Year 11 Biology Notes 2024-2026 PDF
Document Details
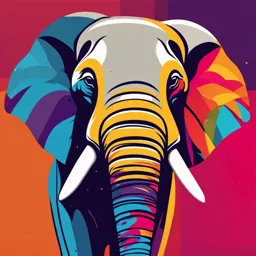
Uploaded by WiseConnotation636
Carlingford High School
2024
Madeleine Wainwright
Tags
Summary
These are Year 11 Biology course notes covering a complete syllabus from 2024-2026. The notes include details about cells, organisation of living things, biological diversity, and ecosystem dynamics.
Full Transcript
Year 11 Biology Complete Course Notes 2024–2026 Madeleine Wainwright Contents I Cells as the Basis of Life 1 1 Cell Structure...
Year 11 Biology Complete Course Notes 2024–2026 Madeleine Wainwright Contents I Cells as the Basis of Life 1 1 Cell Structure 2 1.1 Prokaryotic cells......................................... 2 1.2 Eukaryotic cells......................................... 2 1.3 Technologies for investigating cell structure and function.................... 3 1.3.1 Cell culturing...................................... 3 1.3.2 Light microscopy.................................... 3 1.3.3 Fluorescence microscopy................................ 4 1.3.4 Electron microscopy................................... 4 1.3.5 Cell fractionation.................................... 5 1.3.6 Immunohistochemistry................................. 5 1.3.7 Computational genomics................................ 5 1.3.8 PCR........................................... 5 1.4 Cell diagrams.......................................... 6 1.4.1 Eukaryotic cell diagrams................................ 6 1.4.2 Cells in the human body................................ 6 1.4.3 Prokaryotic cells..................................... 6 1.5 Comparison of cell organelles and arrangements........................ 7 1.6 Fluid mosaic model....................................... 8 2 Cell Function 9 2.1 Diffusion and osmosis...................................... 9 2.2 Active transport......................................... 11 2.3 Endocytosis and exocytosis................................... 11 2.4 Factors affecting exchange of materials............................. 12 2.4.1 Surface-area-to-volume ratio.............................. 12 2.4.2 Concentration gradients................................. 12 2.4.3 Characteristics of materials............................... 13 2.5 Cell requirements........................................ 13 2.6 Biochemical processes..................................... 14 2.6.1 Photosynthesis..................................... 14 2.6.2 Cell respiration..................................... 15 2.6.3 Removal of cellular products and wastes in eukaryotic cells.............. 16 2.7 Enzymes............................................. 16 2.7.1 Chemical structure of enzymes............................. 17 2.7.2 Lock and key model................................... 18 2.7.3 Induced fit model.................................... 18 2.7.4 Effect of environment on enzyme activity........................ 18 II Organisation of Living Things 21 1 Organisation of Cells 22 1.1 Types of organisms....................................... 22 1.1.1 Unicellular organisms.................................. 22 1.1.2 Colonial organisms................................... 22 1.1.3 Multicellular organisms................................. 22 1.2 Cell differentiation and specialisation.............................. 23 iv Copyright © 2024 InStudent Publishing Pty. Ltd. 2 Nutrient and Gas Requirements 27 2.1 Structure of Plants....................................... 27 2.1.1 Leaf........................................... 27 2.1.2 Stem........................................... 28 2.1.3 Root........................................... 29 2.2 Movement of products of photosynthesis............................ 29 2.3 Microscopic gas exchange structures.............................. 30 2.3.1 Mammals: alveoli.................................... 30 2.3.2 Plants: leaf structure.................................. 31 2.4 Macroscopic Gas Exchange Structures............................. 31 2.4.1 Mammals: lungs..................................... 31 2.4.2 Fish: gills........................................ 32 2.4.3 Insects: tracheae.................................... 32 2.5 Theories and models of plant structure and function...................... 33 2.5.1 Theory of photosynthesis................................ 33 2.5.2 Transpiration-Cohesion-Tension Theory........................ 34 2.6 Digestion processes....................................... 34 2.7 Autotrophs and heterotrophs nutrient and gas requirements.................. 36 3 Transport 37 3.1 Transport system in plants.................................... 37 3.2 Transport system in animals................................... 38 3.2.1 Circulatory system: mammals.............................. 38 3.2.2 Circulatory system: fish................................. 39 3.2.3 Circulatory system: insects............................... 40 3.3 Gas exchanges......................................... 40 3.3.1 Animals......................................... 40 3.3.2 Plants.......................................... 41 3.4 Comparing structures and functions of transport systems................... 41 3.4.1 Transport systems in animals.............................. 41 3.4.2 Vascular systems in plants and animals........................ 42 3.4.3 Changes in transport medium composition....................... 43 III Biological Diversity 44 1 Effects of the Environment on Organisms 45 1.1 Biotic and abiotic factors..................................... 45 1.2 Changes in a population..................................... 47 2 Adaptations 48 2.1 Structural adaptations...................................... 48 2.2 Physiological adaptations.................................... 48 2.3 Behavioural adaptations..................................... 49 2.4 Charles Darwin......................................... 49 2.4.1 Finches of the Galapagos Islands............................ 50 2.4.2 Australian flora and fauna................................ 50 3 Theory of Evolution by Natural Selection 51 3.1 Diversification of life on Earth.................................. 51 3.2 Microevolution and macroevolution............................... 53 3.3 Convergent and divergent evolution............................... 54 3.4 Punctuated equilibrium..................................... 56 Copyright © 2024 InStudent Publishing Pty. Ltd. v 4 Evolution – the Evidence 57 4.1 Evidence in support of evolution................................. 57 4.2 Techniques for dating fossils................................... 60 4.2.1 Absolute dating methods................................ 60 4.2.2 Relative dating methods................................. 60 4.3 Examples of modern evolutionary change........................... 61 IV Ecosystem Dynamics 62 1 Population Dynamics 63 1.1 Ecosystem relationships..................................... 63 1.1.1 Impact of abiotic factors................................. 63 1.1.2 Impact of biotic factors................................. 64 1.1.3 Components of the biosphere.............................. 64 1.1.4 Ecological niches.................................... 65 1.1.5 Consequences of predation, competition, symbiosis, and disease........... 65 1.1.6 Measurement of populations.............................. 66 1.2 Extinction events......................................... 67 1.2.1 Cretaceous-Paleogene extinction event......................... 67 2 Past Ecosystems 69 2.1 Palaeontological and geological evidence of ecosystem changes............... 69 2.1.1 Aboriginal rock paintings................................ 69 2.1.2 Rock structure and formation.............................. 70 2.1.3 Ice core drilling..................................... 70 2.2 Technological evidence of ecosystem changes......................... 71 2.2.1 Radiometric dating................................... 71 2.2.2 Gas analysis...................................... 72 2.3 Present-day organism evolution................................. 73 2.3.1 Evidence for evolution of small mammals........................ 73 2.3.2 Evidence for evolution of sclerophyll plants....................... 74 2.4 Reasons for ecosystem changes................................ 74 3 Future Ecosystems 76 3.1 Human-induced selection pressures causing species extinction................ 76 3.2 Models for predicting future impacts on biodiversity....................... 76 3.2.1 Impact of climate change on ecosystems........................ 77 3.3 Restoring damaged ecosystems................................ 77 3.3.1 Restoration of mining sites............................... 