rock bolts used to stabilize a slope in the Bighorn Mountains, slabs of rock at the surface; some above valleys are poised to fall. Wyoming. (B) In 1996, a 200-ton block crashed down, shattering on impact Photograph © Doug Sherman/Geofile with a blast that leveled thousands of trees. (B) Photograph by Gerry Wieczorek, U.S. Geological Survey A prospective home buyer can look for additional signs erly may reflect a warped frame due to differential movement in that might indicate unstable land underneath (figure 8.34). the soil and foundation. Sliding may have caused leaky swim- Ground slippage may have caused cracks in driveways, garage ming or decorative pools, or broken utility lines or pipes. If move- floors, freestanding brick or concrete walls, or buildings; cracks ment has already been sufficient to cause obvious structural in walls or ceilings are especially suspicious in newer buildings damage, it is probable that the slope cannot be stabilized ade- that would not yet normally show the settling cracks common in quately, except perhaps at very great expense. Possible sliding old structures. Doors and windows that jam or do not close prop- should be investigated particularly on a site with more than 15% 190 Section Three Surface Processes mon24085_ch08_171-194.indd Page 191 11/24/09 9:21:10 PM user-s176 /Volumes/204/MHDQ173/mon24085/0073524085/mon24085_pagefiles A Bent and Younger Tilted posts A broken wall gravestone Tilted Older fence gravestone posts B Figure 8.33 (A) Tilted tree trunks are a consequence of creep, as seen here on a weathered slope in Bryce Canyon National Park. (B) Other signs of creep: tilted monuments, fences, utility poles. slope (15 meters of rise over 100 meters of horizontal distance), B on a site with much steeper slopes above or below it, or in any Figure 8.32 area where landslides are a recognized problem. (Given appropri- Areas prone to landslides may be recognized by the failure of ate conditions, slides may occur on quite shallow slopes.) vegetation to establish itself on unstable slopes. (A) Rock Creek Valley, Montana; note road snaking across slide-scarred surfaces. (B) This road was cut into the hillside to dodge around a bay on Landslide Warnings? the California coast, which probably contributed to the sliding In early 1982, severe rain-triggered landslides in the San Fran- now revealed by the fresh, raw slope above and the fresh asphalt cisco Bay area killed 25 people and caused over $66 million in on the road. damages. In response to this event, the U.S. Geological Survey Chapter Eight Mass Movements 191 mon24085_ch08_171-194.indd Page 192 11/24/09 9:21:26 PM user-s176 /Volumes/204/MHDQ173/mon24085/0073524085/mon24085_pagefiles began to develop a landslide warning system. The basis of the The system, though incomplete, was first tested in Febru- warning system was the development of quantitative relationships ary of 1986. Warnings were broadcast as special weather advi- among rainfall intensity (quantity of water per unit time), storm sories on local radio and television stations. These warnings duration, and a variety of slope and soil characteristics relating to were rather specific as to time, more general as to area at risk slope stability—slope angle, pore fluid pressure, shear strength, (necessarily so, given the complexity of the geology and terrain and so on. These relationships were formulated using statistical and lack of highly detailed data on individual small areas). analyses of observational data on past landslides. For a given Some local government agencies recommended evacuations, slope, it was possible to approximate threshold values of storm and many were able to plan emergency responses to the land- intensity and duration above which landsliding would become slides before they occurred. Many landslides did occur; total likely, given the recent precipitation history at the time (how satu- estimated landslide damage was $10 million, with one death. rated the ground was prior to the particular storm of concern). Of ten landslides for which the times of occurrence are known precisely, eight took place when forecast. Similar studies in the Seattle area have yielded general relationships between rainfall and the probability of landslides (figure 8.35). These allow local residents to anticipate when 6 P3, 3-day cumulative precipitation, 5 4 Landslides likely inches 3 Cumulative precipitation 2 threshold Landslides 1 unlikely 0 0 1 2 3 4 5 6 7 8 9 10 P15, 15-day cumulative precipitation before P3, inches A A 0.2 Threshold 0.18 Mean rainfall intensity,I, inches per hour March 1997 landslides 0.16 January 1986 landslides 0.14