Epstein-Barr Virus-Associated Gastric Cancer: A Distinct Subtype (2020) PDF
Document Details
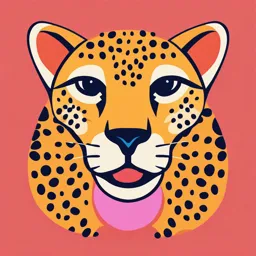
Uploaded by SensibleRadium
The First Affiliated Hospital of South China
2020
Jing Yang, Zhifeng Liu, Bin Zeng, Guangsheng Hu, Runliang Gan
Tags
Summary
This is a review article on Epstein-Barr virus-associated gastric cancer (EBVaGC). It discusses the molecular features, clinicopathological characteristics, and the role of the Epstein-Barr virus (EBV) genome in the development of this cancer subtype. The review highlights EBVaGC as a distinct subtype compared to other gastric cancers.
Full Transcript
Cancer Letters 495 (2020) 191–199 Contents lists available at ScienceDirect Cancer Letters journal homepage: www.elsevier.com/locate/canlet Epstein–Barr virus-associated gastric cancer: A distinct subtype Jing Yang a, b, 1, Zhifeng Liu c, 1, Bin Zeng a, Guangsheng Hu a, Runliang Gan b, * a Departmen...
Cancer Letters 495 (2020) 191–199 Contents lists available at ScienceDirect Cancer Letters journal homepage: www.elsevier.com/locate/canlet Epstein–Barr virus-associated gastric cancer: A distinct subtype Jing Yang a, b, 1, Zhifeng Liu c, 1, Bin Zeng a, Guangsheng Hu a, Runliang Gan b, * a Department of Gastroenterology, The First Affiliated Hospital of University of South China, Hengyang, 421001, Hunan Province, China Cancer Research Institute, Hunan Province Key Laboratory of Tumor Cellular & Molecular Pathology, University of South China, Hunan, 421001, China c Department of Otorhinolaryngology, The First Affiliated Hospital of University of South China, Hengyang, 421001, Hunan Province, China b A R T I C L E I N F O A B S T R A C T Keywords: Gastric cancer Epstein-barr virus (EBV) Molecular features Clinicopathological features Epstein–Barr virus (EBV)-associated gastric cancer (EBVaGC) is a common malignant tumor associated with EBV infection. The molecular classification of gastric carcinoma indicates that EBVaGC is a distinct subtype in terms of oncogenesis and molecular features. Viral proteins, Bam–HI–A rightward transcripts (BART) miRNAs, and Bam-HI A rightward frame 1 (BARF1) promote oncogenesis after EBV infection via the induction of methylation, regulation of host gene expression, and malignant transformation. Together with abnormal mutations and amplification of the host genome as driving factors, interactions between the EBV genome and host genome accelerate carcinogenesis. The molecular profile of EBVaGC is that of EBV driving DNA hypermethylation, frequent phosphatidylinositol-4,5-bisphosphate 3-kinase, catalytic subunit alpha (PIK3CA) mutations, and the overexpression of Janus kinase 2 (JAK2), programmed death ligand-1 (PD-L1), and PD-L2. Clinically, the fre quency of lymph node metastasis is lower, and the prognosis is better for EBVaGC than EBV-negative gastric cancer (EBVnGC). Pathologically, EBVaGC is a gastric adenocarcinoma with lymphoid stroma. This review in terprets how the EBV genome is involved in the oncogenesis of gastric cancer and describes the molecular and clinicopathological features of EBVaGC. 1. Introduction Gastric cancers are responsible for ~780,000 deaths annually, making them the third-most prevalent type of cancer mortality globally. Epstein–Barr virus-associated gastric cancer (EBVaGC) is a common malignancy associated with EBV infection. The EBVaGC comprises about 1.3%–30.9% of all gastric cancers according to geographic dis tribution [3–6], with a global average of 8.9% of all gastric cancers accounting for about 75,000 new diagnoses annually. What drives mutations and the molecular characteristics of GC have been under investigation. Epstein-Barr virus (EBV)-positive GC has the CpG island methylator phenotype [7,8]. High-throughput sequencing has shown that EBV-positive gastric cancer has distinct mutation pat terns [9,10]. Gastric cancers are generally divided into the following molecular subtypes: EBV-positive, microsatellite instability (MSI), genomic stability (GS), and chromosomal instability (CIN). Mo lecular classification evidence from patients with EBVaGC has indicated that EBVaGC is a distinct subtype in terms of oncogenesis. We reviewed EBV infection, roles of the EBV genome in oncogenesis, molecular pro file, and clinicopathological features of EBVaGC, highlighting the involvement of the EBV genome in gastric carcinogenesis. 