77 3.3.2 Restoration of agricultural land............................. 78 vi Copyright © 2024 InStudent Publishing Pty. Ltd. Module I Cells as the Basis of Life Copyright © 2024 InStudent Publishing Pty. Ltd. 1 Cell Structure Section 1 Cell Structure S YLLABUS : Inquiry question: What distinguishes one cell from another? 1.1 Prokaryotic cells S YLLABUS : Investigate different cellular structures, including but not limited to: Examining a variety of prokaryotic and eukaryotic cells Describe a range of technologies that are used to determine a cell’s structure and function K EY P OINT : Prokaryotic cells: cells which do not have a membrane-bound nucleus. Prokaryotes are unicellular organisms which do not have a membrane-bound nucleus, mitochondria, or any Section 1 – Cell Structure membrane-bound organelles (organelles enclosed within membranes). There are two types of prokaryotes: archaea and bacteria. Electron microscope image of E. coli bacteria Electron microscope image of Halobacterium archaea 1.2 Eukaryotic cells K EY P OINT : Eukaryotic cells: cells with a nuclear membrane and organelles. Eukaryotes are organisms which have a nucleus and other organelles which are enclosed within membranes. The nuclear envelope encloses the nucleus. Eukaryotes may be unicellular or multicellular. Animal, plant and fungi cells are all eukaryotic. Electron microscope image of an animal cell 2 Copyright © 2024 InStudent Publishing Pty. Ltd. 1.3 Technologies for investigating cell structure and function Electron microscope image of a plant cell Electron microscope image of a fungi cell 1.3 Technologies for investigating cell structure and function A range of technologies have been developed to expand the fields of cell biology and microbiology, allowing a deeper understanding of the prokaryotic and eukaryotic organisms which populate the earth. 1.3.1 Cell culturing Cell culturing is the process scientists use to grow cells under controlled conditions in vitro (meaning outside of normal bio- logical conditions; literally, ‘in the glass’). Each cell culturing Section 1 – Cell Structure method is specialised, and will depend on the organism you are trying to grow. So how do we grow cells in vitro? We need to replicate their natural growth conditions. Firstly, pick an appropriate medium. Most cells like to have an adherent surface to grow on (for example, agar), but some can be grown free-floating in medium. Next, we need to ensure that the growth media has the right nutrients for your organism to grow. Addition of a food source (carbohydrates), amino acids, vitamins, miner- als, gases (CO2 and O2 ) and growth factors are important. It is also essential that the culture conditions are regulated, so Agar plate culturing method a pH buffer is added, and temperature kept constant. 1.3.2 Light microscopy Light microscopy is the passing of visible light through a sample, with the use of lenses to mag- nify the image. It can be used to successfully im- age objects from 1 millimetre to 0.2 micrometers in size. Staining methods can be used to enhance the appearance of cell structures under the microscope. A very common staining technique is Gram staining, which colours bacterial cell membranes either pink or purple, and can therefore help in identifying the type of cell. Scientific diagram of a light microscope Copyright © 2024 InStudent Publishing Pty. Ltd. 3 1.3 Technologies for investigating cell structure and function Gram negative bacteria Gram negative bacteria 1.3.3 Fluorescence microscopy Fluorescence microscopes are able to detect fluorescence or phosphorescence, often producing images which can identify certain aspects of cells. For example, mammalian cells can be stained with fluorescent compounds which bind to nucleic acids, enabling us to identify where the nucleus of the cell is. Fluorescent microscopy is also useful to identify cells which express fluorescent reporter proteins, a common technique in bacterial culturing. 1.3.4 Electron microscopy Electron microscopes use a beam of electrons to illuminate objects. As electrons have significantly smaller wavelengths compared to visible light photons, electron microscopy can image objects on a much smaller Section 1 – Cell Structure scale, anywhere from 1 millimetre to 0.1 nanometre. It also provides more detail than light microscopy. This allows a greater observation of structures within cells, such as organelles, which might be difficult to distinguish under a light microscope. A drawback of electron microscopy, however, is that the images must be produced in a vacuum. This means that cells must be dead to be imaged, so we are not able to view cellular processes in action and the structure of the cell may be slightly altered. It is also significantly more expensive than light microscopy. 4 Copyright © 2024 InStudent Publishing Pty. Ltd. 1.3 Technologies for investigating cell structure and function 1.3.5 Cell fractionation Cell fractionation is a process used by scientists to separate out different cell components so that they can be analysed individually. Cell component function is preserved, allowing us to conduct experiments which test what the component does, as well as its structure. An example of a fractionation technique is differential centrifugation, which separates organelles by their density. 1.3.6 Immunohistochemistry All cells have different protein markers, antigens, coating their exterior. Antigens act as tissue identifiers. For each antigen, there exists a specifically shaped complementary antibody which binds to it. Immunohisto- chemistry is a technique which takes advantage of these different antigens/antibody pairings by conjugating marker molecules to antibodies, and then introducing them into a tissue culture. The antibodies will bind to their complementary tissue antigens, and these can be imaged due to the presence of the enzyme attach- ment. This technique allows us to identify different biological tissues, and can identify the location of specific proteins within a tissue. Section 1 – Cell Structure 1.3.7 Computational genomics Computational genomics is the technique of analysing genomic information (DNA and RNA) with computa- tional and statistical techniques. It enables us to understand gene sequences, and their associated functions. By deciphering patterns of genomic sequences, computational genomics has enabled us to make predictions about how cell signalling works, and which genomic regions are associated with embryonic development. 1.3.8 PCR PCR (Polymerase Chain Reaction) is a technique used across a number of fields of biological research to amplify genes which are present in cells. By isolating and amplifying genes, we are able to better identify what genetic information is present, and therefore what functional and structural characteristics a cell may have. S YLLABUS : Investigate a variety of prokaryotic and eukaryotic cell structures, including but not limited to: Drawing scaled diagrams of a variety of cells Comparing and contrasting different cell organelles and arrangements Modelling the structure and function of the fluid mosaic model of the cell membrane Copyright © 2024 InStudent Publishing Pty. Ltd. 5 1.4 Cell diagrams 1.4 Cell diagrams 1.4.1 Eukaryotic cell diagrams Animal Cell Plant Cell Section 1 – Cell Structure 1.4.2 Cells in the human body Neuron Epithelial Cell Red blood cell 1.4.3 Prokaryotic cells Bacteria: Escherichia coli 6 Copyright © 2024 InStudent Publishing Pty. Ltd. 1.5 Comparison of cell organelles and arrangements 1.5 Comparison of cell organelles and arrangements Cell structure, including the types of organelles and their arrangements, is varied depending of the function of the cell. As eukaryotic cells are often found in multicellular arrangements (e.g. animals and plants), they are much more complex. Contrastingly, prokaryotic cells are unicellular, and have markedly less complex machinery, as processes occurring in the cell are required only to keep that one organism alive. Below is a table summarising the major differences between prokaryotic and eukaryotic cells, with the function of major organelles described. Cellular Feature and definition Eukaryotic Prokaryotic Nucleus: membrane enclosed organelle containing Yes, bound to the No genetic information membrane Nucleic acid storage Multiple chromosomes Nucleoid and plasmids within the nucleus (circular DNA) Endoplasmic reticulum: network of Yes, present in both No membrane-enclosed sacs involved in protein and lipid smooth and rough form synthesis Ribosomes: composed of RNA, molecular structure Yes, found bound to the Yes, free-floating in that is the site of protein synthesis rough endoplasmic the cytoplasm; smaller reticulum; larger than than the eukaryotic Section 1 – Cell Structure prokaryotic type type Mitochondria: organelles responsible