Breeding for Resistance to Biotic Stresses in Plants PDF
Document Details
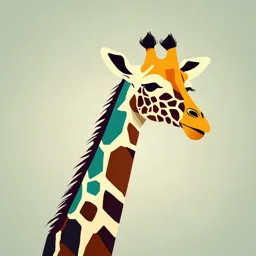
Uploaded by ExhilaratingPennywhistle
Sher-e-Kashmir University of Agricultural Sciences and Technology
2017
Naresh Kumar Bainsla, H.P. Meena
Tags
Summary
This chapter discusses breeding for resistance to biotic stresses in plants. It examines the significance of biotic stresses, sources of resistance, and different breeding approaches. The chapter also highlights the role of modern tools in disease and pest resistance breeding and the importance of resistance durability.
Full Transcript
See discussions, stats, and author profiles for this publication at: https://www.researchgate.net/publication/321796021 Breeding for Resistance to Biotic Stresses in Plants Chapter · December 2017 CITATIONS READS 9...
See discussions, stats, and author profiles for this publication at: https://www.researchgate.net/publication/321796021 Breeding for Resistance to Biotic Stresses in Plants Chapter · December 2017 CITATIONS READS 9 16,380 1 author: Hari Prakash Meena ICAR-Indian Institute of Oilseeds research, Hyderabad (Formerly: Directorate of Oilseeds Research) 102 PUBLICATIONS 514 CITATIONS SEE PROFILE All content following this page was uploaded by Hari Prakash Meena on 26 December 2017. The user has requested enhancement of the downloaded file. 2016, Recent Advances in Plant Stress Physiology Pages 379–411 Editors: Praduman Yadav, Sunil Kumar and Veena Jain Published by: Daya Publishing House, New Delhi 17 Breeding for Resistance to Biotic Stresses in Plants Naresh Kumar Bainsla1* and H.P. Meena2 1 Division of Field Crops, ICAR – Central Agricultural Research Institute, Port Blair, Andaman and Nicobar Islands 2 ICAR – Indian Institute of Oilseeds Research, Rajendranagar, Hyderabad – 500 030, India Abstract Biotic stresses cause significant loss in crop plants and management of biotic stresses (Diseases and pests) not only increases the cost of production but also has implications on environment and ecology. Increasing use of chemical agents for biotic stress management is concern for growers, exporters and animal and human health. The best method is to use resistant varieties which are economical, healthier and eco-friendly approach. Breeding for disease and pest resistance is major objective in most of the breeding programs across the crop species and world. The resistance breeding requires the resistant source or donor which may be the same species, related species of same genera or family, or altogether an alien species. There is need of recipient or target species and method of transfer of resistance. There are different approaches for breeding for resistance against different kinds of stress which involve both conventional and modern tools. Resistance breeding approach is to be at least one step ahead of the pathogen or pest in question. Achieving the goal of development of a resistant variety also needs attention on the durability of resistance for which the knowledge of resistance on the inheritance, expression and interaction with fellow genes and environment aspects. In this chapter emphasis has been given on importance of biotic stresses, inheritance, resistance sources, breeding approaches, and modern tools. ——————— *Corresponding author: E-mail: [email protected] 380 Recent Advances in Plant Stress Physiology Introduction Biotic stresses as the name suggests is the stress due to living or biotic factors. In the world of life there are different kinds of species which coexist on the planet earth. This coexistence is not that beautiful as it looks in first impression, in fact living beings do behave in numerous ways with the kinds of their own as well as other kinds of life in the presence of non living factors called environment. Therefore, there are mainly three factors of any life form i.e. Individual in question, other individuals of same or different species and environment. These three factors can interact in all sorts of permutations and combinations which decide the survival, longevity, reproduction and future of individuals. The relations can be mutually beneficial, parasitic, competitive and destructive with various degrees of expression. Plants like any other life form are also affected by biotic and abiotic factors. But here we are more concerned about the community or population of plants of same species grown for its economic use to benefit the mankind called crop. Crop plants will be referred to the scope of this chapter in terms of biotic stresses and breeding approaches. The breeding approaches shall cover the points of view on classical methods as well as modern tools. The biotic agents which cause biotic stresses include bacteria, nematodes, fungi, viruses, insect pests (crop as well as stored grain) and weeds. Each of the categories of these biotic agents has its own way of attacking or harming the crops. The stress may be air borne like in case of many rusts, seed borne as in case of smuts and bunts, soil borne like in case of many wilts, blights, nematodes or it may be transmitted through carriers which include insects and weeds. Weeds generally compete for space, water and nutrients with the crop plants and sometimes also act as secondary hosts to facilitate the other biotic agents to complete their life cycle. Different biotic agencies based upon the available circumstances can cause varying degree of losses to yield or quality of the plants and its produce. The minor reaction can be loss below economic threshold level wherein control of the disease or pest is not desirable. The losses can be higher causing significant loss in the yield or quality of the crop in a locality or sometimes so devastating that it can cause epidemic by spreading to larger areas even continent forcing to famine like situations. Therefore, degree of loss defines the economic importance of a disease or pest and accordingly called a minor for very low or major for significantly higher incidence of disease or pest. The importance of biotic stresses can be imagined from the facts epidemic spread of Phytopthora could result into Irish famine during nineteenth century. During the famine approximately 1 million people died and a million more emigrated from Ireland. Single outbreak of locusts could devastate the vegetation across the continents. The desert locust, the most notorious of about a dozen locust species for its ability to rapidly multiply and travel long distances, could threaten an area of 32 million square kilometres, stretching across 50 countries from west Africa to India. Similarly the rusts of wheat, mildew of maize and millets have caused serious losses to crops and affected a large number of human populations. The outbreak of Ug99 race of has shaken the world with its possible implications and many projects Breeding for Resistance to Biotic Stresses in Plants 381 involving huge money were started across the world in order to identify the source of resistance. It is generally thought that molecular biology is altogether different from biochemistry and similarly molecular genetics or so called biotechnology is some kind of super science which is different from genetics. In fact as the science grows the different terminologies also come in to knowledge but the relevance of new terms is actually stated by the basics behind it. For many beginners it sometimes becomes difficult to come to basics and understand the fundamentals behind each terminology which creates lot of confusion. Therefore efforts have been made to show the co linearity between the modern and classical terminologies and fundamentals. Further the chapter includes more of the general and common findings based upon the understanding like a common man rather than making it difficult to understand by including large number of references and complex definitions. What is Stress and Biotic Stress? Whenever any combination of an individual with the fellow individuals of same species or other species and environment becomes detrimental to its survival or reproduction or for future the individual is said to be in stress. If the major cause of this stress is from individuals of same species or any other species but definitely with the help of environment it will be known as biotic stress. Therefore biotic stress per se requires three factors to play role in its existence. Biotic factors affecting plants can be bacteria, fungi, virus, mycoplasma, insects, nematodes, rodents and even mammalian pests. But to cause a stress interaction of a susceptible host, aggressive or virulent pathogen or pest and favorable environment is must. Why Biotic Stress Resistant Varieties Required? Crop loss caused by plant pathogens have been reviewed extensively in a number of review articles over the past 50 years (Gaunt, 1995; James, 1974), beginning with Chester in 1950. However, throughout the world, approximately 800 million people are malnourished and the demand for food will increase as the population increases. It is predicted that by 2050 the world population will increase from approximately 6.7 billion to over 9 billion and that the current trend for more resource-intensive diets, which include more dairy and meat products, will continue. It is estimated that current global production of wheat must increase annually by about 2 per cent (Singh and Trethowan, 2007). At the same time, the demand for land from uses other than agriculture is increasing. Due to biotic stress up to 35 per cent of the total food production is lost. The estimated crop loss was of the value of Rs 90,000 crores in 2004 and in 2009, Rs. 1,40,000 crores (Suresh and Malathi, 2013). Overall, pests accounted for pre-harvest losses of 42 per cent of the potential value of output, with 15 per cent attributable to insects and 13 per cent each to weeds and pathogens. An additional 10 per cent of the potential value was lost postharvest (Orke et al., 1994). Out of a US$ 1.3 trillion annual food production capacity worldwide, the biotic stresses caused by insects, diseases and weeds cause 31-42 percent loss (US$ 500 billion), with an additional 6-20 percent (US$ 120 billion) lost post harvest to insects and to fungal and bacterial rots. Crop losses due to pathogens are often more severe in developing countries (e.g. cereals, 382 Recent Advances in Plant Stress Physiology 22 percent) when compared to crop losses in developing countries (e.g. cereals, 6 percent) (Oerke et al., 1994). Since more than 42 per cent of the potential world crop yield is lost owing to biotic stresses (15 per cent attributable to insects, 13 per cent to weeds, and 13 per cent to other pathogens), a reduction in this incidence will be one of the more important possibilities for improving plant production (Pimentel, 1997). Humans and insects have always competed for food and fibre, so they have been constantly at was with each other. Insects cause millions of dollars worth of losses annually to food crops and other plants all over the world. Crop losses due to insects and nematodes, estimated at 10–20 per cent for major crops, are signicant factors in limiting crop yields. The development of insect- and nematode resistant plants is therefore an important objective of plant breeding strategies with relevant implications for both farmers and the seed and agrochemical industries. In