2. Definition Burke et al. (1990) initially identified EBVaGC as EBV-infected tumor cells using polymerase chain reaction (PCR) analysis of paraffin-embedded samples of undifferentiated lymphoepithelial gastric carcinoma. Today, EBV-negative GC (EBVnGC) and EBVaGC in Abbreviations: BARF1, BamHI-A rightward frame 1; BARTs, BamHI-A rightward transcripts; BHRF1, BamHI fragment H rightward open reading frame 1; BCOR, BCL6 co-repressor; CIN, chromosomal instability; DLBCL, diffuse large B cell lymphoma; EBER, EBV-encoded small RNA; EBER1/2-ISH, EBV-encoded small RNA 1/2 in situ hybridization; EBNA1, EBV nuclear antigens 1; EBV, Epstein-Barr virus; EBVaGC, Epstein–Barr virus-associated gastric cancer; EBVnGC, Epstein–Barr negative gastric cancer; GS, genomic stability; JAK2, Janus kinase 2; LMP, latent membrane protein; MSI, microsatellite instability; NPC, nasopharyngeal carcinoma; PD-L1, programmed death ligand 1; PIK3CA, phosphatidylinositol-4,5-bisphosphate 3-kinase, catalytic subunit alpha; TCGA, The Cancer Genome Atlas. * Corresponding author: Cancer Research Institute, Hunan Province Key Laboratory of Tumor Cellular & Molecular Pathology, University of South China, Hunan, 421001, China. E-mail address: [email protected] (R. Gan). 1 These authors contributed equally to this work. https://doi.org/10.1016/j.canlet.2020.09.019 Received 23 June 2020; Received in revised form 28 August 2020; Accepted 21 September 2020 Available online 23 September 2020 0304-3835/© 2020 The Authors. Published by Elsevier B.V. This is an open (http://creativecommons.org/licenses/by-nc-nd/4.0/). access article under the CC BY-NC-ND license J. Yang et al. Cancer Letters 495 (2020) 191–199 histopathological specimens are usually distinguished using EBV-encoded small RNA 1/2 in situ hybridizations (EBER1/2-ISH). Moreover, a new method of droplet digital PCR diagnosing (ddPCR) can detect EBVaGC in tissue samples by calculating the copy numbers of EBV-DNA. the absence of autonomous replication. The EBV genome does not integrate into the host genome and is maintained as plasmids called episomes. Notably, EBER-ISH is positive in all infected gastric carcinoma cells and adjacent dysplastic epithelium, but, negative in surrounding normal epithelial cells and lymphocytes, indicating that EBV infection occurs before transformation. The uniform terminal fragment of EBV nuclear antigens 1 (EBNA1) expressed by GCs suggests that EBVaGC results from the monoclonal proliferation of EBV-infected cells. Depending on the physiological and pathological status of the host, EBV expresses latency 0, I, II, and III genes. Fig. 1 shows that these la tency types differ among diverse EBV-associated malignancies. 3. EBV infection and oncogenesis Double-stranded DNA EBV isolated from a Burkitt lymphoma cell line was the first virus to be associated with human malignancy in 1964. Today, EBV is a confirmed pathogen associated with a broad spectrum of multiple human malignancies including Burkitt, Hodgkin and NK/T-cell lymphomas, nasopharyngeal carcinoma (NPC), leio myosarcoma, and gastric carcinoma [16,17]. A prospective study of 2, 760 patients with GC including 140 with EBV-positive GC validated by EBER-ISH found that plasma EBV-DNA load was a good marker of recurrence and chemosensitivity. The plasma EBV-DNA load in patients with EBVaGC decreases after gastrectomy and chemotherapy, and increases during disease progression. These findings suggested the etiological involvement of EBV in gastric oncogenesis. 3.2. EBV latent genes in EBVaGC Epstein-Barr virus is a direct transforming pathogen through its regulation of host gene expression and cell cycle pathways. The roles of EBV in oncogenesis involve the expression of its own genes and regulation of the host genome. Viral gene expression by EBVaGC is closer to latency I and limited to EBV-encoded small RNA (EBER), EBNA1, and BamHI-A rightward transcripts (BARTs) including micro RNA from BARTs. However, recent findings suggest that the viral latent profile of EBVaGC corresponds to latency I or II; in the latter, latent membrane protein (LMP)-1, 2A, and 2B, BamHI-A rightward transcripts 1(BARF1) are also expressed. Fig. 1 shows this inter mediate state of EBVaGC between latency I and II. The abundance of EBER is proportional to that of EBV DNA genomes, and thus is the gold standard for detecting EBVaGC. Datasets of RNA sequences have been re-analyzed to quantify EBV gene expression in a cohort of gastric carcinomas in TCGA. That study classified most EBV positive samples as latency I, and a minority as latency II. Most of the EBV reads were BARTs. Latent membrane protein 2A, and to a lesser extent, LMP1 were expressed. This variety might have been due to sample size and technology. Today, investigators agree that the latent gene expression of EBVaGC is limited to EBER, EBNA1, and BARTs with most having no or low LMP1 and LMP 2B expression, and LMP2A is expressed in about 40% of pa tients with EBVaGC [26–28]. Fig. 2 shows the levels of relevant latency gene expression by EBV in GC, which are discussed separately in the following sections. 