Biology Study Notes PDF
Document Details
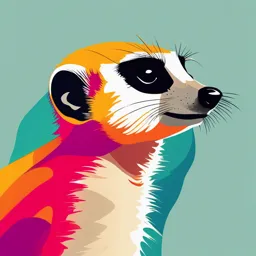
Uploaded by AppreciatedCornflower
I.P.E.T. N°260
Tags
Summary
These notes provide an overview of cell biology, specifically differentiating prokaryotic and eukaryotic cells. The text details characteristics, structures, and functions of various cellular components and organelles.
Full Transcript
Biology Study Notes Preliminary Examinations Module One: Cells as the Basis of Life What distinguishes one cell from another? ❖ investigate different cellular structures, including but not limited to: examining a variety of prokaryotic and eu...
Biology Study Notes Preliminary Examinations Module One: Cells as the Basis of Life What distinguishes one cell from another? ❖ investigate different cellular structures, including but not limited to: examining a variety of prokaryotic and eukaryotic cells describe a range of technologies that are used to determine a cell’s structure and function ❖ investigate a variety of prokaryotic and eukaryotic cell structures, including but not limited to: drawing scaled diagrams of a variety of cells comparing and contrasting different cell organelles and arrangements modelling the structure and function of the fluid mosaic model of the cell membrane Section One: What Distinguishes One Cell from Another Cells are the fundamental units of life, and they can vary significantly in structure and function. The key factors that distinguish one cell from another include: ❖ Cell Type: Prokaryotic Cells: These cells are simple cells without a nucleus or membrane bound organelles. Examples include bacteria and archaea. Characteristic Prokaryotic Cells Genetic Material Single, circular DNA molecule (nucleoid) Membrane-bound Organelles Absent Cell Wall Composition Typically have a peptidoglycan cell wall Size Generally smaller (1-5 micrometres) Examples Bacteria, Archaea Eukaryotic Cells: These cells have a true nucleus and membrane bound organelles. Examples include plant, animal and fungi cells. Characteristic Eukaryotic Cells Genetic Material Linear DNA organised into multiple chromosomes Membrane-bound Organelles Present (eg. nucleus, mitochondria, ER etc.) Cell Wall Composition Variable (eg. cellulose, chitin, none etc.) Size Generally larger (10-100 micrometres) Examples Animals, plants, fungi, protists ❖ Cell Size: Cells can vary in size from micrometres to millimetres. For example, typical animal cells are around 10-30 micrometers in diameter, while some plant cells can be larger, like the elongated cells of a celery stalk. ❖ Cell Shape: Cell shapes can vary greatly. Some common shapes include spherical (e.g., blood cells), cuboidal (e.g., liver cells), columnar (e.g., intestinal cells), and elongated (e.g., muscle cells). ❖ Cell Function: Cells specialise in different functions. For instance, red blood cells are specialised for oxygen transport, while nerve cells (neurons) are specialised for transmitting electrical signals. Section Two: Cellular Structures As mentioned above, Prokaryotic Cells are simpler, and lack membrane-bound organelles. Below is a table outlining the key features in Prokaryotic Cells: Key Feature Description Examples Cell Wall Provides structural support and protection. Bacterial cell walls Plasma Membrane Regulates the papssage of molecules in and out. Phospholipid bilayer Cytoplasm Contains cellular components and enzymes. Various proteins, DNA Nucleoid Region with the bacterial chromosome. Single circular DNA Flagella Whip-like structures for movement. Bacterial Flagella Ribosomes Sites of protein synthesis. 70S ribosomes In comparison, Eukaryotic Cells are more complex and compartmentalised. Below is a table outlining the key features in Prokaryotic Cells: Key Feature Description Examples Nucleus Contains DNA and controls cell Genetic information storage and activities. regulation Endoplasmic Reticulum Synthesises proteins and lipids. Rough ER (with ribosomes) and Smooth ER Golgi Apparatus Modifies, sorts, and packages Protein and lipid processing and molecules. trafficking Mitochondria Produces ATP (energy) through Powerhouse of the cell cellular respiration. Chloroplasts (in plants) Site of photosynthesis. Converts sunlight energy into chemical energy Vacuole (in plants) Stores water, nutrients, and waste. Maintains turgor pressure and storage Lysosomes Contains enzymes for digestion. Breaks down cellular waste and foreign material Drawing Scaled Diagrams of Cells: Creating scaled diagrams of cells helps illustrate their unique structures. For instance, a simple representation of an animal cell might include the nucleus, cell membrane, mitochondria, and endoplasmic reticulum. Section Three: Technologies for Studying Cellular Structures Modern biology relies on a range of technologies to investigate cell structure and function. These technologies provide valuable insights into cellular processes. Some prominent techniques include: Technology Description Applications Light Microscopy Uses visible light to magnify and Identifying cell structures. observe cells. Electron Microscopy Uses electron beams to achieve Detailed subcellular structures. high-resolution imaging. Fluorescence Microscopy Lables specific cellular components Visualising proteins and organelles. with fluorescent dyes. Cell Fractionation Separates cell components based on Studying organelle function. size and density Flow Cytometry Analyses cells in a fluid stream based Sorting and characterising cells. on their property. Immunohistochemistry Uses antibodies to detect specific Localising proteins in cells. proteins in tissues Section Four: Modelling the Fluid Mosaic Model of the Cell Membrane The cell membrane is composed of a phospholipid bilayer embedded with proteins. This fluid mosaic model describes the dynamic nature of the membrane. ❖ Phospholipid Bilayer: Consists of hydrophilic (water-attracting) heads and hydrophobic (water-repelling) tails. It forms a flexible barrier around the cell. ❖ Proteins: The cell is made up of two different types, or “classes”, of proteins. Integral proteins are nestled into the phospholipid bilayer and stick out on either end. Integral proteins are helpful for transporting larger molecules, like glucose, across the cell membrane. They have regions, called “polar” and “nonpolar” regions, that correspond with the polarity of the phospholipid bilayer. ❖ Cholesterol: Cholesterol is a type of steroid which is helpful in regulating molecules entering and exiting the cell. We’ll talk about this in more depth later, but for now remember it’s part of the cell membrane. ❖ Phospholipids: There are two important parts of a phospholipid: the head and the two tails. The head is a phosphate molecule that is attracted to water (hydrophilic). The two tails are made up of fatty acids (chains of carbon atoms) that aren’t compatible with, or repel, water (hydrophobic). When cellular membranes form, phospholipids assemble into two layers because of these hydrophilic and hydrophobic properties. The phosphate heads in each layer face the aqueous or watery environment on either side, and the tails hide away from the water between the layers of heads, because they are hydrophobic. There are 3 main factors that influence cell membrane fluidity: I. Temperature: The temperature will affect how the phospholipids move and how close together they are found. When it’s cold they are found closer together and when it’s hot they move farther apart. II. Cholesterol: The cholesterol molecules are randomly distributed across the phospholipid bilayer, helping the bilayer stay fluid in different environmental conditions. The cholesterol holds the phospholipids together so that they don’t