the United States in 1987, crop losses caused by diseases and insects in specific vegetables were, respectively: cole crops 9 and 13 per cent, lettuce 12 and 7 per cent, potato 20 and 6 per cent, tomato 21 and 7 per cent, sweet corn 8 and19 per cent, onion 21 and 4 per cent, cucumber 15 and 21 per cent, pea 23 and 4 per cent, and pepper 14 and 7 per cent. World-wide losses from diseases range from 9 per cent to 16 per cent in rice, wheat, barley, maize, potato, cotton, soybean (Chakraborty et al., 2000). If pest related losses are taken into consideration, it contributes to 14 to 25 per cent on average of the total global agricultural production (see DeVilliers and Hoisington, 2011). Estimated losses due to pests among some major crops were estimated to be 26 per cent for soybean, 28 per cent for wheat, 31 per cent for maize, 37 per cent for rice and 40 per cent for potatoes (Oerke and Dehne, 2004). The global loss potential caused by pests is particularly high in crops grown under high productivity environments and also in the tropics and sub-tropics where climatic conditions favour the damaging function of pests (Oerke, 2006). Furthermore, losses due to pathogens, animal pests and weeds were estimated to be 16, 18, and 34 per cent, respectively. Table 17.1: Overview of Potential and Actual Losses Attributable to Fungal and Bacterial Pathogens, Viruses, Animals Pests and Weeds as well as the Efficacy of the Applied Pest Control Operations in Maize, Wheat, Rice, Barley, Potatoes, Soybean, Sugar Beet, and Cotton Maize, Wheat, Rice, Barley, Potatoes, Soybean, Sugar Beet, and Cotton Pests and Pathogens Fungi and Viruses Animal Weeds Total Bacteria Pests Loss potential (per cent)a 14.9 3.1 17.6 31.8 67.4 Actual losses (per cent)a 9.9 2.7 10.1 9.4 32.0 Efficacy (per cent)b 33.8 12.9 42.4 70.6 52.5 Source: Modied from Oerke and Dehne (2004) a: As percentage of attainable yields; b: As percentage of loss potential prevented. Breeding for Resistance to Biotic Stresses in Plants 383 Past Research Achievements In upland cotton, variety MCU-5 is tolerant to verticilium wilt. In wheat, variety Rescue with solid stem is resistant to stem sawfly. It was released in Canada in 1946. In okra, variety Prabhani Kranti is resistant to yellow mosaic virus. In USA barley variety ‘Will’ is resistant to green bugs was released. In alfalfa, varieties ‘Cody, Moapa and Zia are resistant to alfalfa spotted aphid. These varieties were released in USA. In oat, variety Greta is resistant to stem eelworm. It was developed in Belgium. In upland cotton, varieties B-1007, Khandwa-2, SRT-1, DHY-286 and PKV-081 are resistant to jassids. Other cotton varieties Kanchana, Supriya and LK- 861 were resistant to whitefly. Intra-hirsutum cotton hybrid LHH-144 is resistant to leaf curl virus. It was developed from Punjab Agricultural University, Ludhiana. Susceptibility, Tolerance, Resistance, Immunity, Escape? Great Darwin’s theory of "survival of fittest" seems to be quite relevant to biotic stress but here comes the factor of the degree of stress. An individual may surrender and die due to stress (Susceptible) or may survive in stress and can bear the parasite for whole life (Tolerant) or can eliminate the parasite or pathogen or so called pest without affecting its performance (Resistant), the individual may show a hypersensitive or non acceptance of entry of pathogen or pest into its system (Immune) or it may acquire a mechanism to complete its cycle or go into dormant stage or protective state before the parasite causes the loss to life (Escape). Therefore all these situations are quite relative in terms of their degree but at the same time quite different in terms of mechanisms devised for healthy life. Plants have their own naturally devised system of defense such as pre-formed structures and compounds that contribute to resistance prior to immune response these may include plant cuticle/surface, plant cell walls, antimicrobial chemicals (for example: glucosides, saponins), antimicrobial proteins, Enzyme inhibitors, Detoxifying enzymes that break down pathogen-derived toxins, receptors that perceive pathogen presence and activate inducible plant defences. Plants do have inducible plant defences that are generated after infection these mechanisms involve cell wall reinforcement (callose, lignin, suberin, cell wall proteins), antimicrobial chemicals (including reactive oxygen species such as hydrogen peroxide, or peroxynitrite, or more complex phytoalexins such as genistein or camalexin), antimicrobial proteins such as defensins, thionins, or PR-1, antimicrobial enzymes such as chitinases, beta- glucanases, or peroxidases, hypersensitive response - a rapid host cell death response associated with defence mediated by “Resistance genes.”Endophyte assistance: Plant’s roots release chemicals that attract beneficial bacteria to fight off infections. Plant immune systems show some mechanistic similarities with the immune systems of insects and mammals, but also exhibit many plant-specific characteristics. Plants can sense the presence of pathogens and the effects of infection via different mechanisms than animals. As in most cellular responses to the environment, defences are activated when receptor proteins directly or indirectly detect pathogen presence and trigger ion channel gating, oxidative burst, cellular redox changes, protein kinase cascades, and many other responses that either directly activate cellular changes (such as cell wall reinforcement or the production of antimicrobial 384 Recent Advances in Plant Stress Physiology compounds), or activate changes in gene expression that then elevate plant defence responses. Two major types of pathogen detection systems are observed in plant immune systems: PAMP-Triggered Immunity (PTI; also known as MAMP-triggered immunity or MTI), and Effector-Triggered Immunity (ETI). The two systems detect different types of pathogen molecules, and tend to utilize different classes of plant receptor proteins to activate antimicrobial defences. Although many specific examples of plant-pathogen detection mechanisms are now known that defy clear classification as PTI or ETI, the larger trend across many well-studied plant-pathogen interactions supports continued use of PTI/ETI concepts. Treatment or Prevention? It is said that prevention is better than cure. But can we always depend upon the prevention or cure alone? We may have to opt for the suitable strategy to tackle the ailment through preventive as well as curative methods and at times combination of both the techniques seems suitable for minimizing the losses. The curative approaches wherever successful find the quickest control on the situation but not permanent in nature and are costly to the pocket of farmer as well to environment. The preventive methods however can be of mechanical, management and resistance and are time consuming but sustainable and eco-friendly and economic and the only solution in many cases. Plant Breeding and Biotic Stresses Plant breeding is the science which is a complex compound of art of culturing plants with skills of selection, standing on the platform of science of genetics at meta as well as micro/nano/pico or atomic level using statistics as its main tool may be through simple chi sqaures, random tables, calculators to high throughput computational software. In common man’s words we can say plant breeding is the system of getting educated young ones by virtue of education done to their parents. Plant breeding plays very important role in preventive, immunized and curative designer crop plants against biotic stresses. Plant breeding has been effective tool in controlling many diseases and insect pests wherein the chemical methods were too much costly to be affordable by a common farmer especially from poor countries. Guiding Principle for Development of Biotic Stress Resistant or Acclaimed Crop Plants The plants do have the inherent capacity to response against any kind of unwanted pressure due to environment or its biotic components. The genetic makeup of the plant makes it either resistant or susceptible. In a group of large number of plants of a species having sufficient chance of random segregation of alleles some plants are ought to be resistant as compared to other plants for a given stress. Similarly the pathogens do have complementary genes for virulence. Thus the guiding principle for the resistance in plants lies in genome of host as well as pathogen.The gene-for-gene relationship was discovered by Harold Henry Flor in 1942 who was working with rust (Melampsoralini) of flax (Linum usitatissimum). Flor was the first scientist to study the genetics of both the host and parasite and to integrate them into one genetic system (Flor 1942, 1947 and 1955). Gene-for-gene Breeding for Resistance to Biotic Stresses in Plants 385 relationships are a widespread and very important aspect of plant disease resistance. The combination of suitable genes makes it resistant while that of deleterious genes makes it susceptible. The inheritance of the resistance trait under study and its heritability is quite important in the development of a resistant variety or line. Based upon the inheritance and heritability of the resistance trait the breeding methodology can be prioritized for the given species which is further dependent on the pollination behaviour of species in question. The simply inherited genes are easier to transfer through directional selection in bi-parental crossing programmes.There are several different classes of R Genes. The major classes are the NBS-LRR genes and the cell surface pattern recognition receptors (PRR). The protein products of the NBS-LRR R genes contain a nucleotide binding site (NBS) and a leucine rich repeat (LRR). The protein products of the PRRs contain extracellular membrane, trans membrane and intracellular non-RD kinase domains. Within the NBS-LRR class of R genes are two subclasses the first class is Toll/Interleukin 1 receptor homology region (TIR) and has an amino-terminal. This includes the N resistance gene of tobacco against tobacco mosaic virus (TMV).The other subclass does not contain a TIR and instead has a leucine zipper region at its amino terminal. Plant Breeding for Disease Resistance Typically includes Identification of resistant breeding sources (plants that may be less desirable in other ways, but which carry a useful disease resistance trait). Ancient plant varieties and wild relatives are very important to preserve because they are the most common sources of enhanced plant disease resistance. Crossing of a desirable but disease-susceptible plant variety to another variety that is a source of resistance, to generate plant populations that mix and segregate for the traits of the parents. Growth of the breeding populations in a disease-conducive setting. This may require artificial inoculation of pathogen onto the plant population. Careful attention must be paid to the types of pathogen isolates that are present, as there can be significant variation the effectiveness of resistance against different isolates of the same pathogen species. Selection of disease-resistant individuals. Breeders try to sustain or improve numerous other plant traits related to plant yield and quality, including other disease resistance traits, while they are breed for