3.1. EBV infection EBV (also known as human herpesvirus 4) infects over 90% of the adult population, mostly with a lifelong asymptomatic state. Rates of infection due to free EBV in saliva entering gastric epithelial cells are extremely low. However, precisely how EBV infects epithelial cells has remained unclear. Direct cell-to-cell contact mediated by B lym phocytes is the predominant way of EBV entry into epithelial cells. Infection with EBV starts in the oropharynx where it targets oral mucosal epithelium and oropharyngeal B cells during primary infection. The oropharyngeal mucosa is heavily infiltrated with lymphocytes, and cell-free virus in saliva binds firstly to the cell membrane of B lympho cytes, then to oropharyngeal epithelial cells. The efficiency of EBV infection is 800-fold higher in epithelial cells co-cultured with EBV-positive B cells than in cell-free infection. After persistent infection of oral epithelial cells, EBV selectively be comes a latency that is a carrier state, but not lytic. The viral genome replicates simultaneously with that of the host cell in latency, in Fig. 1. The spectrum of EBV latency gene expression. Epstein-Barr virus latency is classified as 0, I, II, and III, according to profiles of latent gene expression [24,25]. Latency genes of Epstein-Barr virus-associated gastric cancer (EBVaGC) include EBER1, EBER2, EBNA1, BART miRNAs, and BARF1, most of which lack or express low levels of latent membrane proteins (LMP) 1 and LMP 2B. LMP2A is expressed in about 40% of patients with EBVaGC [26–28]. DLBCL, diffuse large B cell lymphoma; PTLD, post-transplant lymphoproliferative disease. 192 J. Yang et al. Cancer Letters 495 (2020) 191–199. Primary gastric epithelial cells infected with recombinant EBV showed a disordered cell cycling phenotype with restricted expression of a set of viral latent genes, which was similar to EBV-positive gastric carcinoma biopsy specimens without EBNA2 and LMP1. These findings indicated that LMP1 is probably not a key oncogenic protein in EBVaGC. 3.2.4. LMP2A Latent membrane protein 2A (LMP2A) is expressed in 40% of pa tients with EBVaGC in contrast to the low levels of LMP1 and 2B expression [26,27]. The carcinogenic effects of LMP2A in EBVaGC are controversial. The LMP2A-induced upregulation of survivin that results in resistance to serum free-induced apoptosis in the EBV-MKN-1 cell line, can be blocked using an NF-κB inhibitor, indicating that LMP2A can resist apoptosis and progress via survivin. Latent membrane protein 2A represses the expression of aquaporin (AQP) 3, which played crucial roles in cancer progression and metastasis in vitro. In mechanistic terms, LMP2A induces the phosphorylation of ERK, which activates DNMT3a transcription and downregulates AQP3 through methylating CpG islands in the AQP3 promoter of the host genome. Lentivirus-mediated RNAi knockdown of LMP2A inhibits the growth and colony formation of the EBV-positive GC cell line GT38 and arrests the cell cycle at the G0/G1 phase. However, Seo et al. established a gastric adenocarcinoma cell line (AGS) expressing detectable LMP2A protein using a retroviral vector. Colony-forming assays in soft agar showed that the proliferation of clones of this cell line was not enhanced compared with control AGS cells. Such divergence requires more comprehensive investigation in vivo and indicated that LMP2A targeted therapy might not apply to all patients with EBVaGC. Fig. 2. Profiles of EBV viral gene transcription expression in Epstein-Barr virusassociated gastric carcinoma. Red shows EBV latent gene transcription expressed in EBVaGC. Gray bar shows relative expression levels of corre sponding genes. This figure was drawn based on the original data [26–28]. (For interpretation of the references to colour in this figure legend, the reader is referred to the Web version of this article.) 3.2.1. EBER The non-coding small RNA EBER1 and EBER2 comprise 167 and 173 nucleotides, respectively that are transcribed by EBV using RNA poly merase III. Although they are abundant in latent EBVaGC, their roles in gastric carcinogenesis remain unknown. Transfecting EBER into the EBV-negative GC cell line NU-GC-3 induces the expression of insulin-like growth factor 1 (IGF-1), which serves as an autocrine growth factor to promote cell proliferation in gastric carcinoma cells. This effect can be blocked by anti-IGF-1 antibodies. Besides, EBER can upre gulate the expression of IL-6 and downstream STAT3 to downregulate the cell cycle inhibitors, p21, and p27, which are associated with che moresistance in vitro. Moreover, EBER promotes the migration phenotype in vitro by activating pro-metastatic pFAK and pPAK1, and downregulating anti-metastatic RhoGD1 and KAI-1. 