separate too far, letting unwanted substances in, or compact too tightly, restricting movement across the membrane. Without cholesterol, the phospholipids in your cells will start to get closer together when exposed to cold, making it more difficult for small molecules, like gases to squeeze in between the phospholipids like they normally do. Without cholesterol, the phospholipids start to separate from each other, leaving large gaps. III. Saturated and unsaturated fatty acids: Fatty acids are what make up the phospholipid tails. Saturated fatty acids are chains of carbon atoms that have only single bonds between them. As a result, the chains are straight and easy to pack tightly. Unsaturated fats are chains of carbon atoms that have double bonds between some of the carbons. The double bonds create kinks in the chains, making it harder for the chains to pack tightly. What can go through the cell membrane? Phospholipids are attracted to each other, but they are also constantly in motion and bounce around a little off of each other. The spaces created by the membrane’s fluidity are incredibly small, so it is still an effective barrier. For this reason, and the ability of proteins to help with transport across the membrane, cell membranes are called semi-permeable. There are 5 broad categories of molecules found in the cellular environment. Some of these molecules can cross the membrane and some of them need the help of other molecules or processes. One way of distinguishing between these categories of molecules is based on how they react with water. Molecules that are hydrophilic (water loving) are capable of forming bonds with water and other hydrophilic molecules. They are called polar molecules. The opposite can be said for molecules that are hydrophobic (water fearing), they are called nonpolar molecules. Here are the 5 types: ❖ Small, nonpolar molecules (e.g. oxygen and carbon dioxide): These molecules can pass through the lipid bilayer and do so by squeezing through the phospholipid bilayers. They don't need proteins for transport and can diffuse across quickly. ❖ Small, polar molecules (e.g. water): These molecules can also pass through the lipid bilayer without the help of proteins, but they do so with a little more difficulty than the molecule type above. Recall that the interior of the phospholipid bilayer is made up of the hydrophobic tails. So, it's not easy for water molecules to cross, and it is a somewhat slower process. ❖ Large, nonpolar molecules (e.g. carbon rings): These rings can pass through but it is also a slow process. ❖ Large, polar molecules (e.g. simple sugar - glucose): The size and charge of large polar molecules make it too difficult to pass through the nonpolar region of the phospholipid membrane without help from transport proteins. ❖ Ions (e.g. Na+): Similarly, the charge of an ion makes it too difficult to pass through the nonpolar region of the phospholipid membrane without help from transport proteins. How do cells coordinate activities within their internal environment and the external environment? ❖ investigate the way in which materials can move into and out of cells, including but not limited to: conducting a practical investigation modelling diffusion and osmosis (ACSBL046) examining the roles of active transport, endocytosis and exocytosis (ACSBL046) relating the exchange of materials across membranes to the surface-area-to-volume ratio, concentration gradients and characteristics of the materials being exchanged (ACSBL047) ❖ investigate cell requirements, including but not limited to: suitable forms of energy, including light energy and chemical energy in complex molecules matter, including gases, simple nutrients and ions removal of wastes ❖ investigate the biochemical processes of photosynthesis, cell respiration and the removal of cellular products and wastes in eukaryotic cells (ACSBL049, ACSBL050, ACSBL052, ACSBL053) ❖ conduct a practical investigation to model the action of enzymes in cells (ACSBL050) ❖ investigate the effects of the environment on enzyme activity through the collection of primary or secondary data Section One: Cellular Transport Mechanisms Understanding how materials move into and out of cells is crucial in the field of biology. This process involves various mechanisms such as diffusion, osmosis, active transport, endocytosis, and exocytosis. Additionally, the exchange of materials across cell membranes is influenced by factors like the surface area-to-volume ratio, concentration gradients, and the characteristics of the materials involved. Diffusion and Osmosis ❖ Diffusion is the passive movement of molecules from an area of higher concentration to an area of lower concentration. It is a critical process for cellular nutrient uptake and waste removal. ❖ Osmosis is a specific type of diffusion involving water molecules. It occurs when water moves across a selectively permeable membrane to equalize solute concentrations on both sides. Osmosis is vital for maintaining cell turgidity and preventing cell lysis or plasmolysis. Active Transport ❖ Active transport is the movement of molecules against their concentration gradient using energy, typically provided by ATP. This process is crucial for importing essential nutrients and expelling waste products. Example: The sodium-potassium pump in animal cells actively transports sodium out and potassium into the cell, maintaining the cell's electrochemical gradient. Endocytosis and Exocytosis ❖ Endocytosis is the process of engulfing large particles or fluids by the cell membrane, forming vesicles. It includes phagocytosis (solid particles) and pinocytosis (liquids). ❖ Exocytosis is the opposite process, where vesicles fuse with the cell membrane, releasing their contents outside the cell. Example: Neurons use exocytosis to release neurotransmitters into synapses for cell communication. Factors Influencing Matrial Exchange Several factors affect material exchange across cell membranes: ❖ Surface Area-to-Volume Ratio: Smaller cells with a high surface area-to-volume ratio facilitate faster exchange of materials. ❖ Concentration Gradients: The steeper the concentration gradient, the faster the diffusion rate. Cells often maintain concentration gradients for essential molecules. ❖ Characteristics of Materials: Size, charge, and solubility of materials influence their ability to pass through membranes. Section Two: Cellular Requirements and Processes Cell Energy Requirements ❖ Cells require suitable forms of energy for their functions: Light Energy → Photosynthetic cells use light energy to convert carbon dioxide and water into glucose and oxygen. Chemical Energy → Cells store and utilise energy in the form of ATP (adenosine triphosphate), generated during processes like cellular respiration. Matter Requirements ❖ Cells need various forms of matter: Gases → Oxygen for respiration and carbon dioxide for photosynthesis Simple Nutrients → Glucose, amino acids, and fatty acids for growth, maintenance, and energy Ions → Essential ions like sodium, potassium, calcium, and chlorine for cellular processes. Removal of Wastes ❖ Cells produce waste products that need removal: Carbon Dioxide → Generated during respiration, must be expelled to maintain proper pH. Toxic Metabolites → Byproducts of metabolism, like urea in mammals, require excretion. Biochemical Processes ❖ Photosynthesis Photosynthesis is the process by which autotrophic cells convert light energy into chemical energy (glucose). The equation for this is: 6CO2 + 6H2O + lightenergy → C6H12O6 + 6O2 ❖ Cell Respiration Cell respiration is the process by which cells break down glucose to produce ATP. It occurs in three stages: glycolysis, the