improved resistance to any particular pathogen. Factors Affecting Breeding Methodology Breeding approach may vary from the point of view of different workers based upon the economic importance of stress, the stress in question, the genetics of resistance, availability of resistance sources, expertise and the facilities available etc. The most important from the point of view of a plant breeder is the genetics of resistance of the stress. There are numerous successful examples wherein the conventional breeding approaches have done wonders some of these include the resistant varieties against the rusts and smuts in wheat, downy mildew in maize 386 Recent Advances in Plant Stress Physiology and pearl millets. In fact the fruits of green revolution in India could be harvested amply due to conventionally bred rust resistant varieties in wheat. The change of cytoplasm in case of maize and pearl millet restored the hybrids in these crops. The breeding for monogenic or oligogenic traits with high heritability gives faster results as compared to the complex traits. The availability of resistance source is another factor which keeps its prime importance. Lack of proper resistance source against many insect pests has been the source of business to many pesticide industries with multimillion dollar turnovers. Later on the resistance source identified in totally unrelated organisms like Bacillus thuringiensis has again resulted to non- conventional answers to dreaded pests and weeds. Similarly the concept of non host resistance is also gaining importance. The approach may seem to be modern and non-conventional but the basics of genetics of resistance and the selection methods breeding tools still remain conventional. The first and foremost thing is to dissect the inheritance of resistance followed by identification of resistance source, selection of method to introgress the resistance in target germplasm and lastly to evaluate the final product. Sources of Disease Resistance A known variety: Resistant plants were isolated from commercial varieties in the cases of cabbage yellows in cabbage (Brassica oleracea var. capitata), currely top in resistant in sugar beet (Beta vulagaris L.) etc. Germplasm collection: Resistant to net bloch in barley (Hordeum vulgare L.), resistant to wilt in watermelon (Citrullus lanatus L.) etc. Related species: Prabhani Kranti a YVM resistant variety has been developed in which resistant is transferred from Abelmoschas album. Resistant to grassy stunt virus in rice (Oryza sativa L.) has been transferred from Oryza nivara. Mutation: Resistant to some disease may be obtained through mutations arising spontaneously or induced. Resistant to victoria blight in Oats (Avena sativa L.) was introduced by irradiation with X-ray or thermal neutrons. Somaclonal variation: Ono variety (a somaclonal from variety Pindar) of sugarcane (Saccharum officinarum L.) is resistant to Fiji disease. Unrelated organism: e.g. coat protein genes of a pathogenic virus, genes for novel phytoalexins. Breeding Methods for Biotic Stress Resistance Methods of breeding for disease resistance are, (1) selection, (2) introduction, (3) mutation, (4) hybridization (pedigree and backcross method), (5) somaclonal variation, and (6) genetic engineering. Selection Selection is the most ancient and basic procedure in plant breeding. Selection of biotic resistance plants from a commercial variety is the cheapest and the quickest method of developing a resistant variety. This method has been useful in many Breeding for Resistance to Biotic Stresses in Plants 387 cases in the past, but it has only a limited usefulness at the present level of crop improvement. For example, Kufri Red potato is a disease resistant selection from Darjeeling Red Round. Other such examples include resistance to curly-top in sugarbeets, to mildew and leaf spot in alfalfa, to cabbage yellows in cabbage and to Periconia root ro in sorghum. Pusa Sawani bhindi is a selection from a collection from Bihar; it was comparatively resistant to yellow mosaic under field conditions. Cotton (Gossypium hirsutum L.) variety MCU-1 (Madras, Combodia, Uganda-1) was selected from the variety Coimbatore-4 (CO-4) for resistance to black-arm; it had an acceptable level of resistance under field conditions. For example, selection for resistance to potato leafhopper and spotted alfalfa aphid in two broad based germplasms of alfalfa was highly effective in both the populations. A relatively recently released pigeonpea sterility virus (PSV) and wilt resistant variety of pigeon pea, Maviya Vikalp (MA3), is a pureline selection from material collected from a farmers field in Mirzapur (U.P.). Pusa Sawani okra variety is a YVM resistant selection from Bihar. Introduction Introductions have served as a useful method of disease control. Resistance varieties may be introduced for cultivation in a new area. This offers a relatively simple and quick means of obtaining resistant varieties. For example, Ridley wheat introduced from Australia had been useful as a rust resistant variety. Early varieties of groundnut introduced from USA were resistant to leaf spot or tikka disease. Kalyan Sona and Sonalika wheat varieties originated from the segregating materials introduced form CIMMYT, Mexico, and were rust resistant. Introductions also serve as sources of resistance in breeding programmes. For example, African pearl millet introductions have been used for developing Downey mildew resistant male sterile lines (Tift23 cytoplasm) for use in hybrid seed production. There are many examples of introduction of insect resistant varieties, the most striking of them being the introduction of D. Vitifoliae resistant grape root-stocks from USA into France; this saved the grape industry of France from virtual extinction. Ridley wheat variety introduced from Australia has been useful as a rust resistant variety. Mutation A mutagenic treatment may convert a susceptible genotype into a resistant one. If the mutation is a point mutation, the resistant mutant will be identical to the original cultivar, except for its resistance. Usually, however, there are undesirable side-effects of the mutagenic treatment. Several other genes may also have undergone changes, or the mutation for resistance has undesirable pleiotropic effects. As a consequence, the selection of a resistant mutant should be followed by further breeding efforts (i.e. backcrossing) to produce a commercially acceptable cultivar. The value of induced mutations is usually highlighted using the example of peppermint (Mentha piperita) cultivation in USA with annual value of 20 million U.S. dollars. This monoclonal crop became susceptible to Verticillium wilt, and its commercial cultivation was in jeopardy. A large scale mutation breeding project permitted the isolation of Verticillium wilt resistant mutant that had the same specific quality of peppermint oil as the parent variety; this saved the peppermint crop from 388 Recent Advances in Plant Stress Physiology being wiped out. Another impressive example of the economic success of mutant varieties is provided by the over 200 million Dutch florius chrysanthemum industry of the Netherlands; in 1981, mutants accounted for 35 per cent of the total sale of 600 million cuttings. Using γ-rays, amber grained mutants of Sonora-64 and Lerma Rojo were produced and released as Sharbati Sonora and Pusa Lerma respectively. In North America, one variety of common bean resistance to Anthracnose namely ‘Samilac’ was released by Down and Anderson in Michigan in 1956. Some other examples of disease resistance induced by mutagenic agents include resistance to stripe rust in wheat, crown rust in oats, mildew in barley, flax rust and leaf spot and stem rot resistance in pea nuts. Hybridization The most frequently employed plant breeding technique is hybridization. The aim of hybridization is to bring together desired traits found in different plant lines into one plant line via cross pollination. Hybridization is the most common method of breeding for disease resistance. It serves the following two chief purposes: (1) transfer of disease resistance from an agronomically undesirable variety to a susceptible but otherwise desirable variety (by back cross method), and (2) combining disease resistance and some other desirable characters of one variety with the superior characteristics of another variety (by pedigree method). In both these cases, one parent is selected for disease resistance; it should have a high degree of resistance to as many races of the pathogen as possible, and the resistance should be governed by few oligogenes. When the resistant variety is unadapted and agronomically undesirable, back cross method is the obvious choice. But when the resistant variety is well adapted and has some other desirable features as well, the pedigree method of breeding is preferred. Kufri Jyoti a prominent variety of potato developed by hybridization. It is resistant to late blight of potato. Kufri Khyati produces white oval tubers with shallow eyes, is moderately resistant to late blight, and is suitable for cultivation in plains of India. Pedigree Method Pedigree method consists of selecting individual F2 plants for desirable features, including resistance to diseases. Progenies of these selections are reselected in each succeeding generation until homozygosity is obtained. This is very common method used to develop improved cultivars to diseases. This method is quite suited for breeding for horizontal or polygenic resistance, for which backcross method is of limited value. Pedigree method for breeding of disease resistance is not materially different from the method used for other quantitative traits, e.g., yield. However, in breeding for disease resistance, artificial disease epidemics are generally created to enable an effective selection. A vast majority of disease resistant commercial varieties have been developed through this method e.g., Kalyan Sona, Sonalika, Malviya-12, Malviya-37, Malviya-206, Malviya-234, and many other wheat varieties. G. hirsutum variety Laxmi resistant to red leaf blight was developed using pedigree method from the cross between susceptible parent Gadag-1 and the resistant parent Coimbatore Combodia-2. Resistance to diseases such as anthracnose and bean common mosaic necrosis virus (BCMNV) have been successful, and in recent years Breeding for Resistance to Biotic Stresses in Plants 389 pedigree breeding has also used marker assisted selection to identify specific genes for disease resistance (Miklas et al., 2001). But an important limitation to pedigree selection is the amount of time needed (Fehr, 1987). Backcross Method This method is useful in transferring genes for resistance from a variety that is undesirable in agronomic characteristics to a susceptible variety, which is widely adapted and is agronomically highly desirable. The backcross programme would differ depending upon whether the allele for resistance is recessive or dominant to that for susceptibility. A rigid selection is done for disease resistance, and the plant type of recurrent parent is also selected for. In general, 4-6 backcrosses are made, but with an effective use of marker assisted selection three backcrosses may be adequate. At the end of backcross programme, the progeny are selfed and resistant plants are selected. Progenies derived from different homozygous resistant plants that are identical in agronomic characteristics are usually bulked to produce the new disease resistant variety. The new variety would be almost identical to the recurrent parent, except for the disease resistance; therefore, extensive yield trials are usually not required for its release. There are many examples of interspecific transfer of genes; “Transfer” was the first commercial wheat variety in which rust resistance was transferred from a related species. Rust resistance has been transferred to Kalyan Sona from several diverse sources, e.g., Robin, K-1, Bluebird, Tobari, Frecor, HS-19, etc., using backcross method. Three multiline varieties, viz., KSML-3, KSML-11 and KSML-7406, have been released for cultivation as a result of the above programmes using Kalyan Sona as recurrent parent and exotic lines resistance to leaf rust as non-recurrent parent. The Advantages of this method: 1) when resistant variety is unadapted and agronomically undesirable, backcross method is an obvious choice. 