for the majority Yes No of ATP (energy) production in the cell Cytoskeleton: structures which give the cell shape, Yes May be absent organise cellular parts, and are involved in transport of depending on cell type molecules around cells, and are composed of microtubules, actin filaments and intermediate filaments Lysosomes: cytoplasmic organelle containing Yes No enzymes to break down biomolecules, responsible for waste disposal Golgi apparatus: responsible for packaging proteins Yes No for transport around the cell Chloroplasts: organelles containing chlorophyll, Yes in plant cells No, photosynthetic responsible for photosynthesis prokaryotes have chlorophyll dispersed in cytoplasm Cell wall: structural layer outside the cell membrane Yes in plant cells and Yes fungi Vacuole: vesicle found in cytoplasm, containing fluids Yes Yes (wastes, water), and providing structural support (turgor) Flagella: ‘tail’ present in some cells, responsible for Yes, long and rod-like, Yes, composed of one movement composed of multiple fibre, sub-microscopic fibres, microscopic Size 10 – 100 µm 1 – 10 µm Copyright © 2024 InStudent Publishing Pty. Ltd. 7 1.6 Fluid mosaic model 1.6 Fluid mosaic model Cellular membranes (also known as plasma membranes) are composed of a phospholipid bi-layer, which forms a selectively permeable protective layer around cellular contents. The membrane controls movement of ions and organic molecules in and out of the cell, performing an impor- tant role in maintaining cell structure and function. It is also responsible for cell adhesion (the binding of cells to their environments), and cell signalling (communication between cells). Section 1 – Cell Structure The current theory of how the cell membrane functions is called the ‘fluid mosaic model’. This model describes the cell membrane as a two-dimensional liquid, which restricts diffusion of molecules across it’s lipid bilayer. The lipid bi-layer is formed as the hydrophilic ‘heads’ face outward, and the hydrophobic ‘tails’ bind together inwardly to form a structure with two layers. Components of the cellular membrane, including proteins, choles- terol and carbohydrates, and are incorporated into the phospholipid bilayer. They are not static, but move around the membrane as required, depending on the cellular environment. They perform important functions, such as channelling specific molecules across the membrane, maintaining structural integrity, and allowing for cell recognition. 8 Copyright © 2024 InStudent Publishing Pty. Ltd. Cell Function Section 2 Cell Function S YLLABUS : Inquiry question: How do cells coordinate activities within their internal environment and the external environment? The plasma membrane which surrounds the cell is selectively permeable. This means that it only allows cer- tain substances to move in and out, through a number of different mechanisms. This permeability ensures that the cell only collects molecules that it requires (such as glucose, lipids, and amino acids), and excludes other materials. It also allows the cell to excrete unwanted molecules, such as metabolic wastes, so that the cell can maintain a constant internal environment. There are a number of mechanisms used to pass materials through the cell membrane, including diffusion, osmosis, active transport, endocytosis, and exocytosis. S YLLABUS : Investigate the way in which materials can move into and out of cells, including but not limited to: Conducting a practical investigation modelling diffusion and osmosis Examining the roles of active transport, endocytosis and exocytosis Relating the exchange of materials across membranes to the surface-area-to-volume ratio, concen- tration gradients and characteristics of the materials being exchanged Section 2 – Cell Function 2.1 Diffusion and osmosis K EY P OINT : Diffusion: the movement of molecules from an area of high concentration to an area of low concentration, until an equilibrium is reached. It does not require an energy input. When a molecule moves in such a way, it is called moving down the concentration gradient. In biological terms, this refers to diffusion across a semi-permeable membrane. Molecules which are needed for cell function, such as water and ions, use diffusion to move in and out of cells. Diffusion is a passive transport mechanism, meaning that no input of energy is required for the molecules to move through the membrane. Diffusion can also be facilitated by the cell. This means a mechanism, such as an ion channel, exists across the membrane to flow certain molecules through. Osmosis is a subset of diffusion, referring solely to the passive movement of water across a membrane, to equalise the concentration of a solute on both side. Diffusion and osmosis are used by the cell to regulate their internal conditions, ensuring optimal cell function. At normal, balanced water levels a cell is isotonic in solution. When there is too much water within a cell, it is hypotonic, and may lyse due to increased pressure on the membrane. When there is too little water within a cell, it is hypertonic, and may shrivel due to the lack of water. Copyright © 2024 InStudent Publishing Pty. Ltd. 9 2.1 Diffusion and osmosis To model the process of diffusion by passive transport, a simple practical task can be performed: Aim To investigate the movement of molecules and ions across a semi-permeable membrane Hypothesis That only the small molecules and ions, water and iodine, will be able to diffuse across the membrane, while starch will remain enclosed by the membrane Materials Dialysis tubing, beaker, measuring cylinder, water, starch solution, iodine, stopwatch Method 1. Using a measuring cylinder, pour 10ml of starch solution into the dialysis tubing. Tie off the end to seal. 2. Add 100ml of water to the beaker, and add up to 5 drops of iodine (until the water appears a faint yellow colour). 3. Place the dialysis tube containing the starch solution into the beaker. 4. At 15 minute intervals, observe any changes to the colour of the solutions, and record results. 5. Control: Repeat steps 1–4, without the addition of iodine. Results Table of results (approximate): Time (minutes) Observations 0 Liquid within dialysis tubing clear. Liquid outside of dialysis tubing clear with amber Section 2 – Cell Function yellow colour. 15 Liquid within dialysis tubing medium purple colour. Liquid outside of dialysis tubing faint yellow colour. 30 Liquid within dialysis tubing dark purple colour. Liquid outside of dialysis tubing very faint yellow colour. Image of beaker after 30 minutes: Conclusion When in the presence of starch, iodine becomes a dark purple colour. As seen in the results, this reaction occurred within the dialysis tubing, but not in the rest of the beaker. This displays that the iodine was able to diffuse across the semi-permeable membrane from a solution of high concentration of iodine molecules, to a solution with low concentration of iodine molecules, within the tubing. Starch, however, was unable to diffuse out the tubing across the membrane, because the molecules are too large to be passively moved across a membrane. Therefore, it stayed within the tubing, and the solution on the outside remained a clear, yellow colour. 10 Copyright © 2024 InStudent Publishing Pty. Ltd. 2.2 Active transport 2.2 Active transport K EY P OINT : Active transport: the movement of molecules across a membrane requiring an input of energy – often ATP. Active transport occurs in cells when molecules are either too large to move across a membrane on their own, or when molecules are trying to move against a concentration gradient (i.e. from areas of low concentration to high concentration). Section 2 – Cell Function Active transport is important for moving molecules, such as glucose, in and out of the cell. It is also essential for creation of membrane potentials; which cells use to drive production of ATP in the mitochondria. 2.3 Endocytosis and exocytosis Endocytosis is a type of active transport used to bring external materials, such as proteins, into the cytoplasm of a cell. It does so by engulfing them in part of the outer membrane, forming a vesicle inside of the cell cytoplasm. Exocytosis is the opposite of endocytosis, a mechanism for cells to actively transport large or charged molecules to the extra-cellular space. It is also used as a method to add proteins, lipids, and other molecules to the cell membrane. Copyright © 2024 InStudent Publishing Pty. Ltd. 11 2.4 Factors affecting exchange of materials 2.4 Factors affecting exchange of materials 2.4.1 Surface-area-to-volume ratio The process of diffusion for exchanging nutrients and wastes can be effected by a number of factors, such as the ratio of the cell surface area to volume ratio. The term ‘surface-area-to-volume ratio’ refers to the ratio between the external membrane surface of a cell and the size of its contents. The larger a cell gets, the smaller it’s surface area to volume ratio becomes, because more cytoplasm is being contained in a relatively smaller coating of membrane. 