citric acid cycle and oxidative phosphorylation. C6H12O6 + 6O2 → 6CO2 + 6H2O + ATP ❖ Enzyme Activity Enzymes are biological catalysts that speed up chemical reactions. The rate of enzyme activity is influenced by factors such as temperature, pH, substrate concentration, and enzyme concentration. To model enzyme activity, you can conduct experiments measuring the affect of these tactors on enzyme reaction rates. ❖ Environment and Enzyme Activity The environment significantly affects enzyme activity. Extreme pH levels or temperatures can denature enzymes, rendering them ineffective. Collecting primary or secondary data through experiments can reveal the optimal conditions for enzyme function. Module Two: Organisation of Living Things How are cells arranged in a multicellular organism? ❖ compare the differences between unicellular, colonial and multicellular organisms by: investigating structures at the level of the cell and organelle relating structure of cells and cell specialisation to function ❖ investigate the structure and function of tissues, organs and systems and relate those functions to cell differentiation and specialisation ❖ justify the hierarchical structural organisation of organelles, cells, tissues, organs, systems and organisms Section One: Unicellular Organisms Structures at the Cellular Level ❖ Unicellular organisms are composed of a single cell that performs all essential functions for life. This cell contains organelles, which are membrane bound structures with specific functions. ❖ An example of this is bacteria: Cell Membrane: Regulates the passage of molecules in and out of the cell. Cytoplasm: Contains enzymes and molecules necessary for metabolism. Ribosomes: Synthesise proteins. Nucleoid (DNA region): Contains genetic material. Flagella or Cilia: Aid in locomotion (if present). Cell Specialisation and Function In unicellular organisms, all cellular structures are specialized to perform various functions necessary for survival. For example, the cell membrane helps regulate nutrient uptake and waste removal, while ribosomes synthesize proteins essential for cell growth and function. Section Two: Colonial Organisms Structures at the Cellular Level ❖ Colonial organisms consist of multiple similar cells that live together but do not have a specialized division of labor among cells. Each cell within a colony is similar in structure and function. ❖ An example of this is Volvox: Somatic Cells: Responsible for locomotion and protection. Reproductive Cells: Produce new colonies. Cell Specialisation and Function While cells within a colony may be similar in structure, they can exhibit limited specialization. For instance, somatic cells in Volvox colonies specialize in locomotion and protection, whereas reproductive cells focus on reproduction. Section Three: Multicellular Organisms Structures at the Cellular Level ❖ Multicellular organisms are composed of numerous specialized cells organized into tissues, organs, and organ systems. ❖ An example of this are Humans: Muscle Cells: Contract for movement. Neurons: Transmit electrical signals. Red Blood Cells: Transport oxygen. Liver Cells: Metabolize nutrients and detoxify the body. Cell Specialisation and Function ❖ In multicellular organisms, cells are highly specialized, and their functions are closely related to their structures. For example, red blood cells are biconcave in shape to increase surface area for oxygen transport, while muscle cells contain numerous contractile proteins for movement. Section Four: Tissues, Organs, and Systems Tissues Tissues are groups of similar specialised cells that work together to perform a specific function. Examples include muscle tissue, nervous tissue, and epithelial tissue. Organs Organs are structures composed of different tissues organised to carry out specific functions. For instance, the heart is an organ composed of muscle, nervous, and connective tissues. Systems Organ systems are groups of organs that work together to perform complex functions. In humans, the circulatory system comprises the heart, blood vessels, and blood, working collectively to transport nutrients and oxygen. Section Five: Heirarchial Structural Organisation The hierarchical structure organisation in biology is justified by the need for efficiency and specialisation. ❖ Organelles are specialised for specific cellular functions ❖ Cells are specialised to perform particular roles within a multicellular organism ❖ Tissues allow for more efficient execution of functions compared to individual cells ❖ Organs combine various tissues to perform complex tasks ❖ Organ systems coordinate multiple organs to achieve specific functions ❖ Organisms have specialised organ systems, optimising survival and reproduction What is the difference in nutrient and gas requirements between autotrophs and heterotrophs? ❖ investigate the structure of autotrophs through the examination of a variety of materials, for example: dissected plant materials microscopic structures using a range of imaging technologies to determine plant structure ❖ investigate the function of structures in a plant, including but not limited to: tracing the development and movement of the products of photosynthesis ❖ investigate the gas exchange structures in animals and plants through the collection of primary and secondary data and information, for example: microscopic structures: alveoli in mammals and leaf structure in plants macroscopic structures: respiratory systems in a range of animals ❖ interpret a range of secondary-sourced information to evaluate processes, claims and conclusions that have led scientists to develop hypotheses, theories and models about the structure and function of plants, including but not limited to: photosynthesis transpiration-cohesion-tension theory ❖ trace the digestion of foods in a mammalian digestive system, including: physical digestion chemical digestion absorption of nutrients, minerals and water elimination of solid waste ❖ compare the nutrient and gas requirements of autotrophs and heterotrophs Section One: Investigating Autotroph Structure Autotrophs are organisms capable of producing their own food through processes like photosynthesis. To understand their structure, we can examine various materials, including dissected plant materials and microscopic structures, using imaging technologies. Dissected Plant Materials: ❖ Plant Roots → Roots anchor the plant and absorb water and nutrients. They may have specialised structures like root hairs for increased surface area. ❖ Stems → Stems provide structural support and transport materials (eg. water and nutrients). They may have vascular tissues (xylem and phloem) ❖ Leaves → Leaves are the primary site of photosynthesis. They have specialised structures called chloroplasts containing chlorophyll. Microscopic Structures: ❖ Chloroplasts → These organelles contain chlorophyll and are responsible for photosynthesis. ❖ Stomata → Tiny openings on the underside of leaves that regulate gas exchange. ❖ Vascular Tissues → Xylem transports water, while phloem transports nutrients. Section Two: Investigating Plant Function Understanding the function of plant structures involves tracing the development and movement of products of photosynthesis. ❖ Photosynthesis → This process converts light energy into chemical energy (glucose) using carbon dioxide and water. Oxygen is released as a byproduct. ❖ Transport → Xylem transports water and minerals from the roots to the leaves, while phloem carries sugars produced in photosynthesis to other parts of the plant. Section Three: Investigating Gas Exchange Structures Gas exchange structures are vital for respiration in both animals