3.2.5. BARTs The major miRNA clusters, BamHI-A rightward transcripts (BARTs) and BamHI fragment H rightward open reading frame 1 (BHRF1) , which comprises 25 EBV miRNA precursors and 44 EBV mature miRNA in EBV are documented in the miRBase (http://www.mirbase.org/). The BARTs cluster, consisting of 22 miRNA precursors and 40 mature miRNA from the BamHI-A region, are expressed in all EBV-infected hosts [46, 47]. The BHRF1 cluster, including 3 miRNA precursors and 4 mature miRNA, are expressed in lytic infected hosts and latency III hosts. The BARTs are multi-spliced rightward, 150,000–161,000 nt tran scripts of BamHI A fragments of the EBV genome. Several of these transcripts with predicted open reading frames, such as BARF0, RPMS1, and A73, probably encode proteins, but these have not been confirmed in EBVaGC samples. Evidence of BARTs expression in EBVaGC consists of BARF1 and 40 mature BART miRNAs that have been vali dated in EBVaGC tissue samples by quantitative RT-PCR, but evidence of the BHRF1 cluster is nonexistent [47,49]. 3.2.2. EBNA1 EBNA1 is the only protein encoding gene involved in all latent types of EBV-associated malignancies. Although important for the replication and stabilizing of EBV episomes, the expression of EBNA1 in EBVaGC keeps very low levels, sometimes even absent. By competing with p53 for ubiquitin-specific protease 7, which degrades p53 protein to lose its function by ubiquitination, EBNA1 can promote EBV-mediated host cell growth and transformation in vitro. In addition, EBNA1 expressed in LL/2 cells enhances tumorigenicity in immunocompetent allograft Balb/c mice according to xenograft tests. Furthermore, EBNA1 contributes to the epigenetic deregulation of gastrokines (GKN) 1 and 2, which are specific tumor suppressor genes in GC. EBNA1 directly combines with the divergent promoter of the GKN1 and 2 genes such that both GKN are transcriptionally repressed by DNA methylation in vitro. However, considering the low level of EBNA-1 expression in EBVaGC, its significance in oncogenesis and as a therapeutic target re quires more supportive high-throughput sequencing data. 3.2.5.1. BART miRNAs. High levels of BART miRNAs expression and absent BHRF1 clusters have been confirmed in EBVaGC cell lines and patients [47,48,50]. Shinozaki et al. profiled the expression of 44 known EBV miRNA in human tissues and EBVaGC cell lines, and analyzed their target genes in silico. Their bioinformatics analysis uncovered various potential target genes, including those involved in oncogenesis, tumor suppression, adhesion-associated pathways, and apoptosis, such as the Bcl-2 family. They validated the antiapoptotic role of EBV-miR-BART4-5p via the downregulation of Bid expression in EBV-positive cell lines. Epstein-Barr viral miR-BART5-3p (BART5) can promote the oncogenesis of GC by directly targeting the tumor suppressor gene TP53, mutations of which are rare in EBVaGC tissues. Mechanistically, BART5 directly targets the 3′ -UTR of TP53, downregulates TP53 expression and consequently decreases CDKN1A, BAX, and FAS expression, which leads to apoptosis resistance after chemotherapeutic drug and ionizing radiation in vitro. Not only do EBV miRNAs interfere with host genome expression but they also inhibit 3.2.3. LMP 1 The latent membrane proteins (LMP) 1, 2A, and 2B, are located in the host cell membrane. The LMP1 gene encodes a viral protein that is expressed in Hodgkin lymphomas, nasopharyngeal carcinoma, and GC. Investigators agree that LMP1 is expressed at low levels in EBVaGC [34–37]. One systematic review found that LMP1 was expressed in only 10% of patients with EBVaGC. This might be explained by methylation of the LMP1 promoter. Li et al. determined the methylation profiles of promoters of LMP1, LMP2A, and LMP2B in 41 EBVaGC samples and 5 EBV-positive cell lines by methylation-specific PCR and bisulfite sequencing. They concluded that all LMP promoters were methylated to different degrees and that LMP1 expression could be recovered using a demethylation agent. From a mainstream perspective, LMP1 pro motes oncogenesis by activating survival and growth pathways in Hodgkin lymphomas and NPC. However, LMP1 enhances apoptosis in gastric carcinoma cells, unlike the growth enhancement in NPC cells 193 J. Yang et al. Cancer Letters 495 (2020) 191–199 Fig. 3. Roles of EBV and host genomes in the oncogenesis of GC. The EBV enters gastric epithelial cells mainly via cell-to-cell contact with EBV-infected B lym phocytes. The EBV genome, as an episome, is transcribed to include EBERs, EBNA1, LMP1, LMP2A, BART miRNAs, and BARF1, and some are translated into proteins after a persistent latent infection. Abnormal host genetic mutations and amplifications are oncogenic driving factors. Some viral proteins, such as EBNA1 and LMP2A, promote oncogenesis by the CpG island methylation of promoters of host tumor suppressor genes, some of which induce malignant transforming represented by BARF1. Interactions between EBV and host genomes accelerate carcinogenesis, which should highlight the regulatory role of BART miRNAs targeting the 3′ UTR of host mRNA transcription. Immune system evasion by PD-L1 and PD-L2 amplification shields carcinogenic transformation. All of these factors ultimately dictate the fate of oncogenesis. the expression of self-viral antigens, such as LMP1 and LMP2A, thereby interfering with antigen presentation and immune cell activation that enables host cells to escape immune recognition. Given that BART miRNAs constitute 15% of total miRNAs in EBVaGC cell lines , details of the regulatory mechanisms of BART miRNAs require further investigation for targeted therapy. 