Model Organisms for Genetic Analysis - BMN1003/L07 PDF
Document Details
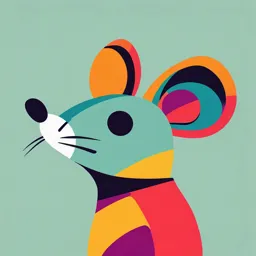
Uploaded by WellRoundedNobelium7192
Newcastle University Medicine Malaysia
Pamela Knight
Tags
Summary
This lecture summarizes model organisms used in genetic analysis, covering types, characteristics, and applications in research. It also touches on issues of generating and manipulating different model organisms. Examples include yeast, flies, worms, fish, and mice, outlining their significance in studying various biological processes and diseases.
Full Transcript
BMN1003/L07 Genetics Lecture 7: Model eukaryotic organisms for genetic analysis Dr. Pamela Knight Senior Lecturer (Non-Clinical) Biomedical Sciences NUMed Malaysia Email: [email protected]...
BMN1003/L07 Genetics Lecture 7: Model eukaryotic organisms for genetic analysis Dr. Pamela Knight Senior Lecturer (Non-Clinical) Biomedical Sciences NUMed Malaysia Email: [email protected] 1 What we cover in this session: What are model organisms understanding importance in biomedical science Examples of model organisms- know key differences between models (Advantages & Limitations) – life cycles – reproductive differences – how they are manipulated Considerations when choosing a model organism (discussion) 2 What are model organisms? 3 What is a model organism? A well established experimental biological system Characteristics: Rapid rate of development (to maturity) Easily genetically manipulated Some can be transformed with foreign DNA Short life span (life cycle) Readily available (source / stocks) Large numbers offspring per generation Easy to maintain within a laboratory setting (in an appropriate environment for the organism) 4 Types of Model Organisms Experimental May not be genetically amenable but have certain other advantages e.g. Xenopus (clawed toad) 5 Types of Model Organisms Genomic Occupy a special position in evolution or have a particular genome size or composition e.g. Fugu rubripes (pufferfish), Octopus bimaculoides 6 https://www.nature.com/articles/nature14668 Types of Model Organisms Genetic- species amenable to genetic analysis short generation time and breed in large numbers which allows large scale genetic crosses availability of range of mutations and/or tools to make mutations detailed genetic maps, sequenced genome 7 Gene knockout: gene sequences are completely or partially removed and gene expression is completely eliminated mRNA Protein Protein absent product gene gene Wild type knockout Gene knockdown: techniques that reduce/interfere with the expression of the gene e.g. RNA interference, morpholinos inhibition mRNA mRNA Greatly Protein reduced product protein gene gene Wild type knockdown 8 Why do we need model organisms? 9 Why not just study humans? Ethical issues!!! - so must be limited to: clinical observations/sampling only- with permission (limited, highly variable) or Approved clinical trials (e.g. a vaccine/drug trial - once previous work has shown the intervention is safe for human use) or voluntary participation in a study which doesn’t cause stress or harm (e.g. filling out a questionnaire!) 10 What about tissue culture? “refine, reduce + replace”- substituting in vivo with in vitro models Limitations: Can investigate cells, not organs/ tissues Doesn’t represent an organ system/ living system- ie effect on a whole organism of a pathogen, drug etc. Can’t study genetic components! 11 Examples of model organisms 12 Eukaryotic Models for Genetic Analysis Yeast: S. cerevisiae & S. pombe Fly: Drosophila Worm: C. elegans Fish: Zebrafish Mouse 13 Homologue A gene related to a another gene by descent from a common ancestral DNA sequence Can be Orthologues- genes in different species that evolved from a common ancestral gene. Normally, orthologues retain the same function in the course of evolution Paralogues - genes separated by the event of genetic duplication within a genome 14 Yeast S. cerevisiae & S. pombe Small, well-documented genome Easy to grow in the lab Possible to do ‘rescue’ experiments 15 Yeast Unicellular, microscopic eukaryotes Linear chromosomes within a membrane bound nucleus Divide by budding or fission The two major yeast genetic models are: Saccharomyces cerevisiae – budding yeast Schizosaccharomyces pombe - fission yeast 16 Saccharomyces cerevisiae (Baker’s or Brewer’s yeast) Model since the 1930s, intensively studied Small size and simple growth and storage conditions Rapid growth rate (80 min/gen) Can exist as diploid or haploid cells First eukaryotic genome sequence (1996) 12.8 Mb genome ~6000 genes 17 Saccharomyces cerevisiae (Baker’s or Brewer’s yeast) Extremely