Biosignaling - Chapter 12 PDF
Document Details
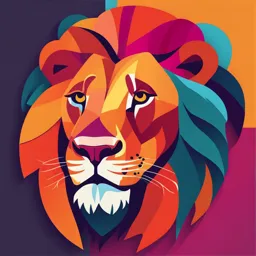
Uploaded by OptimisticDulcimer9673
University of Illinois at Chicago
Yury Polikanov
Tags
Summary
This chapter details the mechanisms of biosignaling in cells, focusing on signal transduction and relevant receptors. It describes the role of different types of receptors, including GPCRs and enzyme-linked receptors, and explores the concept of secondary messengers.
Full Transcript
University of Illinois at Chicago College of Liberal Arts and Sciences Department of Biological Sciences CHAPTER 12 LEHNINGER PRINCIPLES OF BIOCHEMISTRY: BIOSIGNALING by Prof. Yury Polikanov Introductory Biochemistry BIOS-352/CHEM-352 ...
University of Illinois at Chicago College of Liberal Arts and Sciences Department of Biological Sciences CHAPTER 12 LEHNINGER PRINCIPLES OF BIOCHEMISTRY: BIOSIGNALING by Prof. Yury Polikanov Introductory Biochemistry BIOS-352/CHEM-352 Chapter 12 | Biosignaling © 2013 W. H. Freeman and Company General Features of Signal Transduction All cells have specific and highly sensitive signal- transducing mechanisms, which have been conserved during evolution A wide variety of stimuli act through specific protein receptors in the plasma membrane The receptors bind the signal molecule and initiate a process that amplifies the signal, integrates it with input from other receptors, and transmits the information throughout the cell Biological Role of Signal Transduction Cells receive signals from the environment beyond the plasma membrane Hormones Neurotransmitters Pheromones Antigens Light Touch These signals cause changes in the cell’s composition and function Differentiation and antibody production Growth in size or strength Sexual vs. asexual cell division Main Features of Signal-Transducing Systems Signaling Through the Plasma Membrane Ligands include small organic Receptors are protein or molecules, ions, peptides, proteins, and glycoprotein molecules polysaccharides Receptors A membrane-bound or soluble protein or protein complex, which exerts a physiological effect after binding its natural ligand G-protein coupled receptors (GPCRs) Epinephrine (adrenalin) receptor Enzyme-linked receptors Insulin receptor Ligand-gated ion channels Nicotinic acetylcholine receptor Other membrane receptors Integrin receptors Nuclear receptors Steroid hormone receptors Receptors Bind Specific Ligands Receptors Bind Specific Ligands Typical ligands are: Small inorganic ions (ferric ion binds to bacterial ferric receptor) Organic molecules (adrenalin binds to epinephrine receptor) Polysaccharides (heparin: fibroblast growth factor) Peptides (insulin binds to insulin receptor) Proteins (various growth factors bind to their receptors) Most Common Signal-Transduction Systems G-protein coupled receptors (GPCRs) Interact with G-proteins and activate enzymes that generate intracellular second messengers. This type of receptor is illustrated by the β-adrenergic receptor system that detects epinephrine. Receptor tyrosine kinases Plasma membrane receptors that are also enzymes. When one of these receptors is activated by its extracellular ligand, it catalyzes the phosphorylation of several cytosolic or plasma membrane proteins. Insulin receptor and receptor for epidermal growth factor (EGFR) are the examples. Receptor guanylyl cyclases Plasma membrane receptors with an enzymatic cytoplasmic domain. The intracellular second messenger for these receptors, cyclic guanosine monophosphate (cGMP), activates a cytosolic protein kinase that phosphorylates cellular proteins and thereby changes their activities. Most Common Signal-Transduction Systems Gated ion channels Open and close (hence the term “gated”) in response to the binding of chemical ligands or changes in transmembrane potential. These are the simplest signal transducers. The acetylcholine receptor ion channel is an example of this mechanism. Adhesion receptors Interact with macromolecular components of the extracellular matrix (such as collagen) and convey instructions to the cytoskeletal system about cell migration or adherence to the matrix. Integrins illustrate this general type of transduction mechanism. Nuclear receptors Bind specific ligands, such as steroid hormones, and alter the rate at which specific genes are transcribed and translated into cellular proteins. G-Protein Coupled Signaling G-Protein Coupled Receptors (GPCRs) are α-helical integral membrane proteins G-proteins are heterotrimeric (αβγ) membrane-associated proteins that bind GTP/GDP G-proteins mediate signal transduction from GPCRs to other target proteins G-Protein Coupled Receptors (GPCRs) Share a common structural arrangement of seven transmembrane α- helices and act through heterotrimeric G proteins Upon ligand binding, GPCRs catalyze the exchange of GTP for GDP on the G protein, causing dissociation of the Gα subunit from Gβγ Gα stimulates or inhibits the activity of an effector enzyme, changing the level of its second-messenger product Sensing Epinephrine Signal via GPCR Epinephrine: The “Fight or Flight” Hormone Hormone made in adrenal glands (pair of organs on top of kidneys) Mediates stress response: mobilization of energy Binding to receptors in muscle or liver cells induces breakdown of glycogen Binding to receptors in adipose cells induces lipid hydrolysis Binding to receptors in heart cells increases heart rate Sensing the Epinephrine Signal via GPCR Cyclic AMP (cAMP) Serves as a Secondary Messenger Allosterically activates cAMP-dependent protein kinase A (PKA) Activation of PKA leads to the activation of enzymes that produce glucose from glycogen Cyclic AMP (cAMP) is a potent secondary messenger Activation of cAMP-dependent Protein Kinase (PKA) Activation of cAMP-dependent Protein Kinase (PKA) A Kinase Anchoring Protein Signal Amplification in Epinephrine Cascade Activation