2) Useful to transfer one or few major genes (vertical resistance). 3) Extensive yield trials are usually not required before its release for commercial cultivation. 4) Multiple resistance breeding is possible. Disadvantage: No advancement in yield potential is possible. Incorporation of genes for rust resistance in Indian wheat varieties through backcross method led to the development of several near isogenic lines like HW 2004 (backcross line of C 306 carrying Lr24), HW 2044 (backcross line of PBW 226) for commercial cultivation. Somaclonal Variation Disease resistant somaclonal variants can be obtained in the following two ways. Firstly, plants regenerated from cultured cells or progeny of such plants are subjected to disease test and resistant plants are isolated (screening). Secondly, cultured cells are selected for resistance to the toxin or culture filtrate produced by the pathogen and plants are regenerated from the selected cells (cell selection). In most cases, these plants are also resistant to the disease in question. Cell selection strategy is most likely to be successful in cases where the toxin is involved in disease development. Genetic Engineering In this approach genes expected to confer disease resistance are isolated, cloned and transferred into the crop in question. In case of viral pathogens, several 390 Recent Advances in Plant Stress Physiology transgenes have been evaluated, viz., virus coat protein gene, DNA copy of viral satellite RNA, defective viral genome, antisense constructs of critical viral genes, and ribozymes. Viral coat protein gene approach seems to be the most successful (Singh, 2011), and a virus resistant transgenic variety of squash is in commercial cultivation in USA. Genes conferring insect resistance in plants have been transferred from B. thuringiensis (cry gene) and from other plants through genetic engineering. The cry gene transfers are the most successful, and insect resistant transgenic varieties of maize, soybean, cotton, etc. Expressing this gene is being cultivated in USA and other countries. In India, insect resistant Bt-cotton hybrids are in cultivation since 2002. Generations for Dissection of Inheritance Behaviour Generally the inheritance studies are done in early filial generation in case of biparental crosses. The half of the F1 seed can be used for knowing dominance of the trait, if all the F1 survive or resist than inheritance will be said to be governed by dominant alleles and if reverse is true then recessive alleles are the player. The F2 population is considered best for the study of inheritance but it should be large enough to represent all possible combinations of alleles. Some workers prefer to go for Back cross population but the practical limitation lies in case of crops where low seed set is there a large number of back crosses need to be attempted and then verified to represent a larger back cross population set. Use of F2 population also becomes tricky when we are dealing with destructive stress and there is no recovery of seed from the diseased or affected plant. The size of F2 or back cross population can be decided based upon the pollination behaviour of crops. For self pollinated crops where survival nature of species is balanced through high homozygosity level and resistance being highly heritable the population size can be just above the perfect F2 population size for number of genes in question. For example for single gene where three classes are supposed to be there and at least four plants are required but practically a population size of 30- 40 plants can be ideal in order to signify the results and sufficient statistical exercise. For two genes at least 16 plants are required but a population size of 60 to 70 is required for proper scoring. For three genes 64 plants are required but the population should have at least 200 individuals. Similarly for often cross pollinated crops where the survival of species lies in some degree of heterozygocity a fairly higher number (10 - 30 per cent) of plants is required for population. For cross pollinated crops the population size should always be higher side at least 1.5 times that of self pollinated crops. Odeny et al. (2009) conducted a study to determine the inheritance of resistance to Fusarium wilt in pigeonpea, which remains unknown, and to assess whether its genetic control would differ between African and Indian germplasm. Two resistant lines; one from African germplasm (ICEAP00040) and another of Indian origin (ICP8863) were used to make three different crosses (NPP670 x ICEAP00040, ICPL87091 x ICP8863, and KAT60/8 x ICP8863). Tests of F1, F2 and backcross generations under controlled conditions indicated involvement of one recessive gene in ICEAP0040 and 2 recessive genes in ICP8863. F1, F2 and backcross populations were developed by crossing resistant accessions (ICEAP 00554, ICEAP 00557) with susceptible accessions (KAT 60/8, ICP 7035). The Parents, F1, F2, backcrosses (BC1F1 and BC2F1) populations were Breeding for Resistance to Biotic Stresses in Plants 391 evaluated for Fusarium wilt resistance. F2 populations derived from ICEAP 00554 × KAT 60/8, ICEAP 00557 × KAT 60/8, ICEAP 00554 × ICP 7035, ICEAP 00557 × ICP 7035 crosses exhibited a 3:1 ratio which indicated that resistance to Fusarium wilt was under the control of major gene, however, a recessive gene was detected from ICP 7035 × KAT 60/8 cross. The genes detected could be valuable for wilt resistance breeding (Karimi et al., 2010). Most of the workers generally prefer the F1, F2 and back cross population for dissection of genetic behaviour and inheritance. However, use of F3 can be practiced wherein the stress is destructive and chances of losing transgressive segregants are more such as wilts. The representative progeny families comprising of 30- 50 plants from each F2 plant can be tested for phenotyping against the stress which by virtue of Hardy Weinberg Law depict the same results in a more decisive manner. Quantitative Inheritance and Resistance against Biotic Stresses Although the resistance traits are generally thought to be of qualitative nature suggesting in majority of cases the resistance is generally governed by monogenic to oligogenic inheritance. Yet the proper dissection of a monogenic trait also reveals the presence of subclasses when the stress scored in larger categories. Generally the biotic stresses or any kind of stress cannot be governed by a single gene if we go at biochemical level simply because the pathogen or pest also evolves in many forms. Resistance mechanism involves the pathway of several products to express the final reaction of resistance. Any breakdown at any step of pathway results in compromise in the degree of resistance. The monogenic and simply inherited trait of resistance is one way simpler to transfer but possess the chances of busting the resistance in the presence of altered pathotype and can cause epidemic due to vertical resistance reaction. A classical example of bacterial blight of rice can be taken, the resistance is pathotype specific and there are more than 30 gene (Xa/xa) having been discovered so far. The single gene concept is although valid for its transfer but resistance is not stable. Therefore efforts are being made to accumulate multiple genes to confer horizontal resistance. A detailed analysis of the genetic basis of resistance to powdery mildew in wheat revealed the presence of more than 15 QTLs. Moreover the qualitative inheritances do behave like quantitative when scored on a normal scale over the time. Resistance against Fusarium wilt in pigeonpea is thought to be of monogenic and digenic by many workers however when scored on percent scale the resistance behaved liked a quantitative trait (Kumar, 2010). Phenotyping of Biotic Stresses The phenotyping of any disease or any biotic stress is quite important aspect. Generally the grades of effect of the stress are decided by the experts who by and large are uniform for most of the diseases and insect pests. The diseases are scored on 0-5 or 1-9 scales or percent disease index. The stresses scored in different scales helps in making the categories or classes of immune, resistant, moderately resistant, moderately susceptible, susceptible etc. But sometimes the scores are given arbitrarily in nature and differ for person to person. In such cases importance should be given to more scientific and quantitative indices rather than qualitative scores. It is always better to score the stress on a normal curve rather than in discrete 392 Recent Advances in Plant Stress Physiology classes. The screening of germplasm or varieties against any biotic stress is also a very crucial step in most of the crop improvement programmes. The screening can be done in more than one ways depending upon the nature of stress and pathogen and available resources. Natural Screening The screening under natural conditions has been practiced in past in majority of the cases. But the major drawback of the natural screening is that there are high chances of error due to escape of otherwise susceptible host due to environment. This method is however quite useful in hot spots of stress where the chances of escape of are minimized due to continued presence of virulent pathogen or pest and congenial environment. This method requires least efforts and resources. This method is the only way in cases where the pathogen or pest cannot be cultured. Sick Plots Another method of screening of the stress is to use sick plot technique. This technique is very simple and effective provided the sick plots are maintained regularly. But this method is quite specific to race of pathogen as the same sick plot cannot be used for different pathovars and variants. This method requires initial efforts in development of sick plot but later can be used with ease. The test entries of different genotypes are planted in the sick plot in a requisite randomized design or augmented design where a large number of entries are there. The beauty of the system is that crop can be raised in similar way to normal crop except the ideal situation for the stress. The sick plot technique is quite effective in case of soil borne pathogens and pests. Sick plot techniques has