2 If we consider to be perfect spheres, the surface area can be calculated as 4π r and volume of the cell cells 4 Section 2 – Cell Function calculated as π r2 where r is the radius of the cell. Therefore, to find the surface-to-volume ratio, we 3 3 calculate. r As the surface-area-to-volume ratio decreases, the efficiency of diffusion also decreases, as the cell contents are less available to the site of exchange. This decreases the efficiency of the cell, as it will not be able to excrete waste and receive nutrients at a rapid enough pace, as these molecules are travelling longer distances to the membrane. This is why cells are restricted in their size, and large organisms such as humans require the coordination of many cells to perform large-scale functions. 2.4.2 Concentration gradients The concentration of a solute will also effect the rate at which it diffuses. The greater a difference in concen- tration across a membrane, the more rapidly it will diffuse. As the system approaches equilibrium, this rate will slow. 12 Copyright © 2024 InStudent Publishing Pty. Ltd. 2.5 Cell requirements 2.4.3 Characteristics of materials Whether or not material can be exchanged across a membrane, and the type of transport used, is determined by the physical and chemical properties of a molecule, and how they interact with the cell membrane. Firstly, the mass of the molecule will affect transport, as large molecules will generally be unable to pass through the selectively permeable membrane without the aid of a transporter protein. This helps maintain the integrity of the membrane, and ensures that only required molecules are able to enter a cell. The solubility of a molecule will also affect how it moves across a membrane. Solubility refers to whether a substance is polar or non-polar. Non-polar substances move more easily through the cell membrane than polar molecules, and therefore will be diffused at a faster rate. 2.5 Cell requirements S YLLABUS : Investigate cell requirements, including but not limited to: Suitable forms of energy, including light energy and chemical energy in complex molecules Matter, including gases, simple nutrients and ions Removal of wastes In order to maintain life, cells require certain fundamental molecules. The types of molecules required vary depending on the types of cells and organisms. Essentially, all cells require an energy source and a carbon source, in order to drive all cell processes. Section 2 – Cell Function K EY P OINT : Autotrophs: organisms which are able to produce their own food from their surroundings, using either photosynthesis or chemosynthesis. Photoautotrophs use light as an energy source, whereas chemoautotrophs use chemicals, such as sulphur, as an energy source. Autotrophs are essential, because they form the basis of the food chain, producing more complex molecules such as glucose from basic inorganic molecules; CO2 and water using sunlight. K EY P OINT : Heterotrophs: organisms which are not able to synthesise their own food, so must rely on consumption of other organisms or external carbon molecules for their nutritional carbon source. For example, humans consume plants in order to obtain carbohydrates, proteins, and nutrients essential for cellular function. Heterotrophs can either be light or chemically dependent for their energy sources. Humans are an example of chemoheterotrophs, as they gain both their carbon and energy sources from chemicals they consume. Copyright © 2024 InStudent Publishing Pty. Ltd. 13 2.6 Biochemical processes Apart from an energy source and a carbon source, cells also require other molecules to aid in metabolic processes. Animal cells require O2 gas in order to conduct respiration (as we will discuss further below), and plant cells require CO2 for photosynthesis. Ions are also essential for cell functioning, performing important roles in protein function as cofactors (Mg2+ , Mn2+ , Fe2+ ), in creating cellular conditions for respiration (H+ , Na+ ), and in electrical communication, particularly for neurons (Na+ , K+ , Ca2+ ). There are also a number of vitamins essential for cell structure and function, such as Vitamin C for collagen synthesis. As outlined in the previous section, there are a number of mechanisms which allows transport of these essen- tial molecules into the cell. Diffusion, active transport, endocytosis, exocytosis, and the activity of organelles such as vacuoles and lysosomes allow entrance of molecules into the cell, and expulsion of wastes. In an- imals, the blood is responsible for collecting the cellular waste, and removing it from the body. Removal of wastes, as we will investigate further in the following sections, is vital to the proper functioning of the cell and its components. 2.6 Biochemical processes S YLLABUS : Investigate the biochemical processes of photosynthesis, cell respiration and the removal of cellular prod- ucts and wastes in eukaryotic cells. Cell biology is dependent on a number biochemical processes, which power cells, recycle waste, and produce essential molecules for structure and function. The biochemical processes that occur in a cell are called Section 2 – Cell Function ‘metabolism’. So firstly, we need to understand, what is metabolism? K EY P OINT : Metabolism: the sum of all chemical processes which take place within an organism. Metabolism therefore includes all anabolic (biochemical processes which synthesise molecules) and catabolic (biochemical processes which breakdown molecules) processes that occur within a cell. Different cells have different biochemical pathways, each contributing to how an organism will function. This is important especially in multicellular organisms, such as humans, as we require specialised cells to perform specialised tasks to maintain health. For example, our liver cells, hepatocytes, are responsible for storing excess glucose, contributing to the function of the liver. Cells also have specialised organelles to perform biochemical functions, as we will see as we begin to understand these processes in more depth. 2.6.1 Photosynthesis Photosynthesis is a process used by autotrophs, ‘self-feeding’ organisms, to produce their own glucose. This can then be used in respiration to produce energy. Non-photosynthetic organisms, heterotrophs, rely upon consumption of external sources of glucose (for example, plants) in order to obtain molecules essential for respiration. The process of photosynthesis converts light energy into chemical energy, and stores this energy in the bonds of glucose molecules. In this process, carbon dioxide and water are converted to glucose and oxygen in the presence of light. K EY P OINT : Photosynthesis: Carbon dioxide + Water + Light −→ Glucose + Oxygen 6CO2 + 6H2 O + light −→ C6 H12 O6 + 6O2 14 Copyright © 2024 InStudent Publishing Pty. Ltd. 2.6 Biochemical processes Chloroplasts are the specialised organelles responsible for performing photosynthesis in plants and algae. They obtain their green colour from chlorophyll, a green pigment which is excited by certain wavelengths of light. This is how the light energy is converted into chemical energy. Once glucose has been produced, it is moved around the organism to cells which need it to produce energy. Excess glucose is stored as saccharide structures in plants (for example, fruit and vegetables). 