3.2.5.2. BARF1. The BARF1 open reading frame is a 40-kb fragment of BamHI-A rightward transcription of the EBV genome. BARF1 encoding a 31–33 kDa protein is expressed in the cytoplasm and the cell membrane , from which it is mainly secreted [54,55], then it activates the cell cycle as a growth factor. Unlike the EBV tumor-associated latency gene, BARF1 is regarded as an early lytic gene, as it induces the lytic cycle in Burkitt lymphoma cell lines, but is expressed during latency in 194 J. Yang et al. Cancer Letters 495 (2020) 191–199 EBVaGC. High levels of BARF1 transcripts are expressed in EBVaGC tissue samples and cell lines. Although evidence in vivo is insuffi cient, BARF1 functions as a viral transforming gene in EBVaGC cell lines [57–59], since it could immortalize a primary monkey kidney epithelial cell line and mouse fibroblast lines. BARF1 can cause GC cells to resist apoptosis induced by chemotherapy, by indirectly increasing Bcl-2, and decreasing Bax in BGC823. BARF1 upregulates the NF-κB/cyclin D1 pathway to promote the proliferation of gastric carci noma cells, and reduces the cell cycle inhibitor p21 in vitro. Turrini et al. generated a BARF1-specific monoclonal antibody with antitumor potential that reduced tumor masses and improved the long-term sur vival of SCID mice engrafted with NPC and lymphoma cell lines. This was explained as mediating complement-dependent and antibody-dependent cell-mediated cytotoxicity against BARF1 positive tumor cells in vitro. These findings indicate that BARF1 could serve as a therapeutic target in EBVaGC. However, BARF1 protein expression in EBVaGC tissues, and whether EBV-specific oncogenes lead to gastric carcinogenesis both require further investigation. Table 1 lists the EBV latent genes related to GC oncogenesis. Based on analyses of whole-genome sequence data of EBVaGC in the TCGA, EBVaGC has a specific expression profile of latent genes combined with a subset of lytic genes of which neither reflect typical lytic or abortive lytic infection, suggesting a unique role of the EBV genome in oncogenesis in EBVaGC. 4.1. Extreme DNA hypermethylation The hypermethylation of DNA is more extreme in EBVaGC than in any other type of cancer [8,11]. Unsupervised CpG methylation clus tering in unpaired tumor samples showed that EBVaGC significantly correlated with the CpG island methylator phenotype (CIMP), unlike other GC subtypes [9,11]. EBVaGC has the cell cycle-related gene CDKN2A (p16INK4A), p14ARF promoter hypermethylation, and absent MLH1 hyper methylation that characterizes EBVaGC and distinguishes it from the MSI subtype [66,67]. In the TCGA context, RCOR2 gene hyper methylation is silent in 100% and 0% of EBV-positive and-negative GCs, respectively. Other than downregulated CDKN2A, specific genes with epigenomic regulation that reportedly differ from EBVnGC comprise E-cadherin, and p73. Infection with EBV induces the hypermethylation of both host and viral genomes, which regulate cellular functions to facilitate immune evasion and viral persistence. Zhao identified 886 genes with aberrant CpG hypermethylation induced by EBV infection in EBVaGC cell line compared with EBVnGC cell line. The novel hypermethylated and downregulated candidates were IL15RA, REC8, SSTR1, EPHB6, MDGA2, and SCARF2. Mechanistically, LMP2A upregulates DNA methyltransferase-3b (DNMT3b) to induce the promoter CpG island methylation of related genes. Epigenetic regulation occurs not only throughout the host cell genome but also within the EBV genome in EBVaGC. The type of EBV latency is regulated by different DNA methylation combinations of the EBV W, C, and Q promoters. Genomic, transcriptomic, and epigenomic comparisons of EBVaGC vs. EBVnGC cell lines and tissue samples have shown that EBV infection leads to genome-wide abnormal methylation of promoter CpG islands, which directly silences the transcription of genes involved in carcino genesis. Some viral proteins such as EBNA1 and LMP2A, might trigger hypermethylation by inducing DNA methyltransferase over expression. Silencing self-viral latent gene expression through hypermethylation is a strategy to escape host immune recognition and responses. Phase I study of 5-azacitidine prior to chemotherapy showed it would reactivate tumor suppressor genes via DNA demethy lation to augment chemotherapy efficacy for patients with resectable gastric adenocarcinoma. 