and plants. Examining these structures involves microscopic and macroscopic observations. ❖ Microscopic Structures: Alveoli in Mammals: Alveoli are tiny air sacs in the lungs where gas exchange occurs. Oxygen enters the bloodstream, and carbon dioxide exits. Leaf Structure in Plants: Stomata and the mesophyll layer in leaves facilitate gas exchange. Oxygen is released, and carbon dioxide is absorbed. ❖ Macroscopic Structures: Respiratory Systems: In animals, various structures aid in gas exchange, such as gills in fish, tracheal systems in insects, and lungs in mammals. Section Four: Interpreting Secondary-Sourced Information To understand the structure and function of plants, we must evaluatie scientific hypotheses, theories, and models such as: ❖ Photosynthesis → The process by which plants convert light energy into chemical energy, leading to the development of the chloroplast model. ❖ Transpiration-Cohension-Tension Theory → Explains how water is transported from roots to leaves through the xylem. Section Five: Digestion in Mammalian Divestive System Digestion in mammals is a complex process involving physical and chemical digestion, absorption of nutrients, minerals, water, and the elimination of solid waste. Physical Digestion: ❖ Mouth → Chewing mechanically breaks down food into smaller particles. ❖ Stomach → The stomach churns and mixes food with gastric juices, creating a semi-liquid mixture. ❖ Small Intestine → Here, food is mixed with digestive enzymes and bile for further breakdown and absorption. Chemical Digestion: ❖ Enzymatic Action → Enzymes like amylase (breaks down carbohydrates), lipase (breaks down fats), and protease (breaks down proteins) play key roles. ❖ Acidic Environment → The stomach's acidity aids in protein digestion. Absorption: ❖ Small Intestine → Nutrients are absorbed into the bloodstream through the small intestine's walls. ❖ Large Intestine → Water and some minerals are absorbed in the colon. Elimination of Solid Waste: ❖ Large Intestine → Undigested materials, water, and minerals are formed into feces, which are elimitated through the rectum and anus. Section Six: Comparing Autotrophs and Heterotrophs Autotrophs and heterotrophs have different nutrient and gas requirements. Nutrient Requirements Gas Requirements Autotrophs They produce their own food They require carbon dioxide for through photosynthesis and photosynthesis and release oxygen as primarily require water, carbon a byproduct. dioxide, and minerals. Heterotrophs They cannot produce their own food They require oxygen for cellular and require complex organic respiration and release carbon molecules, including carbohydrates, dioxide as a waste product. proteins, and fats, obtained through consumption. How does the composition of the transport medium change as it moves around an organism? ❖ investigate transport systems in animals and plants by comparing structures and components using physical and digital models, including but not limited to: macroscopic structures in plants and animals microscopic samples of blood, the cardiovascular system and plant vascular systems ❖ investigate the exchange of gases between the internal and external environments of plantsand animals ❖ compare the structures and function of transport systems in animals and plants, including but not limited to: vascular systems in plants and animals open and closed transport systems in animals ❖ compare the changes in the composition of the transport medium as it moves around an organism Transport systems play a crucial role in both animals and plants, facilitating the exchange of essential substances and maintaining homeostasis. In this study, we will explore the structures, components, and functions of these transport systems, comparing them across macroscopic and microscopic levels, and highlighting the exchange of gases and changes in the composition of transport media. Section One: Macroscopic Structures in Plants and Animals Plant Transport System ❖ Xylem: Xylem is a plant tissue responsible for the transport of water and minerals from the roots to the aerial parts of the plant. It is composed of tracheids and vessel elements, providing mechanical support. ❖ Phloem: Phloem transports the products of photosynthesis, primarily sugars (sucrose), from the leaves to other plant parts. It consists of sieve tube elements and companion cells. Animal Transport System ❖ Cardiovascular System: In animals, the cardiovascular system is responsible for the transport of blood throughout the body. It comprises the heart, blood vessels (arteries, veins, and capillaries), and blood itself. Section Two: Microscopic Samples Blood and Cardiovascular System ❖ Blood: Blood is composed of red blood cells (erythrocytes), white blood cells (leukocytes), platelets (thrombocytes), and plasma. It transports oxygen, nutrients, hormones, and waste products. ❖ Vascular System: Arteries carry oxygenated blood away from the heart, while veins transport deoxygenated blood back to the heart. Capillaries facilitate the exchange of substances between blood and tissues. Plant Vascular System ❖ Tracheids and Vessel Elements: These are the two types of cells in xylem. Tracheids are long, tapered cells with small openings called pits. Vessel elements are shorter and wider, forming continuous tubes. ❖ Sieve Tube Elements and Companion Cells: Sieve tube elements are specialized for sugar transport. Companion cells support sieve tube function by providing energy and metabolic support. Section Three: Exchange of Gases Plants ❖ Photosynthesis: In plants, carbon dioxide (CO2) is taken in from the atmosphere through small openings called stomata, while oxygen (O2) is released as a byproduct. This process occurs primarily in the leaves. ❖ Respiration: Plants also undergo cellular respiration, consuming oxygen and producing carbon dioxide, especially in the absence of light. Animals ❖ Respiration: Animals take in oxygen through their respiratory systems (lungs, gills, or tracheal systems) and release carbon dioxide as a waste product. The circulatory system transports gases between tissues and the respiratory organs. Section Four: Structures and Functions of Transport Systems Vascular Systems in Plants and Animals: Feature Plant Vascular System Animal Cardiovascular System Transport Medium Water and minerals (xylem), sugars Blood (phloem) Pumping organ None (passive flow) Heart Transport Direction Upward (xylem), Bidirectional Bixirectional (phloem) Role in Transport Water and mineral uptake, Nutrient Oxygen and nutrient delivery, Waste distrubution removal Open and Closed Transport Systems in Animals ❖ Open Circulatory System: Found in some invertebrates (e.g., arthropods). Hemolymph, a fluid, bathes the internal organs directly. Limited control over where substances go. ❖ Closed Circulatory System: Found in most vertebrates (including humans) and some invertebrates (e.g., cephalopods). Blood flows within vessels (arteries, veins, capillaries). Precise control over the distribution of substances. Section Five: Changes in the Composition of Transport Media Blood Composition Changes: Component Changes in Blood Oxygen Levels Oxygen-rich (in arteries), Oxygen-poor (in veins) Nutrient Levels Nutrient-rich (after meals), Nutrient-poor (between meals) Waste Product Accumulation Accumulation of metabolic waste products (eg. urea) Hormone Levels Fluctuations in hormone concentrations Plant Transport Medium: The composition of the plant's transport medium (sap) remains relatively stable, with variations in nutrient and hormone concentrations depending on growth stages and environmental conditions. Module Three: Biological Diversity How do environmental pressures promote