2.6.2 Cell respiration One essential biochemical process required for all cells is the ability to produce energy. This is essential so that the cell can breakdown or build-up molecules that it needs to survive. The process of producing energy is called respiration. Contrary to how we commonly use the word respire, in terms of cell biology it does not mean breathing, but production of energy. There are two types of respiration, aerobic, meaning in the presence of oxygen, and anaerobic, meaning without oxygen. Whether or not a cell will use aerobic or Section 2 – Cell Function anaerobic respiration will depend on the type of cell (e.g. whether it is a bacterium found in soil, or a human brain cell), and potentially the availability of oxygen (some human cells can perform anaerobic respiration when there is limited oxygen available, for example during exercise). Respiration always requires a food source, glucose, which is broken down to produce by-products, and energy. The energy released comes from the breaking of chemical bonds during the process. K EY P OINT : Aerobic respiration: Glucose + Oxygen −→ Carbon dioxide + Water + Energy C6 H12 O2 + 6O2 −→ 2CO2 + 6H2 O + ATP energy Aerobic respiration happens consistently in animal and plant cells, which is why they need a constant supply of oxygen to stay alive. Many of the steps involved require the mitochondria (the powerhouse of the cell!) The process is complex and requires many steps, which are summarised in the diagram below. Copyright © 2024 InStudent Publishing Pty. Ltd. 15 2.7 Enzymes Anaerobic respiration is the production of energy without oxygen. Different cells have different pathways for this, but some of the common ways cells perform anaerobic respiration have been identified below: K EY P OINT : Anaerobic respiration in human cells: Glucose −→ Lactic acid + Energy C6 H12 O6 −→ 2C3 H6 O3 + ATP energy We can see from above that human cells are able to use glucose to produce lactic acid and energy, when the cells are starved of oxygen. This is why when you run, you can get cramps or pain in your leg muscles, because there is a build-up of lactic acid affecting your muscle cells. Microbes, such as yeast, are also able to respire without oxygen. This is how we make alcohol, through the process of fermentation (another word for anaerobic respiration). K EY P OINT : Anaerobic respiration in yeast cells: Glucose −→ Ethanol + Carbon dioxide + Energy C6 H12 O6 −→ 2C2 H5 OH + CO2 + ATP energy 2.6.3 Removal of cellular products and wastes in eukaryotic cells There are a number of organelles which are responsible for removing wastes from cells. It is essential that Section 2 – Cell Function wastes are removed for continued, optimal function of cells, as we will see later in our discussion of enzymes. Vacuoles: are membrane bound enclosed compartments, containing dissolved inorganic and organic molecules, enzymes, and in some cases solids. They isolate material that may be harmful to the cell, aid in regulating the pH of the cell by up taking protons, contain waste products, and assist in transport of substances from the cell (through exocytosis). Lysosomes: are vesicles (lipid-bilayer sphere) which dispose of cell wastes. They contain hydrolytic enzymes, functioning best at acidic pH ranges (4.5–5.0), which break down biomolecules. Lysosomes digest unwanted cell material to aid in maintaining cellular function, as well as breaking down pathogens entering the cell. 2.7 Enzymes S YLLABUS : Conduct a practical investigation to model the action of enzymes in cells. What are enzymes? Enzymes are proteins found in all cells which are responsible for speeding up, or catalysing, chemical reactions happening in organisms. For example, when cells are breaking down glucose for energy, the molecule might eventually break down of its own accord, but that would take years. So to speed up the breaking down of chemical bonds, we have enzymes, allowing glucose digestion to happen in a matter of seconds, and a quick release of energy for cells to use. Enzymes are essential in all areas of life, and without them organisms would just be blobs of chemicals slowly reacting over millions of years. K EY P OINT : Enzymes: highly specific biological catalysts which increase the rate of metabolic reactions by lowering the ‘activation energy.’ 16 Copyright © 2024 InStudent Publishing Pty. Ltd. 2.7 Enzymes Enzymes are able to catalyse reactions by lowering the activation energy, the energy needed to begin a reaction between two molecules. Less energy is needed to breakdown or synthesise a molecule when an enzyme is present to facilitate a reaction, as demonstrated by the graph below. 2.7.1 Chemical structure of enzymes Enzymes are made of amino acids, which are bonded in a specific linear order, and then folded to form proteins. Section 2 – Cell Function Protein folding occurs on four levels to influence the final shape. All of these interactions are chemical bonds, which can be effected by the environment in which the protein is situated. Copyright © 2024 InStudent Publishing Pty. Ltd. 17 2.7 Enzymes The way in which enzymes interact with biomolecules to speed up chemical reactions is with their active site. The active site is an area of the enzyme which contains a specific sequence of amino acids with reactive side chains, that can chemically interact with a substance. The biomolecules, called substrates, bind to the active site and undergo a chemical reaction. The shape and reactivity of enzyme active sites makes them very specific, and they are usually only able to act on one type of substance. 2.7.2 Lock and key model There are two main theories about how enzymes and substrates interact to catalyse reactions. Firstly, the ‘lock and key’ model describes the enzyme as a ‘key’, and the substrate a ‘lock’. When the key interacts with the lock, the lock changes shape, but the key remains unchanged for continued use. This is analogous to a substrate interacting with an enzyme, forming an enzyme-substrate complex, and the separating, the substrate changed into products, and the enzyme conserved. 2.7.3 Induced fit model Section 2 – Cell Function The induced fit model is another theory about how enzymes and substrates interact, elaborating upon the lock and key model. The enzyme’s active site it not perfectly shaped to accommodate the substrate, but changes shape to bind the substrate and act on it. 2.7.4 Effect of environment on enzyme activity S YLLABUS : Investigate the effects of environment on enzyme activity through collection of primary or secondary data. As described in the previous section, enzymes are highly specific, and due to their chemical nature, they are significantly affected by changes to their environment. There are a number of scenarios in which enzyme activity is effected by environment, including: changing pH, changing temperature and changing substrate concentration. When factors such as these are altered, there may be changes either to the enzyme’s active site, or to the rate of substrate-enzyme interactions. When the shape protein is significantly altered, it is called denaturation (loss of quaternary, tertiary, and secondary folding structures). Most importantly, this changes the shape of the active site, so the enzyme can no longer act on its substrate. This denaturation can happen at increased temperatures, or at pH outside of the optimal ranges. It occurs because, as we discussed in the previous section, enzymes are made of amino acids, whose chemical bonds can be changed depending on the acidity, alkalinity, or temperature of their environment. 18 Copyright © 2024 InStudent Publishing Pty. Ltd. 2.7 Enzymes Effect of pH pH is a measure of the amount of hydrogen ion (H+ ) in solution. These charged ions in solution have an effect upon the chemical structure of enzymes, as they can interfere with the bonds which determine the shape of the protein. At pH ranges both too acidic and too alkaline, enzymes with denature. Below is an outline of how experimental procedures to investigate enzyme activity may be conducted. Aim To identify the effect of pH on the rate of catalase activity Hypothesis The catalase enzyme will have the fastest rate of reaction around a neutral pH Materials Potato, hydrogen peroxide, buffer solutions at pH 3, 5, 7, 9, and 11, measuring cylinders, test tubes, stopwatch, ruler, scalpel, mass balance Methods Part A: Determining reactivity of enzyme at varied pH 1. Prepare five 1cm3 cubes of potato using a ruler to measure accurately the dimensions and a scalpel to cut. 2. Using an electronic balance, determine the weight of each piece of potato, and where weight difference occurs, recut to make each piece equal. 3. Place one piece of potato into a test tube. 4. Using a measuring cylinder, add 5mL of pH 3 buffer into the test tube containing the potato sample. 5. Add 5mL of H2 O2 into the test tube containing the potato and buffer, and start timer Section 2 – Cell Function immediately. 6. Stop timer at 3 minutes and use a ruler to measure height of bubble column. 7. Record results in table. 