4. Molecular features The genetic and epigenetic tumorigenic profiles of EBVaGC are quite different from that of conventional gastric adenocarcinoma. Global array analyses and high-throughput sequencing studies are underway to classify GCs at the molecular level. Matsusaka et al. (2011) classified GCs into subgroups based on EBV (− )/low, EBV(− )/high, and EBV(+)/high promoter methylation status. Lei et al. (2013) identified proliferative, metabolic, and mesen chymal gastric molecular subtypes with different responses to PI3-kinase inhibitors and 5-fluorouracil. The Cancer Genome Atlas Network (TCGA; 2014) used six genomic and molecular platforms to comprehensively characterize 295 tumors and categorized GC tumor types as being EBV-positive, or having microsatellite instability (MSI), genomic stability (GS), or chromosomal instability (CIN). However, the clinical application of TCGA molecular classification is limited. The Asian Cancer Research Group (ACRG; 2015) identified the following clinically-relevant molecular subtypes based on disease prognosis as MSI, microsatellite stable/epithelial-to-mesenchymal transition (MSS/EMT), MSS/TP53þ and MSS/TP53¡. The ACRG study found that the overall survival (OS) of EBVaGC was second-best, and more frequent in the MSS/TP53+ subgroup. Integrated molecular classi fications have indicated that EBVaGC is a unique type of GCs. 4.2. Somatic genomic abnormalities The mutation profile of EBVaGC is complicated and diverse. The signature genes are discussed below. 4.2.1. PIK3CA mutations The frequency of representative phosphatidylinositol-4,5bisphosphate 3-kinase, catalytic subunit alpha (PIK3CA) mutations in EBVaGC is 40%–80%, in contrast to 3%–42% of other GC subtypes [6, 68]. Furthermore, recurrent PIK3CA mutations are more dispersed in EBVaGC than in EBVnGC where they are mainly localized in the kinase Table 1 EBV latent genes and their roles in EBVaGC oncogenesis. Latent gene Downstream molecules/pathways Biological function Reference EBER Insulin-like growth factor 1 IL-6-STAT3-p21/p27 pFAK and pPAK1, RhoGD1 and KAI-1 Competes with p53 for binding to ubiquitin-specific protease 7 Promoters of the GKN1 and GKN2 NF-κB/survivin ERK-DNMT3a- AQP3 EBV-miR-BART4-5p/Bid EBV-miR-BART5-3p/TP53 Increased Bcl-2/Bax NF-κB/cyclin D1 Autocrine growth factor Induce chemoresistance Promote cell migration Induce chemoresistance DNA methylation of gastrokine tumor suppressor gene Resists apoptosis DNA hypermethylation of AQP3 to promote progression and metastasis Resists apoptosis Promote oncogenesis Resists apoptosis Promotes proliferation EBNA1 LMP2A BART miRNAs BARF1 195 J. Yang et al. Cancer Letters 495 (2020) 191–199 domain (exon 20). Intratumoral heterogeneity of three to five PIK3CA genotypes (including wild type) coexists in the same primary tumor in 14% of EBVaGC. Although PIK3CA has historically been described as an oncogene, high levels of PIK3CA expression are signif icantly associated with better 5-year OS in EBVnGC, but not with EBVaGC (57.8% vs. 33.4%, P < 0.001). T-cell activation, and PD-L1 expression can be regulated by the IFN-γ signaling pathway [91,92]. Programmed death ligand 1 has already been targeted for molecular therapy in various cancers including GC, and clinical trials of PD-L1 monoclonal antibody in patients with advanced PD-L1-positive GC are ongoing [93–95]. The expression of PD-L1 is not as significantly increased in ARID1A-deficient GC cells as in controls treated with IFN-γ, indicating that high levels of PD-L1 expression are associated with the loss of ARID1A. A review of RNA-seq data derived from patients with GC in TCGA has revealed that EBVaGC expresses more PD-L1 immune checkpoint pathway genes than any other type of cancer. The intimate connection between EBVaGC and PD-L1 immune checkpoints requires confirmation in prospective clinical trials. Table 2 lists the genes that are frequently amplified and deleted in EBVaGC according to the TCGA [11,68]. 4.2.2. ARID1A mutations The frequency of AT-rich interactive domain-containing protein 1A (ARID1A) mutations in EBVaGC is 47% –55% , lower than the MSI subtype and higher than EBV–negative gastric cancers. The ARID1A encoded protein is part of the large ATP-dependent chromatin remod eling complex SNF/SWI, which is required for the transcriptional acti vation of genes that are normally repressed by chromatin. Functioning as a tumor suppressor, ARID1A serves as an early-stage prognostic biomarker for undifferentiated GC. One tissue immu nohistochemical microarray study concluded that the loss of 21.1% of ARID1A in 417 GC samples correlated with advanced TNM stage and was related to EBV positivity and TP53. The reduced expression of ARID1A in GC tissues targets and downregulates E-cadherin transcrip tion that enhances GC cell migration and invasion. Inhibiting AKT is effective against ARID1A-deficient GC cells in vitro. Glutathione inhibitors inhibit ARID1A-deficient GC patient-derived cells and xeno graft tumors by producing reactive oxygen species. 