a change in species diversity and abundance? ❖ predict the effects of selection pressures on organisms in ecosystems, including: biotic factors abiotic factors ❖ investigate changes in a population of organisms due to selection pressures over time, for example: cane toads in Australia prickly pear distribution in Australia Selection pressures play a pivotal role in shaping the characteristics and adaptations of organisms within ecosystems. These pressures can be categorised into biotic and abiotic factors, both of which influence the survival and reproduction of species. To understand the effects of selection pressures, we will investigate how they impact populations over time, using the examples of cane toads and prickly pear distribution in Australia. Section One: Selection Pressures in Ecosystems Biotic Factors ❖ Biotic factors encompass all living organisms within an ecosystem. They can exert various selection pressures on each other. Common examples include: Predation: Predators exert pressure on prey populations, favoring individuals with traits that enhance survival, such as camouflage or defensive mechanisms. Competition: Intraspecific (within species) and interspecific (between species) competition for resources like food, territory, and mates can drive adaptations for efficient resource utilisation. Symbiosis: Mutualistic relationships, such as pollination or nitrogen-fixing bacteria in plant roots, can lead to coevolution as organisms adapt to maximize the benefits of the relationship Abiotic Factors ❖ Abiotic factors are non-living components of an ecosystem. They can also impose significant selection pressures: Climate: Temperature, precipitation, and other climatic factors impact an organism's ability to survive in a given environment. Species adapt to temperature extremes or seasonal variations. Geography and Terrain: The physical characteristics of the environment, like altitude, topography, and soil composition, can influence where species can thrive. Resource Availability: Abiotic factors such as nutrient availability and water quality affect an organism's ability to grow and reproduce. Section Two: Changes in Populations due to Selection Pressures Cane Toads in Australia Cane toads (Rhinella marina) were introduced to Australia in the 1930s to control cane beetles. However, they became an invasive species due to limited natural predators and have had profound ecological effects. ❖ Selection Pressures on Cane Toads Predation: Initially, Australian predators were unaccustomed to the cane toads' toxic skin secretions, resulting in high predation avoidance. Over time, some predators developed adaptations, such as altered behavior or resistance, to consume cane toads. Competition: Cane toads outcompeted native amphibians for resources, affecting their populations. Disease: The introduction of cane toads also brought diseases that affected native species. ❖ Effects on Cane Toad Populations Cane toad populations initially increased due to the absence of effective predators. Over time, some native species developed resistance or behavioral adaptations to reduce predation. Competition with native species and disease transmission to local fauna had negative impacts on the ecosystem. Prickly Pear Distribution in Australia Prickly pear (Opuntia spp.) was introduced to Australia in the 19th century. Its unchecked growth became an ecological problem, leading to a famous biological control program. ❖ Selection Pressures on Prickly Pear Competition: Prickly pear competed with native vegetation for space and resources, altering the ecosystem. Herbivory: The introduction of the cactoblastis moth as a biological control agent exerted pressure on prickly pear populations. ❖ Effects on Prickly Pear Distribution Prickly pear spread rapidly, impacting vast areas of land. Introduction of the cactoblastis moth controlled the prickly pear population, resulting in its decline. How do adaptations increase the organism’s ability to survive? ❖ conduct practical investigations, individually or in teams, or use secondary sources to examine the adaptations of organisms that increase their ability to survive in their environment, including: structural adaptations physiological adaptations behavioural adaptations ❖ investigate, through secondary sources, the observations and collection of data that were obtained by Charles Darwin to support the Theory of Evolution by Natural Selection, for example: finches of the Galapagos Islands Australian flora and fauna Adaptations are key evolutionary mechanisms that enhance an organism's ability to survive and reproduce in its environment. These adaptations can be structural, physiological, or behavioral, and they often result from the process of natural selection. Charles Darwin's observations, including those of the finches of the Galapagos Islands and Australian flora and fauna, played a pivotal role in supporting the Theory of Evolution by Natural Selection. In this study, we will explore these concepts in depth. Section One: Adaptations in Organisms Structural Adaptations ❖ Structural adaptations involve physical features that help an organism survive in its environment. These adaptations can be observed in various forms, such as the shape of body parts, coloration, and specialized organs. Example Adaptation Function Giraffe’s Long Neck Long neck and legs Allows them to reach high foliage for food Camouflage in Chameleons Colour-changing Skin Conceals them from predators and prey Cacti’s Water Storage Thick, fleshy stems Stores water to survive in arid environments Psysiological Adaptations ❖ Physiological adaptations involve internal mechanisms that optimize an organism's functioning in its environment. These adaptations often relate to processes like metabolism, temperature regulation, and chemical defenses. Example Adaptation Function Hibernation in Bears Slowed metabolism Conserves energy during winter months when food is scarce. Anti-freeze Proteins in Anti-freeze proteins in Prevents ice formation inside cells in Arctic Fish body fluids extremely cold environments. Desert Plants' Water Crassulacean Acid Allows them to open stomata at Efficiency Metabolism (CAM) night and minimize water loss during the day. Behavioral Adaptations ❖ Behavioral adaptations are actions or strategies that organisms exhibit to improve their chances of survival and reproduction. These behaviors can include mating rituals, communication, and foraging techniques. Example Adaptation Function Bird Migration Seasonal long-distance Helps birds find food and nesting movement sites in different regions throughout the year. Social Cooperation in Ant Division of labor Efficiently forage for food, care for Colonies young, and defend the colony. Defensive Behavior in Resembling harmful Deters predators by appearing Mimicry species dangerous or unpalatable. Section Two: Charles Darwin and the Theory of Evolution by Natural Selection Darwin’s Observations ❖ Charles Darwin made groundbreaking observations during his voyage on the HMS Beagle, particularly in the Galapagos Islands and Australia, which provided evidence for his Theory of Evolution by Natural Selection. ❖ Galapagos Islands → Darwin observed a variety of finches on the Galapagos Islands, each with unique beak shapes suited to their specific food sources. This diversity suggested adaptation through natural selection. Example: The Galapagos finches' beaks demonstrated how variation within a population could lead to adaptation to different ecological niches. Finches with beaks suitable for cracking seeds would thrive where seeds were abundant. ❖ Australian Flora and Fauna → In Australia, Darwin noted marsupials and monotremes, unique