auditory system LA ho.pdf
Document Details
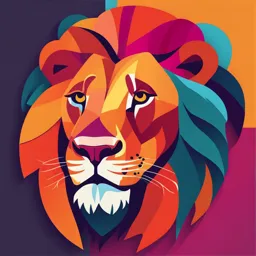
Uploaded by AchievableYew
Tags
Full Transcript
The Auditory System Laura Andreae The auditory system The ear transduces sounds into neural signals which are then processed by the brain. Sound is produced by vibrations => pressure wave that radiates out from source Cycles per sec = frequency in Hz (=> pitch) Human ear can detect range of frequen...
The Auditory System Laura Andreae The auditory system The ear transduces sounds into neural signals which are then processed by the brain. Sound is produced by vibrations => pressure wave that radiates out from source Cycles per sec = frequency in Hz (=> pitch) Human ear can detect range of frequencies from 20 – 20,000 Hz Pressure amplitude (=> loudness) measured in decibels (dB) – log scale Sound pressures > 100dB can damage sensory apparatus of ear The auditory system: overview External ear Middle ear Inner ear External ear helix tragus lobule External ear Elastic fibrocartilaginous structure Main features are helix, lobule and tragus Helps collect sound waves and discriminate direction of sound External auditory meatus Inner 2/3rd temporal bone Outer 1/3rd cartilaginous S-shaped curve, ~ 2.5 cm long, leading to tympanic membrane or ear drum which is concave and lies at an oblique angle Meatus and tympanic membrane are supplied by sensory fibres from vagus and trigeminal Auroscopic view of tympanic membrane The middle ear Sounds cause the tympanic membrane to vibrate – this vibration is then conveyed through the middle ear (air filled) by a series of 3 small bones – ossicles The tympanic membrane and ossicles allow sounds to be transmitted efficiently from air to fluid-filled cochlea. Area of tympanic membrane > area of oval window => Increased pressure at oval window Middle ear communicates with pharynx via pharyngotympanic tube (= Eustachian tube) - opened by swallowing and yawning - opens into nasopharynx at level of inferior concha The cochlea Cochlea – part of bony labyrinth. Inside is cochlear duct (part of membranous labyrinth). doesn’t look like this does look like this The cochlea scala vestibuli scala tympani scala vestibuli helicotrema scala tympani basilar membrane scala media The cochlea Scanning EM of guinea-pig cochlea The organ of Corti OHC IHC Basilar membrane Scala tympani Sensory receptors of auditory system: inner hair cells stereocilia Sensory receptors of auditory system: inner hair cells Frequency coding Basilar membrane Narrow and stiff at base, near oval window Wide and flexible near apex Frequency coding stiff flexible scala vestibuli scala tympani basilar membrane scala media Frequency coding Basilar membrane Narrow and stiff at base, near oval window Wide and flexible near apex Sound => Peak amplitude of wave depends on the frequency of sound: at low freq, peak amp is near apex; at high freq, peak amp is near base. Hair cells situated at point where oscillations are maximal are most excited. => tonotopy, or place code Outer hair cells Outer hair cells amplify BM motion and enhance frequency sensitivity Outer hair cells respond to electrical stimulation by changing their length – unique property, due to special motor protein ‘prestin’. prestin Outer hair cells amplify BM motion and enhance frequency sensitivity Outer hair cells respond to electrical stimulation by changing their length – ‘electromotility’ – unique property, due to special motor protein ‘prestin’. Response is voltage dependent: depolarization => contraction, hyperpolarization => elongation Outer hair cells amplify BM motion and enhance frequency sensitivity Outer hair cells respond to electrical stimulation by changing their length – unique property, due to special motor protein ‘prestin’. Response is voltage dependent: depolarization => contraction, hyperpolarization => elongation In vivo, generate receptor potentials in response to sound OHC motility is thought to contribute to basilar membrane motility and thereby amplify signal, esp weak ones OHCs also emit sounds – otoacoustic emissions. These propagate in reverse, back through middle ear to move tympanic membrane. These are useful to clinicians as hearing tests, especially for infants Afferent fibres and amplitude coding Outer hair cells Inner hair cells Voltage-gated calcium channels sense changes in membrane voltage and adjust rate of glutamate release from inner hair cells so afferent AP firing rate reflects amplitude of BM deflection AP firing rate codes for amplitude of sound (loudness) Anatomical arrangement of auditory nerve fibres follows that of the BM, preserving tonotopy Spiral ganglion Spiral ganglion Central connections trigeminal nerve (cV) trochlear nerve (cIV) facial nerve (cVII) pons cerebellum abducens nerve (cVI) vestibulocochlear nerve (cVIII) cVIII joins the brain stem in the cerebellopontine angle Central connections Cochlear nuclei Vestibular nuclei Inferior cerebellar peduncle CN VIII olive Central connections Dorsal cochlear nucleus Ventral cochlear nucleus Low freq => ventrolateral parts of both nuclei High freq => dorsal parts of both nuclei Tonotopic organisation preserved Central connections Central connections Tonotopic frequency maps exist in cochlear nuclei, superior olivary nucleus, inferior colliculus, the ventral division of medial geniculate body and some parts of auditory cortex Associated with the auditory cortex on the left side of the brain are Wernicke’s speech sensory area and Broca’s speech motor area. Bilateral connectivity allow distance and directional sensitivity Tectospinal connections from inferior colliculus (via superior colliculus) control auditory reflexes (e.g. turning head towards sound) Superior olivary nucleus is source of efferent fibres that project back to the cochlear hair cells……..feedback inhibition to refine sound perception COMMON CAUSES OF HEARING LOSS 1. CONDUCTIVE DEAFNESS Earwax, damage to ear-drum, otosclerosis of the middle ear, trauma, middle ear infections, genetic defects 2. SENSORINEURAL DEAFNESS Cochlea – infection, trauma, noise, age, ototoxic drugs, genetic defects (myosins, gap junction mutations etc), tumours. Cochlear implants COMMON CAUSES OF HEARING LOSS 1. CONDUCTIVE DEAFNESS Earwax, damage to ear-drum, otosclerosis of the middle ear, trauma, middle ear infections, genetic defects 2. SENSORINEURAL DEAFNESS Cochlea – infection, trauma, noise, age, ototoxic drugs, genetic defects (myosins, gap junction mutations etc), tumours. 3. CENTRAL DEAFNESS Vascular accident, trauma, MS, infection, tumour, neonatal distress COMMON CAUSES OF HEARING LOSS 1. CONDUCTIVE DEAFNESS Earwax, damage to ear-drum, otosclerosis of the middle ear, trauma, middle ear infections, genetic defects 2. SENSORINEURAL DEAFNESS Cochlea – infection, trauma, noise, age, ototoxic drugs, genetic defects (myosins, gap junction mutations etc), tumours. 3. CENTRAL DEAFNESS Vascular accident, trauma, MS, infection, tumour, neonatal distress TINNITUS This is a constant sensation of tone(s) – high pitch, low pitch, buzzing, etc which can persist for many years. It is caused by spontaneous activity in hair cells, spiral ganglion cells or neurons of the CNS. It can indicate damage to hair cells (e.g after excessive noise), but is often of unknown aetiology, and is difficult to treat. Suggested reading The Head and Neck: Dean and Pegington Fundamental Neuroscience: Haines Fundamental Neuroscience: Squire et al Principles of Neural Science: Kandel and Schwartz and if you’re really keen (for interest only) - ‘Auditory Neuroscience’: Schnupp et al.