been used in case of fungal, bacterial, nematodes, stem borers etc. Artificial Epiphytic Conditions Artificial inoculation is the best method as it minimizes the time, highly specific and efficient. This method is useful in case of pathogen and pests which can be cultured on specific media without losing the virulence. This method requires both skills and science and therefore resource demanding. The culture of the pathogen is maintained in laboratory and used for inoculating the test entries as and when required. The artificial inoculation technique examples are Xanthomonas oryzae pv oryzae isolates through leaf cutting methods in rice, leaf stappling technique in case of inducing viral infections in groundnut, Fusarium udum inoculation in pigeon pea. The cultures of pathogens can be maintained locally or requested from a laboratory where it is being maintained. Institute of Microbial Technology (IMTech), Chandigarh, India maintains the cultures of a variety of pathogens. Methods to Minimize the Time of Phenotyping All the methods mentioned above are very stage specific and require lot of time as one full generation of the plant is required. However, with the advances in diagnostic tools the phenotyping for stress can be highly specific and faster. Biochemistry has provided the solutions for faster assays of stresses by utilizing the basic property of metabolism of plants or pathogen or pest. Such assays provide an Breeding for Resistance to Biotic Stresses in Plants 393 early answer to screening a large number of samples. ELISA test for viral infections and presence of aflatoxins in seeds, Protein and Carbon analysis are few examples of daily diagnostic tools. Use of DNA or Protein based markers can significantly reduce the time and efforts in growing all the plants in field. DNA fingerprints and linked markers are now the tools for the selection of desired plant types. Molecular Approaches Molecular markers are useful in disease resistance breeding as they can substitute phenotypic screening in the early phase of breeding program and to identify resistant lines at juvenile stage to save time and cost of screening. It helps in easy identification and transfer of recessive genes and to monitor alien gene introgression, reduces the linkage drag and aids in eliminating undesirable traits in much shorter time frame than those expected through conventional breeding programs. It facilitates map-based cloning of disease resistance genes and pyramiding of genes for multiple disease resistance in a single cultivar, faster recovery of the recurrent parent genome in the backcross breeding programme (Tanksley et al., 1989). It could also reduce the need for phenotypic selection that may be inappropriate in identifying genotypic differences and in selection of rare recombinants between tightly linked resistance genes. Molecular markers offer great scope for improving the efficiency of conventional plant breeding. The essential requirements for developing MAS system are (i) availability of germplasm with substantially contrasting phenotypes for the traits of interest, (ii) highly accurate and precise screening techniques for phenotyping mapping population for the trait of interest, (iii) identification of flanking markers closely associated with the loci of interest and the flanking region on either side and (iv) simple robust DNA marker technology to facilitate rapid and cost-effective screening of large population (Paterson et al., 2004). The use of DNA marker systems, such as random amplified polymorphic DNAs (RAPDs) (Williams et al., 1990), amplified fragment length polymorphisms (AFLPs) (Vos et al., 1995), and simple sequence repeats (SSRs) (Akkaya et al., 1992), has contributed greatly to the development of genetic linkage maps for many important crop species including cowpea (Fatokun et al., 1993; Waugh et al., 1997). In combination with the bulked segregant analysis (BSA) method, (Michelmore and Meyers, 1998) the use of RAPDs, AFLPs, and SSRs has made it possible to rapidly identify molecular markers linked to genes of agronomic importance (Lee, 1995; Young, 1999). The development and use of molecular marker technologies has also facilitated the subsequent cloning and characterization of disease, insect, and pest resistance genes from a variety of plant species (Meyers et al., 1999). Tiwari et al. (1998) identified coupling and repulsion phase RAPD markers linked to powdery mildew resistant gene er-1 in pea using bulk segregant analysis of F3 individuals. Marker OPO-18 was found to be linked in coupling phase while, the markers OPE 16 and OPL 6 were in repulsion phase to resistant gene ER -1. Kotresh et al. (2006) identified RAPD markers associated with pigeonpea wilt using F2 population derived from contrasting parents GS l (susceptible), ICPL 87119 (resistant) and ICP 8863 (resistant). PCR testing revealed presence of two amplicons at 704 bp and 500 bp linked with susceptibility. Analysis of individual F2 plants showed a segregation 394 Recent Advances in Plant Stress Physiology ratio of 3:1 for the presence: absence of amplicons in the crosses. Selvi et al. (2006) identified three RAPD markers viz., OPT16, OPS7 and OPAK 19 specific to MYMV resistant parent and resistant bulk but absent in MYMV susceptible parent and susceptible bulk. From linkage analysis, one RAPD marker OPS 7900 was identified to be associated with mungbean yellow mosaic virus resistance. Ganapathyet al. (2009) used two AFLP primer pairs generating 4 markers (E-CAA/M-GTG150, E-CAA/M-GTG60, E-CAG/M-GCC120 and E-CAG/M-GCC150) which were polymorphic between the resistant and susceptible bulks indicating these markers are linked to SMD and located at a map distance of 5.7, 4.8, 5.2 and 20.7 cM respectively. The markers E-CAA/M-GTG150, E-CAA/M-GTG60 were linked in coupling phase to the susceptible dominant allele amplifying only in susceptible individuals which, can be effectively used for marker assisted selection. Trait Mapping With advancement in technology driven tools in modern Plant Breeding the use of map based approach are getting momentum. The resistance genes have been mapped in several crops and with the current advancements in high throughput and super throughput next generation sequencing facilities the genome wide information is available for consensus studies. The presence of the gene can be diagnosed using flanking DNA markers without waiting for the gene effect to be present in the phenotype (Paterson et al., 1991). Bulked segregant analysis (BSA), first described by Michelmore et al. (1991), is a method used to identify molecular markers linked to phenotypic traits controlled by single major genes. This method relies on the availability of bulked DNA samples collected from individuals that segregate for the two extreme divergent phenotypes within a single population. One bulk contains the DNA of the trait being targeted, while the other contains DNA from individuals lacking the trait. DNA Polymorphisms between the bulks are therefore, likely to be linked to genes that govern the trait. In lentil, this method has been used to identify markers that are tightly linked to genes for resistance to Fusarium, vascular wilt and Ascochyta blight (Chowdhury et al., 2001; Eujayl et al., 1998b; Ford et al., 1999). Eujayl et al. (1998b) used an RIL mapping population to identify molecular markers linked to the single dominant gene conditioning Fusarium vascular wilt resistance (Fw). They also identified a RAPD marker (OPS16750) that was 9.1 cM from the radiation-frost tolerance locus (Frt) (Eujayl et al., 1999). However, most probably due to insufficient genome map coverage, the Frtlocus and the linked RAPD marker were unable to be placed on the existing linkage map developed by Eujayl et al. (1998a). Ford et al. (1999) identified RAPD markers, RV01 and RB18, approximately 6 and 14 cM, respectively, from and flanking the foliar Ascochyta blight resistance locus Ral1 (AbR1) in ILL5588. These were subsequently converted to locus-specific sequence characterized amplified region (SCAR) markers and screened for applicability across parental lines in the Australian breeding program. Two RAPD markers, UBC2271290 and OPD-10870, were identified that flanked and were linked in repulsion phase to the resistance gene ral2 in the cultivar Indianhead at 12 and 16 cM, respectively (Chowdhury et al., 2001). Kotresh et al. (2006) identified RAPD markers associated with pigeonpea wilt using F2 population derived from contrasting parents GS l (susceptible), ICPL 87119 (resistant) and ICP 8863 (resistant). PCR testing revealed Breeding for Resistance to Biotic Stresses in Plants 395 presence of two amplicons at 704 bp and 500 bp linked with susceptibility. Analysis of individual F2 plants showed a segregation ratio of 3:1 for the presence: absence of amplicons in the crosses. Fusarium equiseti causes a discoloration on ginseng roots that significantly affects their marketability. The cellular and biochemical changes in affected roots that lead to this symptom, as well as differential gene expression following pathogen inoculation were studied. Marker-Assisted Selection and Trait Pyramiding Marker assisted selection (MAS) is the ability to select for and breed for a desirable trait with a marker, or suite of markers, from within a plant genotype without the need to express the associated phenotype. Marker assisted selection can be applied in such cases where linked markers are available. Therefore, MAS offers great opportunity for improved efficiency and effectiveness in the selection of plant genotypes with a desired combination of traits. This approach relies upon the establishment of a tight linkage between a molecular marker and the chromosomal location of the gene(s) governing the trait to be selected in a particular environment. Once this has been achieved, selection can be conducted in the laboratory and does not require the expression of the associated phenotype. For example, using MAS, disease resistance can be evaluated in the absence of the disease and in early stages of plant development. For example, Abenes et al. (1993) used MAS for selection of brown planthopper resistance (Bph3) and bacterial blight resistance (Xa21) using PCR-based markers in rice during F2 generation. Similarly, PCR-based MAS for Xa21 gene was employed by Reddy et al. (1997) in rice improvement program. Sequence tagged sites (STS) are ideal markers for MAS. STS markers are mapped loci for which all or part of the corresponding DNA sequence has been determined. The sequence information is used to design PCR primers for amplification of all or part of the original sequence. They are more robust and reproducible than the arbitrary sequences they are designed from, such as RAPD markers, as they are developed from the known sequences and produce an amplicon from longer primers. Differences in the lengths of amplified fragments serve as genetic markers for the locus. If no length polymorphism is detected, the amplified fragments can be cleaved with restriction enzymes to observe subsequent length differences. This technique is often referred to as cleaved amplified polymorphic sequences or CAPS (Jarvis et al., 1994). The use of converted locus-specific PCR markers is also referred to as a specific polymorphic locus amplification test (SPLAT), as