groups of mammals not found in other parts of the world. This distribution suggested that organisms evolved in response to their local environments. Example: The platypus and kangaroo are iconic Australian species, adapted to their ecosystems through distinct physiological and behavioral traits. Key Concepts of Natural Selection ❖ Darwin's theory of natural selection rests on several key concepts: Variation: Within populations, individuals exhibit variation in traits. Overproduction: More offspring are produced than can survive. Struggle for Existence: Limited resources result in competition for survival. Survival of the Fittest: Those with advantageous traits are more likely to survive and reproduce. Adaptation: Over time, the population becomes better adapted to its environment. What is the relationship between evolution and biodiversity? ❖ explain biological diversity in terms of the Theory of Evolution by Natural Selection by examining the changes in and diversification of life since it first appeared on the Earth ❖ explain, using examples, how Darwin and Wallace’s Theory of Evolution by Natural Selection accounts for: convergent evolution divergent evolution The Theory of Evolution by Natural Selection, developed by Charles Darwin and independently by Alfred Russel Wallace, is one of the most significant concepts in biology. This theory explains how biological diversity has arisen over time through changes and diversification of life forms on Earth. In this study, we will delve into the theory and examine how it accounts for convergent and divergent evolution. Section One: Theory of Evolution by Natural Selection Key Concepts ❖ Descent with Modification: Species evolve from pre-existing species, with modifications accumulating over generations. ❖ Natural Selection: The environment selects individuals with advantageous traits, leading to the survival and reproduction of those better adapted to their environment. ❖ Variation: Individuals within a population exhibit variation in traits, which is partly heritable. ❖ Overproduction: Populations produce more offspring than the environment can support, leading to competition for resources. ❖ Adaptation: Over time, favorable traits become more common in a population, leading to adaptations to the environment. Changes in and Diversification of Life ❖ The Theory of Evolution explains how life has diversified and changed since its appearance on Earth, leading to the wide variety of species we see today. This diversification occurs through the following mechanisms: Speciation: New species arise when populations become isolated and accumulate genetic differences over time. For example, the Galápagos finches, studied by Darwin, diverged into multiple species due to different food sources on different islands. Adaptive Radiation: When a single ancestral species gives rise to multiple new species, each adapted to different ecological niches. The classic example is the Darwin's finches. Extinction: Species that are less adapted to their environment may become extinct, leaving room for other species to evolve and fill ecological niches. Continual Adaptation: As environments change, species must continually adapt or face extinction. This is evident in the fossil record, where species adapted to different climates have existed over geological time scales. Section Two: Convergent Evolution ❖ Convergent evolution occurs when unrelated species independently evolve similar traits or adaptations due to similar environmental pressures. This phenomenon is a powerful demonstration of the adaptability of life. Examples include: Wings in Birds and Bats: Birds and bats are unrelated but both evolved wings for flight. Echolocation: Dolphins and bats developed echolocation for navigating and hunting, despite their distant ancestry. Camouflage: Various species from different lineages have evolved similar camouflage patterns to blend into their surroundings. ❖ Convergent evolution illustrates the role of natural selection in shaping organisms to fit specific ecological roles, even when they have different genetic origins. Section Three: Divergent Evolution ❖ Divergent evolution is the opposite of convergent evolution. It occurs when two or more species with a common ancestor evolve different traits or adaptations. This divergence often happens when populations become isolated from one another and face different selective pressures. Examples include: Darwin's Finches: As mentioned earlier, the finches on the Galápagos Islands diverged into multiple species with different beak shapes and diets. Homologous Structures: Similar structures in different species, such as the forelimbs of vertebrates, originated from a common ancestor but have diverged in function due to different selective pressures. Adaptive Radiation: When a single species diversifies into several species to occupy different ecological niches, this is a clear example of divergent evolution. ❖ Divergent evolution highlights the idea that diversity arises from ancestral populations adapting to different environments over time. What is the evidence that supports the Theory of Evolution by Natural Selection? ❖ investigate, using secondary sources, evidence in support of Darwin and Wallace’s Theory of Evolution by Natural Selection, including but not limited to: biochemical evidence, comparative anatomy, comparative embryology and biogeography techniques used to date fossils and the evidence produced ❖ explain modern-day examples that demonstrate evolutionary change, for example: the cane toad antibiotic-resistant strains of bacteria Charles Darwin and Alfred Russel Wallace's Theory of Evolution by Natural Selection revolutionized our understanding of how species evolve over time. This theory proposes that species change through natural selection, with individuals possessing advantageous traits more likely to survive and reproduce. Over time, these traits become more prevalent in the population. This study explores evidence supporting this theory, including biochemical evidence, comparative anatomy, comparative embryology, biogeography, dating fossils, and modern-day examples of evolutionary change. Section One: Evidence in Support of Evolution by Natural Selection I. Biochemical Evidence ❖ Biochemical evidence, particularly molecular biology, has provided compelling support for evolution: DNA Sequencing: Comparing the DNA of different species reveals similarities and differences. The degree of genetic relatedness mirrors the degree of evolutionary relatedness. For example, humans share a high percentage of DNA with chimpanzees. Protein Homology: Similarities in protein structures and functions among species suggest common ancestry. Hemoglobin, a protein responsible for oxygen transport, exhibits homology across species. II. Comparative Anatomy ❖ Comparative anatomy examines structural similarities and differences among species: Homologous Structures: These are anatomical structures that have a common origin but may serve different functions. For instance, the pentadactyl limb (five-fingered limb) is found in many vertebrates, including humans, whales, and bats, despite their varied functions. Vestigial Structures: These are remnants of ancestral structures that no longer serve a purpose in modern organisms. Examples include the human appendix and the wings of flightless birds. III. Comparative Embryology ❖ Comparative embryology studies the development of organisms: Embryological Homologies: Embryos of different species often resemble each other during early stages of development. For example, the embryos of vertebrates have gill slits and tails at certain stages, reflecting shared