8. Repeat steps 3–7 for each other pH buffer (5, 7, 9, 11) 9. Repeat process 3 times for each pH. Part B: Controls Control 1: Place one piece of potato into a test tube. Observe and record any reactions. Control 2: Measure 5ml of pH 3 buffer solution using a measuring cylinder, and add to a test tube. Measure 5mL of H2 O2 and add to cylinder, immediately beginning timer. Stop timer at 3 minutes and record any observations of reaction. Controls 3-6: Repeat step 2, varying the pH of buffer solution used (5, 7, 9, and 11). Results Table of experimental results (approximate): pH of solution in test tube 3 5 7 9 11 Height of bubbles produced 0 1 5 1 0 As can be seen in the table above, the pH at which the most bubbles were produced was 7. At pHs 5 and 9, reduced levels of bubbles were observed at 1cm of foam. At pHs 3 and 11, no bubbles were observed in the test tubes. The shape of these results is a clear bell-curve, with a peak at pH 7. K EY P OINT : Remember to state your results without analysing them. Just explain what the data means in clear lan- guage, and do not make any inferences. Copyright © 2024 InStudent Publishing Pty. Ltd. 19 2.7 Enzymes Conclusion Independent variable: change in pH of solution Dependent variable: change in rate of enzyme activity, observed as bubbles of oxygen Controlled variables: temperature, size of test tube, amount of potato used, amount of hydrogen peroxide added. Catalase is an enzyme which breaks hydrogen peroxide down into water and oxygen. The observable result of the rate of catalase activity in our experiment were the bubbles of oxygen produced. As seen in our results, the pH at which the most oxygen bubbles was observed was 7. This indicates that the enzyme has the highest rate of activity at pH 7. At more acidic pH of 5, and basic pH of 9, less activity (only 1cm) of bubbles was observed. This suggests that the enzyme begins to denature within the range of these pH. At significantly more acidic and basic pH of 3 and 11, no activity was observed, suggesting that the enzyme is completely denatured at these pH. In order for the enzyme to fully perform its function at an optimal rate, the results from this experiment suggest that a pH of 7 should be maintained. Effect of temperature Temperature is another environmental factor which effects the rate of enzyme activity. At low temperatures, increasing towards the optimal temperature, the rate of reaction is limited due to the energy in the system. This simply means that the substrate and enzyme are not encountering each other as quickly because they move slower at cooler temperatures. Past the optimal temperature, the enzyme will begin to denature as the heat is altering the chemical bonds within the protein. This changes the shape of the active site, so that the substrate can no longer interact with it, and the rate of activity quickly drops off. Section 2 – Cell Function Effect of substrate concentration The amount of substrate available to react with an enzyme will also affect the rate of reaction. As substrate concentration increases, the rate of reaction will increase, as more chemical reactions are happening. This will occur until the point at which all enzymes are engaged in an enzyme-substrate complex, at which point the rate of reaction will plateau. The reason for this is that a set amount of enzyme can only perform some many reactions at a certain rate, so not matter how much more substrate you add, the enzymes rate of reaction will not increase. 20 Copyright © 2024 InStudent Publishing Pty. Ltd. Module II Organisation of Living Things Copyright © 2024 InStudent Publishing Pty. Ltd. 21 Organisation of Cells Section 1 Organisation of Cells S YLLABUS : Inquiry question: How are cells arranged in a multicellular organism? 1.1 Types of organisms S YLLABUS : Compare the differences between unicellular, colonial and multicellular organisms by: Investigating structures at the level of the cell and organelle Relating structure of cells and cell specialisation to function 1.1.1 Unicellular organisms Unicellular organisms are organisms which are made of only one cell. The main types of unicellular organisms Section 1 – Organisation of Cells are archaea, bacteria, and protozoa. Unicellular organisms can be either prokaryotic (archaea, bacteria) or eukaryotic (protozoa, unicellular algae, and fungi). They are theorised to be the oldest forms of life. The mark of a unicellular organism is that they must carry out all metabolic processes to sustain life within the one cell. These differ from multicellular organisms, which require other cells for survival. Therefore, we can say that in unicellular organisms, the division of labour (i.e. delegating tasks to sustain life) is at the organelle level, and for each task there is low operational efficiency. As unicellular organisms must carry out all life processes they have simplified, yet effective, cell and organelle structures. It is essential that these organisms have a functioning cellular membrane, as they are surrounded by the external environment. The membrane is essential for excluding unwanted substances, and allowing transport of essential nutrients into the cell. For this reason, the cells also cannot be too large. Recalling our discussion on surface area to volume ratio on page 12, this makes sense, because if the cell was too large, substances wouldn’t be able to move in and out of the cell efficiently. Due to the burden of performing all metabolic functions, unicellular organisms are not overly specialised. Organelles which will always be found within a unicellular organism include a genome containing genetic information and ribosomes for protein production. 1.1.2 Colonial organisms Colonial organisms are organisms which form colonies in which the cells are physically connected and inter- dependent. They can either be unicellular, or multicellular. Colonial organisms are thought to have been a stepping stone in evolution from unicellular to multicellular organisms. These growth formations are advanta- geous, as physical proximity allows effective distribution of nutrients. Microbial colonies can grow in structures called biofilms, often containing multiple species. This type of growth is useful for microbes, as it affords the group capabilities much greater than that of the individual organism. Biofilms can be resistant to drugs due to the presence of a protective matrix, and allow stability during changes to the environment. 1.1.3 Multicellular organisms Multicellular organisms are organisms which consist of more than one cell. All animals are multicellular, as well some fungi and algae. Multicellular organisms are dependent on all cells within their system in order to survive. Each type of cell within an organism will perform a specific function, and all cells will work in complementary ways, contributing to overall health. 22 Copyright © 2024 InStudent Publishing Pty. Ltd. 1.2 Cell differentiation and specialisation In multicellular organisms, the division of labour is not at the organelle level, but at the cellular level (or, if the organism is more complex, such as humans, at the tissue or organ level). This allows for multicellular organisms to be very efficient, because they have a large group of cells dedicated to a certain function, so the function is performed in bulk. Cell specialisation, as we will discuss in the next dot point, is very important, as it allows for cells to perform very different tasks. For example, the human body has many highly specialised cells, such as nerve cells which pass electrochemical signals, or epithelial cells which absorb nutrients in the gut. As there are many cells within a multicellular organism, they can be very large in size, although this requires a highly specified organisation of tissues (as we will discuss in the next few sections). The increased ability to effectively carry out metabolic functions means that multicellular organisms can live for long periods of time. Unicellular Multicellular Composition Single cell which Many cells which each carry out carries out all cell specialised functions processes Division of labour Organelle level Cell, tissue, and organ Exposure Exposed at all Only outer cells (specialised to membrane surfaces protect the rest of the tissues) Section 1 – Organisation of Cells Size Small Large Life span Short Long Reproduction rate Quick Slow 1.2 Cell differentiation and specialisation S YLLABUS : Investigate the structure and function of tissues, organs and systems and relate those functions to cell differentiation and specialisation. K EY P OINT : Cell differentiation: the process by which a less specialised cell changes to become a specialised type of cell. For example, the process of a blood stem cell differentiating into a red blood cell. Cell specialisation: the specific function which a cell has, determined by their physiology and cellular structures. For example, red blood cells are specialised with haemoglobin molecules to carry oxygen. Stem cells are un-differentiated cells. When organisms begin development as embryos, all our cells are em- bryonic stem cells. As the embryo continues to grow and cells divide, these stem cells begin to differentiate, so that the cells become certain types of cells which perform specific functions. The changing of a stem cell into a type of cell is