4.2.6. Other genes and pathways Both CD44 and HER2 are significantly amplified in EBV-positive cancers at 11p13 and 17q12, respectively. The GC stem cell marker, CD44, encodes a cell-surface glycoprotein that is involved in aggressive tumorigenic activity. The levels of CD44 variants 3–6 significantly correlates with lymph node metastasis and EBV etiology in patients with GC by immunohistochemistry. Coincidentally, CD44 is also associated with nasopharyngeal carcinoma, an EBV-associated epithelial malignancy [100,101]. The proto-oncogene HER2 (also referred as ERBB2) is overexpressed in GC, especially the genomically stable subtype and in 20%–30% of invasive breast carcinomas [11,102]. The TCGA integrated characteristic signaling pathways within each subtype to find that the most marked increased and diminished path ways in EBVaGC were IL-12 mediated signaling and hypoxia-inducible factor 1α transcription factor network, respectively. 4.2.3. BCOR mutations The ratio of BCL6 co-repressor (BCOR) mutations in EBVaGC is 23%. The BCOR encodes a transcriptional corepressor, and might spe cifically inhibit gene expression when recruited to promoter regions by sequence-specific DNA-binding proteins such as BCL6. The roles of BCOR mutations and their functions in GC remain unknown. The BCOR is critical for maintaining the primary pluripotency of human embryonic stem cells. Notably, BCOR is also associated with EBV-related extranodal natural killer/T cell lymphoma, with BCOR mutations recurring at a rate of 32%. 5. Clinicopathological features 5.1. Clinical features 4.2.4. Rare TP53 mutations Mutations of TP53 in EBV-positive GC are rare, but the rate is 71% in the chromosomal instability subtype. A comparison of TP53 mRNA levels, TP53 immunohistochemistry expression, and TP53 mutations between EBVaGC and EBVnGC showed that TP53 mRNA expression, although decreased, was higher immunohistochemically and that TP53 was rarely mutated in EBVaGC compared with EBVnGC, probably due to p53 stabilization induced by EBV. The role of p53 in tumorigenesis seems to be less significant in EBVaGC than in EBVnGC according to comparisons of DNA-ploidy profiles, immunoreactivity, and apoptosis. Most studies have shown a higher prevalence of EBVaGC in males which has been partially confirmed by meta-analyses [3,4,103,104]. Although this predominance decreases with age in terms of risk esti mates , younger patients have a higher prevalence of EBVaGC than EBVnGC [3,5,104]. Except for gender and age tendencies, EBVaGC preferentially locates in the middle and upper stomach, especially in the remnant stomach after partial gastrectomy. A Japanese study of the clinicopathological features of EBVaGC in 1,132 patients after different methods of gas trectomy showed that EBVaGC was twice as frequent in the upper or middle, than the lower stomach. The large Dutch D1D2 trial that included 566 patients with EBVnGC and EBVaGC, found EBVaGC pre dominance in the proximal part of the stomach. Rates of EBV positivity are highest in remnant GC even when the comparison is restricted to non-remnant cancers in the cardia and middle stomach. Whether EBVaGC is associated with a better prognosis is contro versial. A meta-analysis of 5,081 patients with GC from 15 international populations did not significantly associate EBV positivity with either 4.2.5. Amplification of JAK2, PD-L1, and PD-L2 Comprehensive molecular analyses of EBVaGC have revealed recurrent amplification of a group of genes at the 9p24.1 locus con taining Janus kinase 2 (JAK2), programmed death ligands1 (PD-L1), and PD-L2. Primary immunohistochemical assessment of 59 and 796 patients with EBVaGC and EBVnGC, respectively, found that JAK2, PD-L1, and PD-L2 are not significantly associated with clinicopatho logical features or the OS outcomes of patients. The JAK2 gene encodes a protein tyrosine kinase involved in a specific subset of cyto kine receptor signaling pathways. Little about JAK2 in EBVaGC has been documented. Levels of PD-L1 expression are significantly higher due to the activation of JAK2/STAT1/IRF-1 signaling in EBV positive than negative GC cell lines incubated with the same concentrations of IFN-γ for 24 h. Programmed death ligands 1 and 2 are immunosuppressant ligands of PD-1 that activate the PD-1 pathway to inhibit immune checkpoints and consequently induce immune tolerance to mature CD4+ and CD8+ T cells. High levels of PD-L1 expressed in EBVaGC cell lines suppress Table 2 Genes that are commonly amplified and deleted in EBVaGC. Amplified genes Gene JAK2 CD274 (PD-L1) PDCD1LG2 (PD-L2) ERBB2 (HER2) CD44 196 Deleted genes Location 9p24.1 9p24.2 9p24.3 17q12 11p13 Gene WWOX IMMP2L PTPRD FAM190A PTEN MACROD2 Location 16q23.1 7q31.1 9p24.1 4q22.1 10q23.31 20p12.1 J. Yang et al. Cancer Letters 495 (2020) 191–199 diagnostic clinical stage or disease duration. However, this controversy is gradually being resolved as data accu mulate. Another international