ancestry. IV. Biogeography ❖ Biogeography examines the distribution of species around the world: Island Species: Islands often have unique species that evolved from mainland ancestors. For example, Darwin's finches in the Galápagos Islands exhibit diverse beak shapes, adapted to various food sources. Continental Drift: The distribution of fossils and living species on continents supports the idea of plate tectonics and species evolution. For instance, similar fossils of marsupials are found in South America and Australia, suggesting a common ancestor when these continents were connected. Section Two: Techniques Used to Date Fossils and the Evidence Produced I. Radiometric Dating ❖ Radiometric dating measures the decay of radioactive isotopes to determine the age of fossils. Common methods include: Carbon-14 Dating: Useful for dating relatively recent fossils (up to around 50,000 years old). Potassium-Argon Dating: Suitable for older fossils, such as those in volcanic rock layers. Uranium-Series Dating: Used for dating carbonate deposits in caves. II. Stratigraphy ❖ Stratigraphy involves analyzing the layering of rocks and fossils within them. The principle of superposition states that younger rocks and fossils are found above older ones. III. Fossil Record ❖ The fossil record itself is evidence of evolution. Fossils of transitional forms, like Tiktaalik (a fish-tetrapod transition), provide crucial evidence for species' gradual changes over time. Section Three: Modern-Day Examples of Evolutionary Change I. The Cane Toad (Bufo marinus) ❖ Introduced to control pests in Australia, cane toads have exhibited evolutionary changes: Increased Leg Length: Populations of cane toads in Australia have evolved longer legs, potentially aiding their spread. Resistance to Toxins: Toads exposed to toxic cane toad eggs developed resistance, illustrating natural selection in action. II. Antibiotic-Resistant Bacteria ❖ Bacteria can evolve resistance to antibiotics due to: Selective Pressure: Antibiotics kill susceptible bacteria, but resistant strains survive and reproduce. Horizontal Gene Transfer: Bacteria can acquire resistance genes from other bacteria through plasmids. Module Four: Ecosystem Dynamics What effect can one species have on the other species in a community? ❖ investigate and determine relationships between biotic and abiotic factors in an ecosystem, including: the impact of abiotic factors the impact of biotic factors, including predation, competition and symbiotic relationships the ecological niches occupied by species predicting consequences for populations in ecosystems due to predation, competition, symbiosis and disease measuring populations of organisms using sampling techniques ❖ explain a recent extinction event Ecosystems are dynamic and complex environments where both biotic (living) and abiotic (non-living) factors interact to shape the populations and dynamics of species within them. Understanding these interactions is essential for comprehending the functioning and stability of ecosystems. In this study, we will investigate the relationships between biotic and abiotic factors, examine their impacts, explore ecological niches, predict consequences of various interactions, and delve into population measurement techniques. Additionally, we will explain a recent extinction event to highlight the real-world implications of these ecological concepts. Section One: Abiotic and Biotic Factors Abiotic Factors Abiotic factors include non-living elements like temperature, humidity, soil composition, sunlight, and precipitation. These factors play a significant role in shaping ecosystems by influencing the distribution and behavior of organisms. For example, the availability of sunlight affects photosynthesis in plants, which, in turn, impacts herbivores and predators. ❖ Impact of Abiotic Factors: Temperature: Temperature affects the metabolic rates of organisms, influencing their growth and reproduction. Precipitation: The amount and distribution of rainfall determine the types of plants and animals that can thrive in an area. Biotic Factors Biotic factors involve living organisms within an ecosystem. They include interactions such as predation, competition, and symbiosis. ❖ Impact of Biotic Factors: Predation: Predators feed on prey, controlling prey populations and shaping their behavior, morphology, and life history strategies. Competition: Organisms compete for limited resources like food, territory, and mates. This competition can lead to resource partitioning and niche differentiation. Symbiotic Relationships: Symbiotic interactions include mutualism (both species benefit), commensalism (one benefits, and the other is neither harmed nor helped), and parasitism (one benefits at the expense of the other). Section Two: Ecological Niches ❖ Ecological niches describe the role and position of a species within an ecosystem. It encompasses its habitat, food sources, behavior, and interactions with other species. Species occupying the same niche may compete for resources, leading to niche differentiation to reduce competition. Example: Darwin's finches in the Galápagos Islands exhibit niche differentiation in beak size and shape to exploit different food sources, reducing competition. Section Three: Predicting Consequenses ❖ Predation Predation can lead to population control, prey adaptations (e.g., camouflage or warning coloration), and the evolution of predator-prey relationships. ❖ Competition Competition can result in resource partitioning, where species evolve to use different resources or occupy different niches. It can also lead to competitive exclusion, where one species outcompetes and drives another to extinction. ❖ Symbiosis Symbiotic relationships can have diverse consequences. For example, mutualism can enhance the fitness of both species, while parasitism can harm one species without necessarily leading to extinction. ❖ Disease Disease outbreaks can decimate populations. For example, the chytrid fungus has contributed to the decline and extinction of many amphibian species. Section Four: Population Measurement Techniques Sampling Techniques ❖ Sampling methods like quadrat sampling and transect sampling help estimate population sizes. These methods involve sampling a portion of the habitat to extrapolate to the entire population. Example of Quadrat Sampling: I. Define the study area. II. Divide the area into a grid of equal-sized quadrats. III. Randomly select quadrats to survey. IV. Count the number of individuals or species within each quadrat. V. Extrapolate the data to estimate the entire population size. Section Five: Recent Extinction → The Northern White Rhino ❖ The Northern White Rhino (Ceratotherium simum cottoni) is a tragic example of a recent extinction event. Driven by factors such as habitat loss and poaching for their horns, their population declined rapidly. By 2008, only eight individuals remained. Despite conservation efforts, including attempts at assisted reproduction, the last male Northern White Rhino, Sudan, died in 2018. Today, the subspecies is functionally extinct, serving as a stark reminder of the devastating impact of human activities on Earth's biodiversity. How do selection pressures within an ecosystem influence evolutionary change? ❖ investigate and analyse past and present technologies that have been used to determine evidence for past changes, for example: radiometric dating Determining evidence for past changes is vital in fields like geology, archaeology, and paleontology. Radiometric dating is a powerful technique used to establish the age of rocks, fossils, and archaeological artifacts. This method relies on the principles of radioactive decay