well as sequence characterized amplified region (SCAR) markers and allele specific associated primer (ASAP) markers. SPLAT markers are designed from sequencing the insert of a polymorphic RFLP marker (Gale and Witcombe, 1992), whereas SCAR and ASAP markers are developed from sequencing specific RAPD markers (Gu et al., 1995; Ford et al., 1999; Paran and Michelmore, 1993). The conversion of more technically-demanding RFLP markers into PCR based markers (e.g. SPLAT) may provide a more rapid, cost-effective and efficient tool in lentil breeding. Pyramiding of multiple resistance genes to foliar fungal pathogens should provide a broader and more durable resistance, as similarly shown in rice against bacterial blight (Singh et al., 2001). Table 17.2: Selected Examples of Improved Lines/Cultivars/Varieties Developed with Resistance to Pest and Diseases through Molecular 396 Breeding Approaches in India and Abroad Crop Cultivar/Breeding Line/Parental Line Resistant to Trait Gene(s)/QTL (Q) Reference Rice (Oryza sativa L.) PR-106 Bacterial blight xa5 + xa13 + Xa21 Singh et al. (2001) Improved Pusa Basmati-1 Bacterial blight xa13 + Xa21 Gopalakrishnan et al., 2008 Samba Mahsuri Bacterial blight xa5 + xa13 + Xa21 Sundaram et al. (2008) RPBio-226 (IET 19046) and Bacterial blight xa5 + xa13 + Xa21 Sundaram et al. (2010) RPBio- 210 (IET 19045) Mahsuri Bacterial blight Xa4 + xa13+ xa5+ Xa21 Shanti et al. (2010) Restorer line R-8012 Bacterial blight and Blast Pi25/Xa21/xa13/xa5 Zhan et al. (2012) ADT-43 Bacterial blight xa5 + xa13 + Xa21 Perumalsamy et al. (2010) Pusa Basmati-1 Bacterial blight, blast xa13 + Xa21 + Pi54 + Singh et al. (2012) and sheath blight qSBR11-1 Pusa-6B and PRR-78 Bacterial blight xa13 + Xa21 Basavaraj et al. (2010) CO-39 Bacterial blight, blast Pi1 + Piz5 + Xa21 Narayanan et al. (2004) NSIC Rc142 and NSIC Rc154 Bacterial blight Xa4+xa5+Xa21 Toenniessen et al. (2003) PGMS 3418s Bacterial blight Xa21 Luo et al. (2003) Restorer lines R-8006 and -1176 Bacterial blight Xa21 Cao et al. (2003) Kang-4183 Bacterial blight Xa21 Luo et al. (2005) Pusa-1602 Blast Piz-5 Singh et al. (2012) Pusa-1603 Blast Pi55 Singh et al. (2012) h Pusa-6B and PRR-78 Blast Pi-K and Piz5 Prabhu et al. (2009) IR-50 Blast Piz Narayanan et al. (2002) Duokang #1, Phalguna Asian rice gall midge Gm-2, Gm-6(t) Katiyar et al. (2001) Improved Samba-Mahsuri (ISM) Asian rice gall midge Gm8 Sama et al. (2012) Contd... Recent Advances in Plant Stress Physiology Table 17.2–Contd... Crop Cultivar/Breeding Line/Parental Line Resistant to Trait Gene(s)/QTL (Q) Reference IIYou 8006 Bacterial blight Xa21 Wu et al. (2008) Guodao 3 Bacterial blight Xa21 Cao et al. (2006) RGD-7S/RGD-8S Blast Pi1, Pi2 Liu et al. (2008) Yueza 746/763 Blast Pi1, Pi2 Jin et al. (2007) Huahui 1 Insects Cry1Ac/Cry1Ab Liu et al. (2012) Bph68S/Luohong4A BPH Bph14, Bph15 Zhu et al. (2013) Ning 9108 Stripe blight Stv-bi, Wx-mq Yao et al. (2010) T16S Insects Bt Wu et al. (2010) TP309 Blast Pi9 Qu et al. (2006) Wheat Zak Stripe rust Yr15 Randhawa et al. (2009) (Triticum aestivum L.) HD-2329 Leaf rust Lr24 + Lr28 Kumar et al. (2010) Breeding for Resistance to Biotic Stresses in Plants Biointa-2004 Leaf rust Lr47 Bainotti et al. (2009) HD-2329 Leaf rust Lr9 + Lr24 + Lr28 Prabhu et al. (2009) PBW343 Leaf rust Lr24 + Lr48Lr28 + Lr48 Prabhu et al. (2009) Patwin Stripe and leaf rust Yr17 and Lr37 Helguera et al. (2005) UC-1113 (PI-638741) Stem rust (Ug99) Lr19 + Sr25 Yu et al. (2009) CM-137, CM-138, CM-139, Turcicum leaf blight and RppQ Prasanna et al. (2010a); CM-150and CM-151 Polysora rust Prasanna et al. (2010b) Mace Wheat streak mosaic virus Wsm-1 Graybosch et al. (2009) D3-8-3, D3-8-5 Cyst nematode CreX + CreY Barloy et al. (2007) Yang 158 Powdery mildew – Liu et al. (2000) Maize (Zea mays L.) NA QTLs on Corn borer resistance chrom. 7, 9 and 10 Willcox et al. (2002) Steptoe Stripe rust Bmy1 Toojinda et al. (1998) Contd... 397 Table 17.2–Contd... 398 Crop Cultivar/Breeding Line/Parental Line Resistant to Trait Gene(s)/QTL (Q) Reference Barley NA leaf rust Rphq6 van Berloo et al. (2001) (Hordeum vulgare L.) NA Barley yellow dwarf virus Yd2 Jefferies et al. (2003) DH-lines Barley yellow dwarf virus Ryd2 + Ryd3 Riedel et al. (2011) NA Yellow mosaic virus rym4 + rym5 + rym9+ rym11 Werner et al. (2005) HHB 67-improved Downy mildew – Hash et al. (2006) SloopSA Cyst nematode – Barr et al. (2000) Tango Stripe rust – Hayes et al. (2003) Igri BYDV – Scholz et al. (2009) Pearl Millet Nema TAM Nematode resistance Rma Simpson et al. (2003) Groundnut CR41-10 Cassava mosaic disease CMD2 Okogbenin et al. (2007) (Arachis hypogea L.) Cassava JTN-5503 Soybean cyst nematode rhg1 + Rhg4 + Rhg5 Arelli et al. (2006, 2007) Soybean JTN-5109 Soybean cyst nematode rhg1 + Rhg4 + Rhg5 Arelli and Young (2009) (Glycine max L.) Essex Soybean mosaic virus Rsv1 + Rsv3 + Rsv4 Maroof et al. (2008) S99-2281 frogeye leaf spot – Shannon et al. (2009) White Bean Verano Bean golden yellow – Beaver et al. (2008) mosaic virus Recent Advances in Plant Stress Physiology Breeding for Resistance to Biotic Stresses in Plants 399 Molecular breeding done for biotic stress resistance in India from 2005 to till 2009 (ICAR) (Table 17.3) and molecular breeding for biotic stress resistance in India from 2009 to till 2014 (DBT-GCP/ACIP) (Table 17.4). Some of the successful example mentioned below incorporating bacterial leaf blight resistance genes using marker aided selection in Indian rice cultivars viz., IR-24, IR-64, Samba Mashuri, PR-106, Tapaswini, Pusa Basmati-1, Lalat and Swarna. Using marker assisted selection two cereal cyst nematode genes (CreX and CreY) have been pyramided from Aegilops variabilis in a wheat background (Barloy et al., 2007). Table 17.3: ICAR-Molecular Breeding for Biotic Stress Resistance in India from 2005-2009 Centre Cultivar Genes for Resistance to Bacterial Blight Blast Gall Midge DRR, Hyderabad BPT-5204 xa13 + Xa21 Pi2 + Pi-kh Gm1 + Gm4 CRRI, Cuttack Tapaswani, Lalat, IR-64, Swarna xa13 + Xa21 Pi2 + Pi9 Gm1 + Gm4 IARI, New Delhi Pusa Basmati-1, Pusa6A/B, PRR-78 xa13 + Xa21 Pi-kh + Piz-5 Not required Table 17.4: DBT-GCP/ACIP-Molecular Breeding for Biotic Stress Resistance in India from 2009-2014 Name of the Target Varieties Bacterial Blast Gall Brown Plant Centres Blight Midge Hopper DRR, Hyderabad Sampada, Akshyadhan, xa13 + Pi-kh + GM1 + Bph13 + DRR-17B and RPHR-1005 Xa21 Pi9 GM4 Bph18 (Hybrid rice parental lines) IARI, New Delhi Pusa-1121 and Pusa-1401 xa13 + Piz-5 + – Bph-18 + Xa21 Pi-kh Bph-20 + Bph-21 PAU, Ludhiana PAU-201, PAU-3075-3-38 xa13 + – – Bph13 + and PAU-3105-45 Xa21 + Xa30 Bph18 Quantitative Trait Loci Mapping and Identification of Genes When a trait is governed by multiple and quantitative trait loci (QTL) and/or co-dominantly inherited genes, a more holistic genome mapping approach may be undertaken to identify genomic locations, interaction and subsequent molecular markers for accurate trait selection. QTLs have been detected in many crops but success in transfer of QTLs in desired background still remains a challenge. More than 80 independent QTL and 51 resistance genes from 62 different mapping populations were projected onto the consensus map of wheat for powdery mildew resistance (Marone et al., 2013). In fact QTL per se is not faulty rather the associated facts such as the biased populations to identify the QTLs. Wherein the QTL effects are overestimated due to lesser population size, ignoring outliers, less number of markers, ignoring small effect QTLs, QTL interactions with other QTLs and residual factors. In majority of the recent cases the information on QTLs is published based upon the catchy interpretations and smart work of software tools. A large number of workers interpret QTLs as additive effect of variance however, in biology two 400 Recent Advances in Plant Stress Physiology plus two does not necessarily mean four. A small effect QTL may be likely to be excluded when we think of QTL transfer but for a given population the effect of a large QTL may be induced by the presence of small effect QTL. The small effect QTLs may be different trans factors which say a lot about QTL X QTL interactions. Moreover linkage and QTL mapping is not any different science from classical biometrical plant breeding. As it was difficult for classical breeders to transfer the interaction component of variance it is so difficult to quantify and use interaction component of QTLs. Association Mapping Association or linkage disequilibrium (LD) mapping was used successfully to discover genetic determinants to traits initially in humans is now being used in plants (Flint-Garcia et al., 2003; Thornsbury et al., 2001). Using association mapping, entire genomes can be scanned for markers associated with qualitative and quantitative traits. The association mapping approach may allow plant breeders to break out of restrictive F1-derived mapping populations and employ any plant population including those from breeding programs or germplasm collections to conduct marker-trait association studies (Flint-Garcia et al., 2003). Gebhardt et al. (2004) clearly summarized the four potential benefits of the association mapping approach: (1) it allows assessment of the genetic potential of specific genotypes before phenotypic evaluation; (2) it allows the identification of superior trait alleles in germplasm; (3) it can assist in high resolution QTL mapping; and (4) it can be used to validate candidate genes responsible for individual traits (Gebhardt et al., 2004). The important issues to consider in designing and implementing any association mapping studies in plants are: (1) determination of the population structure (Pritchard et al., 2000); (2) estimation of nucleotide diversity (Zhu et al., 2003); (3) estimates of haplotype frequencies and LD (non random association of alleles at different loci) (Flint-Garcia et al., 2003); and (4) precise evaluation of phenotypes (Neale and Savolainen, 2004). Why genetic engineering for biotic stress: Availability of genetic variation in most of the crop species is one of the problems encountered by conventional breeders. The conventional approach as a whole is time consuming and labour intensive; undesirable genes are often transferred in combination with desirable ones; and reproductive barriers limit transfer of favourable alleles from inter specific and inter generic sources. Due to these reasons genetic engineering is being employed as a potential option worldwide for improving biotic stress tolerance. Breeder versus Molecular Breeder and Genetic Engineer It is a generally conception that breeder who goes to field and spends lot of time in hard work for doing crossing, selection, take data and then bring out the desired product in the form of a variety or breeding line and Molecular breeder on the contrary does his part of smart work while sitting on computer and doing experimentation in air conditioned laboratory. This is a matter of debate and concern that whether expressed genome which talks about the phenotype of a plant and a breeder relies on his skills for selection for desired plant out of a population of hundreds to millions of plants. And in laboratory a molecular breeder who relies Breeding for Resistance to Biotic Stresses in Plants 401 on single base pair (SNP) or few hundred base pairs (SSRs and STS) or on few thousand