differentiation, and the final form they take is specialisation. This is important, because neuron in the brain has very different capabilities to a skin cell, but each are important in their different ways to the overall functioning of an organism. We also have adult stem cells in certain parts of our bodies, such as the bone marrow, which continue to differentiate and replenish stocks of blood cells. Copyright © 2024 InStudent Publishing Pty. Ltd. 23 1.2 Cell differentiation and specialisation Section 1 – Organisation of Cells K EY P OINT : Tissue: a group of cells which work together to perform a function. For example, muscle cells work together in muscle tissues to produce motion in the body. Tissues are collections of specialised cells, which work cohesively to perform a set function. They are spe- cialised because their cell organelles are specifically designed to undertake certain tasks in the body. It is important that cells are specialised, so that a myriad of different complex tissues can be formed. Some examples of tissues in humans are: Muscle tissue: makes up muscles, and is composed of myocytes, which are elongated cells containing specialised cytoskeleton. These structures help the cells contract, aiding the function of movement. Nervous tissue: makes up the nervous system, and is composed of neurons, cells which process and pass along information in the form of electrochemical signals. Neurons have specific structural features, including dendrites, an axon, and synapses, to help perform this function. Epithelial tissue: coats surfaces of the body including the digestive tract and skin. It is composed of epithelial cells, which are specialised to perform secretion, excretion, and absorption functions. Some examples of tissues in plants are: Mesophyll tissue: made up of mesophyll cells, containing chloroplasts to perform photosynthetic func- tions. Xylem tissue: made of tracheids, which are elongated and have a thick cell wall, specialised for allowing water flow. K EY P OINT : Organ: a structure which is composed of a number of tissues which work together to perform a shared function. Organs are groups of tissues arranged in an order to perform a large-scale function (contrasted to individual cell function). Organ structure is influenced by the function it must perform. 24 Copyright © 2024 InStudent Publishing Pty. Ltd. 1.2 Cell differentiation and specialisation For example, the stomach digests food, so it has a large internal cavity containing acids, enzymes, and bacteria which break food down. The cavity is surrounded by epithelial cells, so that nutrients can be absorbed. Additionally, the walls of the stomach contain muscle cells, which are able to contract and influence movement. This helps the organ to physically break the food down. Some examples of organs within the human body are: The lungs which contain smooth muscle tissue, epithelial tissue, bronchioles, alveoli, lymphatic tissue and blood tissues. The heart which contains cardiac muscle tissue, connective tissues, blood tissues and nervous tissue. The kidneys which contain connective tissue, adipose (fat) tissue, blood tissues, lymphatic tissue and renal tissue. An example of a plant organ is roots, which are made of vascular tissues, epidermal tissues, and parenchyma tissue (pith). K EY P OINT : System: a group of related organs which work cohesively together to perform an aspect of bodily function. For example, the renal system is composed of the kidneys, as well as the uterus, bladder, and urethra, which work together to clear wastes from the body. The types of organs which compose a system will depend upon the overall function that the system is at- tempting to achieve. For example, the circulatory system needs to pass blood to all cells in the body. To do Section 1 – Organisation of Cells this, it needs some organ to deliver the blood, and some organ to pump the blood through this delivery net- work. Therefore, the circulatory system is made up of the heart, as well as blood vessels (arteries, veins, and capillaries). However, the systems within organisms are not separate, and interact to achieve joint functions. The circulatory system needs to re-oxygenate blood and get rid of gaseous waste, so it is connected to the respiratory system, which introduces oxygen into the blood and exhales CO2. System Function Organs Nervous system To coordinate bodily function by passing Brain, sensory organs (eyes, electrochemical signals, to detect and ears, tongue, skin), nerves respond to external stimuli Digestive system To breakdown and absorb food Stomach, intestines, mouth, liver Renal system To rid the body of metabolic wastes Kidney, bladder, urethra, ureters S YLLABUS : Justify the hierarchical structural organisation of organelles, cells, tissues, organs, systems and organisms. Hierarchical structure of multicellular organisms Hierarchies are systems which are organised in levels, where each level has a certain role, and forms the basis for the next. Hierarchical structures enable systems to have multiple levels of complexity, yet still be governed by one ‘head’ which gives orders. All multicellular organisms, such as humans, are organised in a hierarchical structure. Organelles and sub- cellular structures are the most basic features of an organism, performing specific metabolic functions within cells to sustain life. Copyright © 2024 InStudent Publishing Pty. Ltd. 25 1.2 Cell differentiation and specialisation Cells are the next layer up, enclosing organelles to isolate their functions to specific areas of the body. Groups of homogenous cells make up tissues. Different tissues are arranged to form organs. Organs operate together within an organ system. Finally, all organs systems are controlled by the brain, which coordinates the multicellular organism. Let’s look at a system in the body to demonstrate the benefit of out hierarchical structural organisation, the renal system. As can be seen in the diagram below, each sub-section contributes to the overall function of the system as a whole. However, this diagram is an over-simplification of what actually happens in the body, and in reality, there are so many little elements that contribute to overall function. Due to the hierarchical structure, each layer is more specialised, and allows for a huge amount of complexity within the organism. Section 1 – Organisation of Cells 26 Copyright © 2024 InStudent Publishing Pty. Ltd. Nutrient and Gas Requirements Section 2 Nutrient and Gas Requirements S YLLABUS : Inquiry question: What is the difference between nutrient and gas requirements between autotrophs and heterotrophs? 2.1 Structure of Plants S YLLABUS : Investigate the structure of autotrophs through the examination of a variety of materials, for example: Dissected plant materials Microscopic structures Using a range of imaging technologies to determine plant structure Section 2 – Nutrient and Gas Requirements Plants are composed of two major organ areas; the shoot system (everything above the ground, including the stem, leaves, any buds or flowers, and fruiting structures) and the root system (everything below the ground, including the roots or tubers). There are three main cell types found in plants; the parenchyma, collenchyma, and the sclerenchyma. Parenchyma cells are responsible for photosynthesis, as well as organism repair, and storage of starch. They are found in the leaves, stems, and fruits of plants. Collenchyma cells provide structural support, and are therefore usually found in the epidermis of the stem and leaf. Sclerenchyma are dead cells, which are used to provide structural support. 