and offers crucial insights into Earth's history and the evolution of life. In this study, we will delve into radiometric dating, exploring its principles, applications, and significant contributions to understanding past changes. Section One: Principles of Radiometric Dating Radiometric dating is based on the principle of radioactive decay. Certain elements, known as isotopes, have unstable nuclei and spontaneously transform into more stable forms over time. This transformation occurs at a predictable rate, referred to as the half-life. The half-life is the time it takes for half of the radioactive isotopes in a sample to decay. By measuring the ratio of parent isotopes to daughter isotopes, scientists can determine the age of a sample. ❖ Here are some commonly used isotopes in radiometric dating: Carbon-14 (C-14): Used for dating organic materials up to about 50,000 years ago. Uranium-238 (U-238): Used for dating rocks and minerals that are millions to billions of years old. Potassium-40 (K-40): Used for dating rocks and minerals, particularly in the range of hundreds of thousands to billions of years. Section Two: Radiometric Dating Techniques Carbon Dating ❖ Carbon-14 dating is a widely known radiometric dating technique used to date organic materials, including archaeological artifacts and fossils. Carbon-14 is formed in the atmosphere when cosmic rays interact with nitrogen-14. Living organisms continuously exchange carbon with their environment, maintaining a constant ratio of carbon isotopes. When an organism dies, it stops absorbing carbon, and the C-14 in its remains begins to decay. By measuring the remaining C-14, scientists can calculate the age of the sample. Uranium-Series Dating ❖ Uranium-series dating is used for dating carbonate materials like speleothems (cave formations) and corals. It relies on the radioactive decay of uranium isotopes and their subsequent daughters, such as thorium-230 and radium-226. By measuring the ratios of these isotopes in a sample, scientists can determine its age. Potassium-Argon Dating ❖ Potassium-argon dating is commonly used for dating volcanic rocks and minerals. Potassium-40, which decays to argon-40, is used in this method. The age of the sample is calculated by measuring the ratio of argon-40 to potassium-40. Section Three: Applications of Radiometric Dating Geological Dating ❖ Radiometric dating is fundamental in determining the ages of rocks and minerals. It helps geologists understand the Earth's geological history, including the timing of volcanic eruptions, mountain formation, and the development of continents. Archaelogical Dating ❖ In archaeology, radiometric dating is used to establish the age of artifacts and ancient structures. This technique has provided insights into the timeline of human evolution and the development of ancient civilizations. Paleontological Dating ❖ Radiometric dating aids in dating fossils, helping paleontologists reconstruct the evolutionary history of life on Earth. It allows them to establish when specific species lived and how they evolved over time. Section Four: Limitations and Considerations ❖ While radiometric dating is a powerful tool, it has limitations: Contamination: Samples can be contaminated with modern or older materials, affecting dating accuracy. Sample Size: The size and quality of the sample can impact the precision of the dating method. Assumptions: Radiometric dating relies on assumptions about the initial isotopic composition, which may not always be accurate. Changing Decay Rates: Extreme conditions, such as high temperatures or pressures, can alter decay rates, potentially leading to incorrect age estimates. How can human activity impact an ecosystem? ❖ investigate changes in past ecosystems that may inform our approach to the management of future ecosystems, including: the role of human-induced selection pressures on the extinction of species models that humans can use to predict future impacts on biodiversity the role of changing climate on ecosystems ❖ investigate practices used to restore damaged ecosystems, Country or Place, for example: mining sites land degradation from agricultural practices Understanding changes in past ecosystems is essential for informing our approach to managing future ecosystems. These changes include the impact of human-induced selection pressures on species extinction, predictive models for biodiversity, the role of climate change in ecosystems, and practices for restoring damaged ecosystems. This knowledge helps us make informed decisions to conserve and restore biodiversity while addressing contemporary environmental challenges. Section One: Role of Human-Induced Selection Pressures on Species Extinction Human Induced Selection Pressures ❖ Human activities such as habitat destruction, pollution, overexploitation, and introduction of invasive species exert selection pressures on ecosystems. These pressures have led to the extinction of numerous species. Example: Passenger Pigeon The passenger pigeon, once abundant in North America, was driven to extinction in the early 20th century due to excessive hunting and habitat destruction. This demonstrates the profound impact of human-induced selection pressures on species survival. Lessons Learned Studying past extinctions highlights the need for conservation efforts, habitat protection, and sustainable resource management to prevent future species loss. Section Two: Predictive Models for Biodiversity Biodiversity Modelling ❖ Scientists use various models to predict the impact of human activities on biodiversity. These models incorporate data on species distribution, habitat loss, climate change, and population dynamics. Example: Species Distribution Models (SDMs) SDMs use environmental variables to predict the potential geographic range of species. They help identify areas at risk and prioritize conservation efforts Lessons Learned Predictive models provide valuable insights for policymakers and conservationists, enabling them to make informed decisions to mitigate biodiversity loss. Section Three: Role of Changing Climate on Ecosystems Climate Change and Ecosystems ❖ Climate change affects ecosystems by altering temperature, precipitation patterns, and sea levels. These changes impact species distribution, migration patterns, and the availability of resources. Example: Polar Bears Polar bears rely on sea ice to hunt seals. Melting sea ice due to rising temperatures threatens their survival, illustrating the direct link between climate change and ecosystem disruption. Lessons Learned Understanding the relationship between climate change and ecosystems underscores the importance of reducing greenhouse gas emissions and implementing climate adaptation strategies. Section Four: Practices to Restore Damaged Ecosystems Ecosystem Restoration ❖ Efforts to restore damaged ecosystems aim to recover biodiversity, ecosystem functions, and ecosystem services. Various practices are used, depending on the type and extent of damage. Example: Mining Site Restoration After mining operations cease, reclamation efforts involve reshaping the land, planting native vegetation, and managing water to restore the ecosystem. This process may take years or even decades. Example: Land Degradation from Agricultural Practices Conservation tillage, crop rotation, and afforestation are practices used to combat land degradation caused by agriculture. These methods help improve soil health and reduce erosion. Lessons Learned Ecosystem restoration demonstrates the potential for humans to mitigate their impact on the environment and revitalize damaged landscapes. It emphasizes the importance of sustainable land management practices.