base pairs (RFLP, AFLP) based markers can do wonders in selection of plants. The matter is clear that if any marker system is closely linked to gene of interest with great heritability is as good as selection of desired plant in field through experience and acquired skills. As far as chances of mistake are not there both the system serve the purpose well. However, a great degree of confusion and efforts are there before getting the selection skills and developing a sure shot genomic tool or marker system for a given crop and trait. As far as science behind the two ways is concerned nothing is different and both systems religiously rely on genetic principles, statistical analysis and interpretation of data. The fact that laboratory based very good genotyping requires very good phenotyping as compulsory step to exclude false positives and proof reading and if one is good in phenotyping then there is no need of genotyping if the purpose is to just bring out the product. But to understand the basis of final product derived in field one has to go for genotyping or phenotyping at biochemical level. Genetic engineering mainly refers to the non conventional methods of designing a plant with one or more genes from quaternary gene pool through alien gene transfer methods using targeted approach. This science has shown its potential in terms of biotic stress resistance. Use of glyphosate resistance gene in different crops such as soybean and maize and use of Bt genes for resistance against lepidopteran pests has revolutionized the modern day Agriculture. Engineering a single or set of genes with modified cassette of promoter and controlling regions for target specific expression is the future of Plant Breeding. This technique has drastically reduced the dependence on harmful chemicals and pesticides. This technique although very effective yet has some reservations on its broad utilization mainly due to business behind it. The end user has to pay handsome royalty for the single gene working in the background of some half a million genes that is agronomically superior genotype which also involves the due consideration. Moreover certain issues pertaining to bio-safety and its regulation make hindrance in the use of this technology. War for economic gains between genetic engineering and chemical industry tycoons has misguided policymakers, farmers and scientific community and created propaganda for slow acceptance of technology. The next line of approach for a win- win situation will be targeting genes from plants which are wild relatives and possess resistance genes against biotic stresses. The use of plants from extreme climates such as mangroves, desserts and marshy lands can feed the bellies of ever- growing population. Multidisciplinary Approach The chances of success of getting a desired variety or plant type are multifold increased with the collective effort of a Plant Breeder, Molecular tools, Biochemist, Physiologist, Pathologist or Entomologist and a crop manager. Without the minimum company of these fellows desired product with full scientific dissection is not likely to come. The members of ideal team may opt to work together for faster results or they will have to work separately for considerably longer to time to reveal the portion of knowledge of their respective domain. A Plant Breeder looks at the phenotype of a plant with certain traits say disease resistant, high yielding, 402 Recent Advances in Plant Stress Physiology timely maturing and highly competitive plant. The pathologist has to ensure the type and degree of stress and its mechanism, Physiologist and biochemist has to go in to system of source to sink pathways, enzymes and system of plant as well as pathogen. A crop manager has to ensure a good crop with proper package of practices to express its fullest potential. Molecular Breeder with the help of markers and genomic tools can drastically reduce the generation time and can establish the linkages between genome and trait with mechanisms and functional properties. The genetic engineering approach can answer the problems of crossing incompatibilities and species borders. Utilization of Resistance Source The use of resistance source is the key factor wherein the breeding objective is to develop a resistant variety. Generally the resistance source for a particular biotic stress taken from the gene pool of biodiversity. Nature has provided the antidote of every problem and it is up to the Breeder where from he tries to extracts the resistant gene or genes. If the resistance is available in same species and varieties can be crossed easily it means the resistance source is primary gene pool. When the resistance source is available in another species of same genera and crossing can be done easily or with some limited difficulties which can be overcome by simpler methods the resistance source is said to be from secondary gene pool for example, Solanum torvum (a wild brinjal) is resistant to all kinds of soil borne diseases and is an excellent source of resistance for S. melongena (cultivated brinjal). Tertiary gene pool is the distantly related species with different genus but same family such gene pools cannot be used with classical methods of gene transfer and pose a great degree of difficulty. Such gene pool can be used with the help of bridging species which can cross with both resistant source as well as susceptible variety. When the resistance source is altogether different family or even kingdom and there is no relation between the donor and recipient species the source is said to be from quaternary gene pool. The classical example is the Bt gene from bacteria transferred in a number of crop species through genetic engineering. This area of science is getting momentum where in all the boundaries at genomic level are taken care through genetic engineering. Some workers also prefer to use mutation and somaclonal variation approaches for creating variability in the available germplasm these techniques are technically dependent on the chance of getting useful mutant and the frequency of such mutant is generally very low (1x 10–6 to 1x 10–9). But the science behind these techniques is also same that is change in the genome through deletions, repetitions, translocations or substitutions in the base pairs of a gene or its trans regulating factors which in turn modifies the expression profile of proteins or enzymes which can bring about the desired change. Challenges and Way Ahead The development of biotic stress resistant varieties particularly in NARS faces the basic challenges of lack of focus on utilization of secondary and tertiary gene pools from the great biodiversity vastly available in country. The monotony of public research efforts on utilization of resistance sources from patented technology has virtually hijacked the efforts for mining and developing our own resistance genes. Breeding for Resistance to Biotic Stresses in Plants 403 There are several reports on microbes to higher plants possessing resistance and genes. India being one of the largest hub of biotechnologists however hardly looking into the immense possibilities of utilization of resistance sources from related species, wild relatives and genes from extreme climatic plants. Shortage of technical people on taxonomy and systemics is another missing link to further utilization of our rich biodiversity. With the advancement in science of cross species gene transfer India can be leader with a record number of gene patents from indigenous flora and fauna. The concerted team efforts on silencing bad genes and knock out of faulty pathways and introgression of useful genes is the way ahead to fill the bellies of billions. References Akkaya, M.S., Bagwhatt, A.A. and Cregan, P.B. (1992). Length polymorphisms of simple sequence repeat DNA in soybean. Genetics, 132: 1131–1139. Arelli, P.R., Pantalone, V.R., Allen, F.L. and Mengistu, A. (2007). Registration of soybean germplasm JTN-5303. Journal of Plant Registrations, 1: 69-70. Arelli, P.R., Young, L.D. and Concibido, V.C. (2009). Inheritance of resistance in soybean PI 567516C to LY1 nematode population infecting cv. Hartwig. Euphytica, 165: 1-4. Arelli, P.R., Young, L.D. and Mengistu, A. (2006). Registration of high yielding and multiple disease resistant soybean germplasm JTN-5503. Crop Science, 46: 2723-2424. Bainotti, C.T., Fraschina, J., Salines, J., Nisi, J., Dubcovsky, J., Lewis, S., et al. (2009). Registration of ‘BIOINTA 2004’ wheat. Journal of Plant Registrations 3: 165-169. Barloy, D., Lemoine, J., Abelard, P., Tanguy, A.M., Rivoal, R. and Jahier, J. (2007). Marker-assisted pyramiding of two cereal cyst nematode resistance genes from Aegilops variabilis in wheat. Molecular Breeding, 20: 31-40. Barr, A.R., Jefferies, S.P., Warner, P., Moody, D.B., Chalmers, K.J. and Langridge, P. (2000). Marker-Assisted Selection in Theory and Practice. Prodeedings of the 8th International Barley Genetics Symposium, Vol I., Adelaide, Australia, 167-178. Basavaraj, S.H., Singh, V.K., Singh, A., Singh, A., Singh, A., Yadav, S., Ellur, R.K., Singh, D., Gopala Krishnan, S., Nagarajan, M., Mohapatra, T., Prabhu, K.V. and Singh, A.K. (2010). Marker-assisted improvement of bacterial blight resistance in parental lines of Pusa RH-10, a superfine grain aromatic rice hybrid. Molecular Breeding, DOI 10.1007/s11032-010-9407-3. Beaver, J.S., Porch, T.G. and Zapata, M. (2008). Registration of ‘Verano’ White Bean. Journal of Plant Registrations, 2: 187-189. Cao, L., He, L., Zhan, X., Zhuang, J. and Cheng, S. (2006). Guodao 3, a new hybrid rice combination with good quality, high yield and disease resistance. Hybrid Rice, 21:83–84. Cao, L.Y., Zhuang, J.Y., Zhan, X.D., Zheng, K.L. and Cheng, S.H. (2003). Hybrid rice resistant to acterial blight developed by marker assisted selection. Zhongguo Shuidao Kexue (Chinese Journal of Rice Science), 17(2): 184-186. 404 Recent Advances in Plant Stress Physiology Chakraborty, S., Tiedemann, A.V. and Teng, P.S. (2000). Climate change: potential impact on plant diseases. Environmental Pollution, 108: 317-326. Chester, K.S. (1950). Plant disease losses: their appraisal and interpretation. Plant Disease Report (Suppl.) 