2.1.1 Leaf The leaves of a plant are vital as they are the site of photosynthesis. This means they are composed of cells responsible for production of glucose and oxy- gen requirements of the organism. As we can see in this microscope image of Elodea leaf cells, there are many green dots. These are the chloroplasts. They are the organelles responsible for producing glucose, as we discussed in Module 1. What we can also clearly see from this microscope image is that each cell has a distinct cell wall. This is essential in plant cells in order to maintain their more rigid structure. On the outer of the leaf there is a layer called the epidermis, which allows for gas exchange into and out of the plant. This layer ranges in thickness depending on the type of plant and its environment. To regulate exchange of gases there are stomata within the epidermis. These are specialised structures which act as pores for the leaf, opening and closing with the aid of guard cells to ensure the right amount of gas enters/leaves the leaf. Coating the epidermis is the cuticle, a waxy layer which protects from excessive loss of water or gas exchange. Below the epidermis there are two layers of mesophyll cells. The palisade mesophyll (also called the palisade parenchyma) is composed of tightly packed, photosynthetic cells. The spongy mesophyll (or spongy parenchyma) is also responsible for photosynthesis, but it’s cells are shaped more irregularly and are loosely packed, which allows them to create air space in the leaf. This enables gas exchange through the stomata below, and in aquatic plants this airy layer gives the plants buoyancy. The leaves also contain vascular bundles, which deliver nutrients and water to cells. Copyright © 2024 InStudent Publishing Pty. Ltd. 27 2.1 Structure of Plants Section 2 – Nutrient and Gas Requirements 2.1.2 Stem The stem provides structural support for the leaves, as well as being the component of the plant which delivers nutrients and water around the plant, via the vascular bundles. Firstly, let’s talk about the vascular bundles, which are composed of two structures. The xylem transports water, and these cells are located towards the centre of the stem. We can see them in the microscope image to the right as the larger cir- cles with a purple stain around them. The phloem is responsible for transporting sugars and nutrients around the cell, see in the microscope image as the small clusters of dark purple cells towards the outer rim of the stem. The xylem and phloem are sep- arated by the cambium. We will explore the xylem and phloem in more detail when we discuss trans- port systems in the next section. The outer layer of the stem is composed of the epidermis, which acts as a protective layer. In some plants, this is accompanied by a woody layer to provide extra support and protection. The tissue surrounding the vascular bundles is known as ground tissue, and is composed of mostly parenchyma cells. Towards the centre of the stem it is called pith, as between the vascular bundles and the epidermis it is called the cortex. 28 Copyright © 2024 InStudent Publishing Pty. Ltd. 2.2 Movement of products of photosynthesis 2.1.3 Root Roots are the structures in plants which provide stability (anchor- ing them into the soil), and are the main source of water and nu- trient collection. Root hairs are located on the very exterior of the root, as elongated parts of the epidermis. They have a very large surface area, able to absorb large amounts of water and nutrients from soil. These substances entre the root hair cells by diffusion and osmosis, and are then delivered to the rest of the plant by the xylem and phloem. The xylem and phloem are located towards the centre of the root. As we can see in the microscope image to the right, the xylem are the larger cells in the centre, and the phloem are the smaller cells surrounding this central complex. Roots contain three sections, the zone of maturation, the zone of elongation, and the meristematic zone. The zone of maturation is where mature cells, in- cluding xylem and phloem cells, are found, providing the basic structure for the root. Section 2 – Nutrient and Gas Requirements The zone of elongation is where new cells are added and begin to elongate. The meristematic zone is where cells are constantly undergoing mitosis, and diving, which creates new cells for the root to grow. 2.2 Movement of products of photosynthesis S YLLABUS : Investigate the function of structures in a plant, including but not limited to: Tracing the development and movement of the products of photosynthesis Let’s recall the equation for photosynthesis: K EY P OINT : Photosynthesis: Carbon dioxide + Water + Light −→ Glucose + Oxygen We know that the products for photosynthesis are glucose and oxygen, and that these are produced in the chloroplasts of photosynthetic cells, but where do they go from there? Firstly, consider the function of these molecules. Glucose is an energy molecule which cells breakdown, obtaining ATP energy by breaking molecular bonds. We know that all cells need energy, so the glucose which photosynthetic cells produce will be transported to cells throughout the plant. Glucose is transported by the phloem in the vascular bundle, which runs from the leaves and throughout the plant, including the stem and roots. Substances are transported in the phloem by a method celled translocation. This method is supported by the pressure-flow theory: Nutrients are moved into the phloem by active transport at the ‘source’ (photosynthetic cells) Water follows by osmosis, creating a pressure gradient Nutrients move passively down phloem, following the pressure gradient Sugars are actively transported out of the phloem at the ‘sink’ (cells which require glucose/nutrients) Copyright © 2024 InStudent Publishing Pty. Ltd. 29 2.3 Microscopic gas exchange structures Once glucose has been transported around the plant is it used, either in aerobic respiration to produce energy, or to produce cellulose for the cell wall, or as a building block of cell proteins. Glucose is also stored in plants, for later use when light isn’t available for continuous photosynthesis. It can be stored as starch, either in small starch plastid packages within cells or in tuber structures. It can also be stored as plant fats and oils. Oxygen is also essential for cellular respiration, so is used by respiring cells within the plant. However, during daylight hours, the rate of photosynthesis is often greater than the rate of respiration, and so excess oxygen is produced. This is released by the plant through stomata in the leaves, by a process called gas exchange. We will investigate this process further in the next section. 2.3 Microscopic gas exchange structures S YLLABUS : Investigate the gas exchange structures in animals and plants through collection of primary and secondary data and information, for example: Microscopic structure: alveoli in mammals and leaf structure in plants Macroscopic structures: respiratory systems in a range of animals 2.3.1 Mammals: alveoli Section 2 – Nutrient and Gas Requirements Blood is the medium of transport within mammals, responsible for carrying nutrients and gases to the cells, and wastes away from the cells. In order to perform aerobic respiration, oxygen is transported into the blood in the lungs, and then carried to the cells. The by-product of aerobic respiration, CO2 , is then diffused into the blood, and this is carried back to the lungs to be exhaled. The surface across which the exchange of oxygen into the blood, and CO2 out of the blood, is performed are membranes of the alveoli. The alveoli are clusters of air sacs, which air flows into from the bronchioles of the lungs. A single air sac is called an alveolus. Blood capillaries are wound around the alveoli. Where their thin membranes touch, gas is able to diffuse based upon concentrations on either side of the membranes. When deoxygenated blood travels from cells to the lungs carrying CO2 , the CO2 will diffuse into the alveoli as there is a high concen- tration of CO2 in the blood and a low concentration in the lungs. It diffuses passively along the concentration gradient. Likewise, O2 will diffuse into the blood, be- cause there is a high concentration in the lungs and a low concentration in the blood. The efficiency of this gas exchange is ensured by a number of structural features. The alveoli have a large surface area, approximately 90 m2 , across which gas can diffuse. The walls of the alveoli are very thin (similar to capillaries, they are only a single cell thick), so gases can flow more easily between spaces. The alveoli are also covered in many capillaries, ensuring a large blood supply for gases to be exchanged with. Additionally, the surface of alveoli are kept moist. This enables oxygen and carbon dioxide to more easily diffuse, as they do so more rapidly when dissolved in water. 30 Copyright © 2024 InStudent Publishing Pty. Ltd. 2.4 Macroscopic Gas Exchange Structures 2.3.2 Plants: leaf structure Stomata are structures essential for gas exchange in plants. During gas exchange, the guard cells of the stoma become swollen and turgid, opening the pore. Oxygen will diffuse out of the stoma, and CO2 will diffuse into the stoma, along the concentration gra- dient. Along with oxygen, water in the plant will also transpire out through the stoma. In dry conditions, when there is less water available, the guard cells of the stoma will not become turgid, so less gas ex- change will occur. Light, wind, and humidity are fac- tors which can also effect the rate of gas exchange. 2.4 Macroscopic Gas Exchange Structures 2.4.1 Mammals: lungs Section 2 – Nutrient and Gas Requirements The lungs are the main organ of the respiratory system in mammals. Th