easy to genetically manipulate: Sexual cycle (enable large scale genetic crosses) Large mutant collections (e.g. deletion libraries) Deletion or integration of DNA happens relatively easily Easily transformable with plasmids 18 Saccharomyces cerevisiae (Baker’s or Brewer’s yeast) shares many basic biological properties with humans: cell division cycle gene expression DNA replication and repair S. cerevisiae budding Dr Gordon Beakes @Uni Ncl Cell signaling 20% of human disease genes have orthologues in yeast Lee Hartwell - Nobel Prize 2001 for studies using budding yeast to dissect the eukaryotic cell division cycle (BMN2006) 19 Schizosaccharomyces pombe Fission yeast (splits to divide) Nuclear genome with 3 linear chromosomes ~14 Mbp with ~4970 genes Has all the same research advantages as S. cerevisiae Small size, rapid growth, sexual cycle for crosses, easily genetic manipulation, easy to transform, many mutants BUT importantly S. pombe and S. cerevisiae are highly evolutionarily divergent 20 Other fungal ‘models’ Neurospora crassa 21 Other fungal ‘models’ Neurospora crassa Red bread mould, 40 Mb genome Used to study circadian rhythm Aspergillus nidulans Filamentous fungus belonging to the family of ascomycetes Genome sequenced in 2005, 30 Mbp and has approx 9,500 genes Has been used to study a wide range of processes 22 Drosophila Drosophila melanogaster, fruit fly A genetic model for > 100 years. Small ~3mm, large numbers can be cheaply maintained Life cycle approx. 2 weeks, females can lay up to 100 eggs per day Genome sequenced in 2001, 165 Mbp, encoding ~14,000 genes 3 pairs of autosomal chromosomes plus X and Y sex chromosomes 23 Drosophila Genetic advantages easy to cross a huge number of mutants are available many phenotypic markers (e.g. eye colour) mature larvae produce giant polytene chromosomes which allow genetic mapping transformable using P element transposon 24 Contributions from Drosophila Genetics Cell Signaling: Study of signalling identified & characterised pathways involved in oncogenesis (e.g. Ras/MAPK, Notch, Wnt/Wingless) Development: Analysis of fly embryonic development has provided much insight into development in humans Neurological Diseases: development of neurological disease models is providing insight into human neurological conditions, e.g. Huntingtons, Alzheimers, Parkinsons 25 Drosophila Neurological Disease Models Disease Model: a mutant model organism that mimics the phenotypes/features observed in a human disease (see also later lectures) studies of fly mutants provide information about: normal role that a protein plays in cells how this function is altered in the human disease 26 Drosophila Neurological Disease Models e.g. Spinal Muscular Atrophy (SMA): Neurodegenerative disease - progressive deterioration of motor functions and loss of motor neurons mutations in human SMN genes Drosophila strains carrying mutations orthologous SMN gene have phenotypes analogous to the human pathology e.g. reduced viability and decreased motility as well as muscular atrophy in the adult thorax 27 QUICK BREAK: What would you have to consider when choosing a model organism? (your points here)… 28 Caenorhabditis elegans Well mapped an understood genome Fate of cells in development known Many mutants available Easy to keep You will be meeting these in a See BMN1011/D/P02 practical soon! 29 Caenorhabditis elegans Small (~1 mm) free living, non parasitic soil nematode Transparent, allows easy observation and manipulation Feeds on bacteria (e.g. E. coli) , can be cheaply maintained and cultivated (up to 10,000 worms petri dish) Short life cycle, egg-to-egg takes approx 3 days 30 Caenorhabditis elegans Short lifespan 2-3 weeks (good for ageing studies) Two sexes - hermaphrodites and males (allows genetic crossing) Extremely fecund, hermaphrodites can produce about 300 offspring 31 Caenorhabditis elegans Genome sequenced in 1997 (first multicellular eukaryote) 97 Mbp, five pairs of autosomal chromosomes one pair of sex chromosomes ~20,000 genes (40% have human orthologues) 32 Caenorhabditis elegans Genetic advantages: easy to perform genetic crosses many mutants available transformable genes can be knocked down using RNA interference 33 C. elegans RNAi screens (each dsRNA targets a different worm gene) 34 Caenorhabditis elegans C. elegans has provided huge insight into many processes Ageing and especially development Wild type worms have a constant 959 cells with a fixed position Made it possible to track cells and follow them through development The complete lineage of every cell was determined 2002 Nobel prize was awarded to Brenner, Horvitz and Sulston 35 Zebrafish