of few GPCRs leads to the activation of few adenylyl cyclase enzymes Every active adenylyl cyclase enzyme makes several cAMP molecules, thus activating several PKA enzymes These activate thousands of glycogen-degrading enzymes in the liver tissue At the end, tens of thousands of glucose molecules are released to the bloodstream Sensing of Epinephrine via GPCR can be Modulated Epinephrine analogs Agonists – structural analogs of a hormone that bind to its receptor and mimic the effects of its natural ligands Antagonists – structural analogs of a hormone that bind to its receptor without triggering the normal effect and thereby blocking the effects of agonists, including the natural ligands Self-Inactivation in G-protein Signaling Epinephrine is meant to be a short-acting signal The organism must stop decomposition of glycogen and production of glucose if there is no more need to “fight or fly” Down-regulation of cAMP production occurs by the hydrolysis of GTP in the α subunit of the G-protein cAMP-phosphodiesterase hydrolyses remaining cAMP Desensitization of β-Adrenergic Receptors cAMP is a Common Secondary Messenger A large number of GPCRs mediate their effects via cAMP (both activating and inhibiting cAMP synthesis) The human genome encodes about 1000 GPCRs (with ligands such as hormones, growth factors, and neurotransmitters) There are also hundreds of different GPCRs that can be responsible for similar processes (such as taste or smell) Ligands for many GPCRs have yet to be identified Some bacterial toxins can inactivate G-proteins Cholera toxin and pertussis toxin transfer ADP-ribose from NAD onto α subunit of G protein Adenylate cyclase is now constitutively active and produces too much cAMP from ATP GPCRs can use other secondary messenger molecules Inositol-1,4,5-triphosphate (IP3) Calcium ions Calcium ions as secondary messenger molecules Enzyme-linked Membrane Receptors Many membrane receptors consists of: Extracellular ligand-binding domain Intracellular catalytic domain Receptor tyrosine kinases (RTKs) External ligand-binding site Internal tyrosine kinase domain(s) Often dimerize on ligand binding Self-activate by autophosphorylation Kinase then phosphorylates targets Receptor guanylate cyclases External ligand-binding site Internal guanylate cyclase domain Convert GTP to cyclic GMP (cGMP) Important regulator of cellular [Ca2+] Insulin: Hormone for Glucose Uptake Insulin is a peptide hormone that is produced by the β-cells of islets of Langerhans in the pancreas Insulin is produced and released from the pancreas in response to nutrients such as glucose Insulin reaches target cells, such as liver, muscle, or fat tissue cells via bloodstream Binding of insulin to the insulin receptor initiates a cascade of events that leads to increased glucose uptake and metabolism Inability to make or sense insulin leads to diabetes Glucose Import in Myocytes Insulin Signaling Cascade Insulin binding to the extracellular domains of the receptor activates the catalytic domain inside the cell Catalytic domain in one receptor phosphorylates Tyr residues in another receptor Receptor auto-phosphorylation allows binding and phosphorylation of protein IRS-1 Insulin Signaling Cascade Indirect interaction of phosphorylated IRS with protein Ras initiates a series of protein phosphorylations ERK, one of the phosphorylated protein kinases, enters the nucleus Transcription factor ELK1 becomes phosphorylated and stimulates the expression of specific genes, such as glucose transporter (GLUT4) Receptor Guanylyl Cyclases Catalytic domain converts GTP to cGMP Works through activation of protein kinase G (PKG) Membranes are Electrically Polarized The inside of the cell is typically negatively charged compared to the outside –50 to –70 mV The membrane potential is largely due to electrogenic Na/K- ATPase 3 Na+ are pumped out 2 K+ are pumped in Flow of ions across the membrane depends on its concentration gradient and overall electrical potential Gated Ion Channels in Nerve Signaling Voltage-gated channels Open when potential difference reaches a specific threshold Ligand-gated channels Open when specific signaling molecule binds to recognition site Important in nerves, muscle Na+, K+, and Ca2+ channels Nicotinic acetylcholine receptor Ionotropic glutamate receptor Gamma-aminobutyric acid (GABA) receptor Gated Ion Channels in Nerve Signaling Nerve signals within nerves propagate as electrical impulses Propagation of the impulse involves opening of voltage gated Na+-channels Opening of voltage-gated Ca2+ channels at the end of the axon triggers the release of neurotransmitter acetylcholine Acetylcholine opens the ligand- gated ion channel on the receiving cell Voltage-Gated Sodium Channel Voltage-Gated Sodium Channel Ligand-Gated Sodium Channel: Acetylcholine Receptor Nicotinic acetylcholine receptor is a ligand-gated sodium channel Ion channel for influx of Na+, Ca2+ Gate opens in response to acetylcholine binding Acetylcholine-esterase terminates signalling Ligand-Gated Sodium Channel: Acetylcholine Receptor Integrins mediate cell adhesion Direct Regulation of Transcription by Hormones Bacterial chemotaxis is controlled by enzyme-coupled receptors Signaling Pathways in Organisms SUMMARY Environmental signals can be physical or chemical Cells sense and respond to signals by means of receptors Major biological signaling systems include G protein-coupled receptors, enzyme-linked receptors, and gated ion channels Vision, smell, and taste are sensed by GPCRs, which bind GTP and activate interacting G-proteins Heterotrimeric G-proteins function as molecular switches Insulin exerts its effects through a receptor tyrosine kinase (RTK) Voltage- and ligand-gated ion channels are the basis of electrochemical signaling in nerve and muscle tissue Disregulation of intracellular signaling cascades can lead to cancer