193: 189-362. Chowdhury, M.A., Andrahennadi, C.P., Slinkard, A.E. and Vandenberg, A. (2001). RAPD and SCAR markers for resistance to ascochyta blight in lentil. Euphytica, 118: 331–337. Daniela, M., Maria, A.R., Giovanni, L., Pasquale, D.V., Roberto, P., Antonio, B., Agata, G., Diego, R. and Mastrangelo, A.M. (2013). Genetic basis of qualitative and quantitative resistance to powdery mildew in wheat: from consensus regions to candidate genes. BMC Genomics, 14: 562. Down, E.E. and Anderson, A.L. (1956). Agronomic use of an X-ray induced mutant. Science, 124: 223-224. Eujayl, I., Baum, M., Erskine, W., Pehu, E. and Muehlbauer, F.J. (1997). The use of RAPD markers for lentil genetic mapping and the evaluation of distorted F2 segregation. Euphytica, 96: 405–412. Eujayl, I., Baum, M., Powell, W., Erskine, W. and Pehu, E. (1998a). A genetic linkage map of lentil (Lens sp.) based onRAPDand AFLP markers using recombinant inbred lines. Theoretical and Applied Genetics, 97: 83–89. Eujayl, I., Erskine, W., Baum, M. and Pehu, E. (1999). Inheritance and linkage analysis of frost injury in lentil. Crop Science, 39: 639–642. Eujayl, I., Erskine, W., Bayaa, B., Baum, M. and Pehu, E. (1998b). Fusarium vascular wilt in lentil: Inheritance and identification of DNA markers for resistance. Plant Breeding, 117: 497–499. Fatokun, C.A., Danesh, D., Menancio-Hautea, D.I. and Young, N.D. (1993). A linkage map for cowpea [Vignaunguiculata(L) Walp] based on DNA markers (2n = 22). In Genetic maps 1992: A compilation of linkage and restriction maps of genetically studied organisms. Edited by J.S. O’Brien. Cold Spring Harbour Laboratory Press, New York. pp. 6256–6258. Flint-Garcia, S.A., Thornsberry, J.M. and Buckler, E.S. (2003). Structure of linkage disequilibrium in plants. Annual Review of Plant Biology, 54: 357– 374. Flor, H.H. (1942). Inheritance of pathogenicity in Melampsoralini. Phytopathology, 32: 653–669. Flor, H.H. (1947). Inheritance of reaction to rust in flax. Journal of Agricultural Research, 74: 241–262. Flor, H.H. (1955). Host-parasite interaction in flax rust - its genetics and other implications. Phytopathology, 45: 680–685. Ford, R., Pang, E.C.K. and Taylor, P.W.J. (1999). Genetics of resistance to ascochyta blight (A. lentis) of lentil and the identification of closely linked RAPD markers. Theoretical and Applied Genetics, 98: 93–98. Breeding for Resistance to Biotic Stresses in Plants 405 Frederick, J., Muehlbauer, S.C., Sarker Ashutosh, McPhee Kevin, E., Coyne Clarice, J., Rajesh, P.N. and Ford, R. (2006). Application of biotechnology in breeding lentil for resistance to biotic and abiotic stress. Euphytica, 147: 149–165. Gale, M.D. and Witcombe, J.R. (1992). DNA markers and marker mediated applications in plant breeding, with particular reference to pearl millet breeding. In: J.P. Moss (Ed.), Biotechnology and Crop Improvement in Asia, pp. 323–332. ICRISAT, Patancheru. Ganapathy, K.N., Gowda Byre, M., Venkatesha, S.C., Rama Chandra, R., Gnanesh, B.N. and Girish, G. (2009). Identification of AFLP markers linked to sterility mosaic disease in pigeonpea Cajanus cajan(L.) Millsp. International Journal of Integrative Biology, 7: 145-149. Gaunt, R.E. (1995). The relationship between plant disease severity and yield. Annual Review of Phytopathology, 33: 119. Gebhardt, C., Ballvora, A., Walkemeir, B., Oberhagemann, P. and Schuler, K. (2004). Assessing genetic potential in germplasm collections of crop plants by marker-trait association: A case study for potatoes with quantitative variation of resistance to late blight and maturity type. Molecular Breeding, 13: 93–102. Gopalakrishnan, S., Sharma, R.K., Rajkumar, K.A., Joseph, M., Singh, V.P., Singh, A.K., et al. (2008). Integrating marker assisted background analysis with foreground selection for identification of superior bacterial blight resistant recombinants in Basmati rice. Plant Breeding, 127: 131-139. Graybosch, R.A., Peterson, C.J., Baenziger, P.S., Baltensperger, D.D., Nelson, L.A., Jin, Y, et al. (2009). Registration of ‘Mace’ hard red winter wheat. Journal of Plant Registrations, 3: 51-56. Gu, W.K., Weeden, N.F., Yu, J. and Wallace, D.H. (1995). Large-scale, cost-effective screening of PCR products in marker-assisted selection applications. Theoretical and Applied Genetics, 91: 465–470. Hash, C.T., Sharma, A., Kolesnikova-Allen, M.A., Singh, S.D. Thakur, R.P. Raj, A.G.B., et al. (2006). Teamwork delivers biotechnology products to Indian small-holder crop-livestock producers: Pearl millet hybrid “HHB 67 Improved” enters seed delivery pipeline. Journal of SAT Agricultural Research, 2: 1-3. Hayes, P.M., Corey, A.E., Mundt, C., Toojinda, T. and Vivar, H. (2003). Registration of ‘Tango’ Barley. Crop Science, 43: 729-731. Helguera, M., Vanzetti, L., Soria, M., Khan, I. A., Kolmer, J. and Dubcovsky, J. (2005). PCR Markers for Triticum speltoides Leaf Rust Resistance Gene Lr51 and Their Use to Develop Isogenic Hard Red Spring Wheat Lines. Crop Science, 45: 728-734. James, W.C. (1974). Assessment of plant diseases and losses. Annual Review of Phytopathology, 12: 27. Jarvis, P., Lister, C., Szab´O, V. and Dean, C. (1994). Integration of CAPS markers into the RFLP map generated using recombinant inbred lines of Arabidopsis thaliana. Plant Molecular Biology, 24: 685– 687. 406 Recent Advances in Plant Stress Physiology Jefferies, S.P, King, B.J., Barr, A.R., Warner, P, Logue, S.J. and Langridge, P. (2003). Marker-assisted backcross introgression of the Yd2 gene conferring resistance to barley yellow dwarf virus in barley. Plant Breeding, 122: 52-56. Jin, S., Liu, W., Zhu, X., Wang, F., Li, J., Liu, Z., Liao, Y., Zhu, M., Huang, H. and Liu, Y. (2007). Improving blast resistance of a thermo-sensitive genic male sterile line GD-8S by molecular marker- assisted selection. Chinese Journal of Rice Science, 21:599–604. Karimi, R., Owuoche James, O. and Silim, S.N. (2010). Inheritance of fusarium wilt resistance in pigeonpea [Cajanus cajan (L.) Millspaugh]. Indian Journal of Genetics and Plant Breeding, 70(3): 271-276. Katiyar, S,K, Tan, Y., Huang, B., Chandel, G., Xu, Y., Zhang, Y. et al. (2001). Molecular mapping of gene Gm-6(t) which confers resistance against four biotypes of Asian rice gall midge in China. Theoretical and Applied Genetics, 103: 953-961. Kotresh, H., Fakrudin, B., Punnuri, S.M., Rajkumar, B.K., Thudi, M., Paramesh, H., Lohithswa, H. and Kuruvinashetti, M.S. (2006). Identification of two RAPD markers genetically linked to a recessive allele of a fusarium wilt resistance gene in pigeonpea (Cajanus cajan L. Millsp.). Euphytica, 149: 113 – 120. Kumar, J., Mir, R.R., Kumar, N., Kumar, A., Mohan, A., Prabhu, K.V., Balyan, H. S. and Gupta, P. K. (2010). Marker assisted selection for pre-harvest sprouting tolerance and leaf rust resistance in bread wheat. Plant Breeding, 129: 617-621. Kumar, N. (2010). Molecular mapping of Fusarium wilt in pigeonpea Cajanus cajan L. Millsp. PhD thesis, CCSHAU, Hisar. Lee, M. (1995). DNA markers and plant breeding programs. Advance Agronomy, 55: 265–344. Liu, J., Liu, D., Tao, W., Li, W., Wang, C.P., Cheng, S. and Gao, D. (2000). Molecular marker-facilitated pyramiding of different genes for powdery mildew resistance in wheat. Plant Breeding, 119: 21–24. Liu, N., Jiang, L., Zhang, W., Liu, L., Zhai, H. and Wan, J. (2008). Role of embryo LOX3 Gene under adversity stress in rice (Oryza sativa L.). Chinese Journal of Rice Science, 22:8–14. Liu, Z., Zhao, J., Li, Y., Zhang, W., Jian, G. and Peng, Y Qi, F. (2012). Non uniform distribution pattern for differentially expressed genes of transgenic rice huahui 1 at different developmental stages and environments. PLoS One, 7:e37078. Luo, Y.C., Wang, S.H., Li, C.Q., Wang, D.Z., Wu, S. and Du, S.Y. (2003). Breeding of the photo-period-sensitive genetic male sterile line ‘3418S’ resistant to bacterial bright in Rice by molecular marker assisted selection rice. Zuowu Xuebao (Acta Agronomica Sinica), 29(3): 402-407. Luo, Y.C., Wang, S.H., Li, C.Q., Wang, D.Z., Wu, S. and Du, S.Y. (2005). Improvement of bacterial blight resistance by molecular marker-assistedselection in a wide compatibility restorer line of hybrid rice. Zhongguo Shuidao Kexue (Chinese Journal of Rice Science), 19(1): 36-40. Breeding for Resistance to Biotic Stresses in Plants 407 Marone D., Maria, A. R., Giovanni L., Pasquale D.V., Roberto P., Antonio B., Agata G., Diego R. and Mastrangelo, A.M. (2013). Genetic basis of qualitative and quantitative resistance to powdery mildew in wheat: from consensus regions to candidate genes. BMC Genomics, 14:562. Maroof, S., Jeong, S.C., Gunduz, I., Tucker, D.M., Buss, G.R. and Tolin, S.A. (2008). Pyramiding of soybean mosaic virus resistance genes by marker-assisted selection. Crop Science, 48: 517-526. Meyers, B.C., Dickerman, A.W., Michelmore, R.W., Sivaramakrishnam, S., Sobral, B.W. and Young, N.D. (1999). Plant disease resistance genes encode members of an ancient and diverse protein family within the nucleotide-binding super family. Plant Journal, 20: 317–332. Michelmore, R.W. and Meyers, B.C. (1998). Clusters of resistance genes evolve by divergent selection and a birth and death process. Genome Research, 8: 1113–1130. Michelmore, R.W., Paran, I. and Kesseli, R.B. (1991). Identification of markers linked to disease-resistance genes by bulked segregant analysis: A rapid method to detect markers in specific genomic regions by using segregating populations. PNAS (USA) 88: 9828– 9832. Narayanan, N.N., Baisakh, N., Cruz, V., Gnanamanickam, S.S., Datta, K. and Datta, S.K. (2002). Molecular breeding for the development of blast and bacterial blight resistance in rice cv. IR50. Crop Science, 42: 2072-2079. Narayanan, N.N., Baisakh, N., Oliva, N.P., Vera Cruz, C.M., Gnanamanickam, S.S. and Datta, K. (2004). Molecular breeding: marker-assisted selection combined with biolistic transformation for blast and bacterial blight resistance in indica rice (cv. CO39). Molecular Breeding, 14: 61-71. Neale, D.B. and Savolainen, O. (2004).Association genetics of complex traits in conifers. Trends in Plant Science, 325–330. Odeny, D.A., Jayashree, B., Gebhardt, C. and Crouch, J. (2009). New microsatellite markers for pigeonpea (Cajanus cajan(L.) millsp.). BMC Research Notes 2: 35. Oerke, E.C. (2006). Crop losses to pests. Indian Journal of Agricultural Sciences, 144: 31-43. Okogbenin, E., Porto, M.C.M., Egesi, C., Mba, C., Espinosa, E., Santos, L.G., Ospina, C., Marín, J., Barrera, E., Gutiérrez, J., Ekanayake, I., Iglesias, C. and Fregene, M.A. (2007). Marker assisted introgression of resistance to cassava mosaic disease into Latin American germplasm for the genetic improvement of cassava in Africa. Crop Science, 47: 1895-1904. Paran, I. and Michelmore, R.W. (1993). Development of reliable PCR based markers linked to downy mildewresistance genes in lettuce. Theoretical and Applied Genetics, 85: 985–993. Paterson, A.H., Stalker, H.T., Gallo-Meagher, M., Burrow, M.D., Dwivedi, S.L., Crouch, J. H. and Mace, E.S. (2004). Genomics and Genetic Enhancement 408 Recent Advances in Plant Stress Physiology of Peanut. In: Legume Crop Genomics. (Eds.) Wilson, R. F., Stalker, H. T. and Brummer, E. C., Champaign, III: AOCS Press. pp. 97-109. Paterson, A.H., Tanksley, S.D. and Sorrells, M.E. (1991). DNA markers in plant improvement. Advance Agronomy, 46: 39–90. Perumalsamy, S., Bharani, M., Sudha, M., Nagarajan, P., Arul, L., Saraswathi, R., Balasubramanian, P. and Ramalingam, J. (2010). Functional marker-assisted selection for bacterial leaf blight resistance genes in rice (Oryza sativa L.). Plant Breeding, 129: 400-406. Prabhu, K.V., Singh, A.K., Basavaraj, S.H., Cherukuri, D. P., Charpe, A., Gopala Krishnan, S., Gupta, S.K., Joseph, M., Koul, S., Mohapatra, T., Pallavi, J.K., Samsampour, D., Singh, A., Vikas K. Singh, Singh, A. and Singh, V.P. (2009). Marker assisted selection for biotic stress resistance in wheat and rice. Indian Journal of Genetics, 69(4) (Spl. issue): 305-314. Prasanna, B.M., Hettiarachchi, K., Mahatman, K., Rajan, A., Singh, O.N., Kaur, B., Kumar, B., Gowda, K.T.P., Pant, S.K. and Kumar, S. (2010). Molecular marker- assisted pyramiding of genes conferring resistance to Turcicum leaf blight and Polysora rust in maize inbred lines in India. In: Zaidi PH, Azrai M, Pixley KN, eds. Maize for Asia: Emerging trends and technologies. Proceedings of 10th Asian Regional Maize Workshop, Makassar, Indonesia 20–23 October 2008. Mexico: CIMMYT. Prasanna, B.M., Pixley, K., Warburton, M.L. and Xie, C. (20