Danio rerio ray finned bony fish native to freshwater rivers in South Asia Great for development studies as fry are transparent Well established (>25yrs) 36 Zebrafish Lay ~ 200 eggs per week = ~35,000 eggs (2 - 4 years) Sexually mature by 3- 4 months old, a generation interval of 2- 3 months Transparent embryos, can see changes 37 Zebrafish Genome and Manipulation 1.7 Gbp genome - 25 chromosomes Complex sequence; repeat sequences and embryonic DNA polymorphisms Sturdy 1mm embryos, micro- injectable (can develop in a microtitre plate – external to parent) 38 Zebrafish Genome and Manipulation Possible to make mutants by random mutagenesis (large scale screens undertaken) Knockdown of expression of specific genes is possible using morpholinos = Small oligomers of synthetic nucleotide analogues that block gene expression Excellent model for: Vertebrate development (organs etc.) Neurobiology Drug discovery and toxicity testing Many human diseases including cancer 39 Mouse A lab favourite... Many years of data Many models of disease states available Relatively easy to keep 40 Mouse Mus musculus: common house mouse Many inbred lab strains Model most closely related to humans (genome size and content) out of these examples Sequenced 2002, ~25,000 genes on 20 chromosomes (2.6 billion bases) 13,000 orthologous genes aligned (mice - human) = 78.5% identity for amino acids 41 Mouse Genetics 2 month breeding cycle, 6-15 offspring per litter Inbreeding = offspring with identical gene composition (homozygous loci) Manipulation of embryonic stem cells allows production of transgenic or gene-knockout mice Transgenic - additional foreign DNA deliberately inserted into their genome (same in every cell) Gene-knockout - a specific gene is inactivated, ("knocked out“) by replacing it or disrupting it with an artificial piece of DNA (same in every cell) Both can be used to study gene function/ regulation and to model human diseases…. 42 Mouse Genetics Generating transgenic or gene-knockout mice: Pronuclear Microinjection: The foreign DNA is introduced directly into the mouse egg just after fertilisation Introduction of DNA into embryonic stem cells (ES cells): ES cells from the very early mouse embryo differentiate into all types of cell when introduced into another embryo have the same, artificially introduced mutation in every cell which abolishes the activity of the gene of interest 43 Mouse Models Mice can be prone to: cancers, blindness, obesity, diabetes, drug addiction, alcoholism, aggression, anxiety, Huntington disease Good for studying human disease/ traits There are many commercially available research mice Ethical considerations- most countries have legislation on use of animal models/ genetic manipulation 44 Other Vertebrate Models African clawed frog: Xenopus laevis very large cells, injectable oocytes, is a tetraploid (4 sets chromasomes) Used for studying cell cycle and signalling Brown rat: Rattus novegicus Alternative to mice used for neurological & toxicity studies 45 Revision guidelines Can you define a model organism? Do you know why these are used? Can you list the key features of a model organism? Can you remember the key model invertebrates, vertebrates, microbial and plant models? Do you know the key differences between these model organisms i.e. do you what their specific advantages and limitations are? Can you recognise how they help us understand humans genetics (in terms of: ageing, cell cycle, disease etc?) Do you know the definitions of homologue, orthologue and paralogue? 46 In Conclusion “....... Keep in mind that understanding how a gene controls a process in yeast is relevant to understanding the same gene and the same process in normal human cells” William Klug, Michael Cummings and Charlotte Spencer "Concepts of Genetics" (2006) 47 Further reading Molecular Biology of the Cell 6th Edition (Garland Science): in Chapter 8 (Analysing and Manipulating DNA): Studying Gene Expression and Function Hard copies in the LRC or use the direct link: (need uni login) https://libsearch.ncl.ac.uk/primo-explore/fulldisplay?docid=NCL_ALMA51148399790002411&context=L&vid=NEWU I&lang=en_US&search_scope=NotPC&adaptor=Local%20Search%20Engine&isFrbr=true&tab=default_tab&query= any,contains,Molecular%20Biology%20of%20the%20Cell&sortby=date&facet=frbrgroupid,include,3074405341&offs et=0 48 Further reading Welcome Trust: The Big Picture: https://www.stem.org.uk/system/files/elibrary-resources/2019/12/mod el%20organisms%20for%20research_final.pdf 49 Any Questions? Dr. Pamela Knight Room 3.44, Bell’s Court Tel: + 607 555 3800 extn. 3867 Email or Discussion Board Email: [email protected] 50