pooled analysis of 4,599 patients with GC from Asia, Europe, and Latin America, inversely correlated EBV posi tivity with clinical stage. That study also associated EBV positivity with lower mortality after adjusting for clinical stage and other con founders (Hazard ratio [HR], 0.72; 95% confidence interval [CI], 0.61–0.86) , which was consistent with the findings of the Dutch D1D2 trial. The Dutch D1D2 trial explained a longer disease-free survival in EBVaGC with fewer lymph nodes involvement, less residual disease, and younger age of patients. Coincidentally, a cohort study data from the TCGA found that the prognosis of EBVaGC was the best in terms of recurrence-free and OS compared with MSI, GS, and CIN subtypes. These findings indicated that EBVaGC is a distinct entity of gastric carcinoma, characterized not only by a unique EBV genomic profile but also by distinct clinical features. Author contributions Runliang Gan conceived and designed the review. All authors collected and assembled the data. Jing Yang wrote the manuscript. Zhifeng Liu participated in the drawing and the revision of the manu script. All authors contributed to the idea. All authors also have read and agreed to the publication of this version of the manuscript. Declaration of competing interest The authors declare no conflict of interest. Acknowledgements This work was funded by the National Natural Science Foundation of China (No. 81372134), Hunan Province Key Laboratory of Tumor Cellular & Molecular Pathology (2016TP1015), and The Key Project of Hunan Provincial Health Commission (20201921). 5.2. Histopathological features References Tumors of EBVaGC usually appear ulcerated or saucer-like with an obviously increased ratio of thickness compared with EBVnGC. EBVaGC can be subdivided into histological subtypes based on degrees and proles of host cellular immune responses as lymphoepithelioma-like carcinoma (LELC), carcinoma with Crohn disease-like lymphoid reaction (CLR), and conventional-type adenocarcinoma (CA). The LELC subtype (also referred to as gastric carcinoma with lymphoid stroma [GCLS]), is a poorly differentiated adenocarcinoma with abundant, diffuse lymphocyte infiltration that resembles EBVpositive nasopharyngeal carcinoma. Tokunaga et al. reported a typical “lace pattern” in early-stage EBVaGC, which consisted of irregular anastomosing neoplastic glands and moderate-to-dense lymphocytic infiltration. Carcinoma with Crohn disease-like lymphoid reaction is characterized by three or more lymphoid follicles at the edge of the tumor with active germinal centers, fewer lymphocytes than tumor cells, and frequent tubule or gland formation. Conventional-type adenocar cinoma that is classified as well-to-moderately differentiated adeno carcinoma with a variable amount of infiltrating lymphocytes and prominent desmoplasia, is categorized in the Lauren classification as an intestinal type of gastric carcinoma. The abundance of lympho cytic infiltration causes difficulties with differentiating carcinoma cell types by routine hematoxylin and eosin (H&E) staining. In all the above situations, EBV positive gastric carcinoma cells should be verified by EBER ISH. Recent studies have shown that patients with LELC and LELC + CLR have significantly longer OS (HR, 0.09 and 0.42, respectively) and DFS (HR, 0.05 and 0.46, respectively; P < 0.02). The present study provides evidence that the prognosis of EBVaGC is partly dependent on the inflammatory responses of patients. F. Bray, J. Ferlay, I. Soerjomataram, R.L. Siegel, L.A. Torre, A. Jemal, Global cancer statistics 2018: GLOBOCAN estimates of incidence and mortality worldwide for 36 cancers in 185 countries, CA A Cancer J. Clin. 68 (2018) 394–424. L.S. Young, A.B. Rickinson, Epstein-Barr virus: 40 years on, Nat. Rev. Canc. 4 (2004) 757–768. G. Murphy, R. Pfeiffer, M.C. Camargo, C.S. Rabkin, Meta-analysis shows that prevalence of Epstein-Barr virus-positive gastric cancer differs based on sex and anatomic location, Gastroenterology 137 (2009) 824–833. M.C. Camargo, G. Murphy, C. Koriyama, R.M. Pfeiffer, W.H. Kim, R. HerreraGoepfert, A.H. Corvalan, E. Carrascal, A. Abdirad, M. Anwar, Z. Hao, J. Kattoor, E. Yoshiwara-Wakabayashi, Y. Eizuru, C.S. Rabkin, S. Akiba, Determinants of Epstein-Barr virus-positive gastric cancer: an international pooled analysis, Br. J. Canc. 105 (2011) 38–43. M. Naseem, A. Barzi, C. Brezden-Masley, A. Puccini, M.D. Berger, R. Tokunaga, F. Battaglin, S. Soni, M. McSkane, W. Zhang, H.J. Lenz, Outlooks on Epstein-Barr virus associated gastric cancer, Canc. Treat Rev. 66 (2018) 15–22. R. Cristescu, J. Lee, M. Nebozhyn, K.-M. Kim, J.C. Ting, S.S. Wong, J. Liu, Y. G. Yue, J. Wang, K. Yu, X.S. Ye, I.-G. Do, S. Liu, L. Gong, J. Fu, J.G. Jin, M.G. Choi, T.S. Sohn, J.H. Lee, J.M. Bae, S.T. Kim, S.H. Park, I. Sohn, S.-H. Jung, P. Tan, R. Chen, J. Hardwick, W.K. Kang, M. Ayers, D. Hongyue, C. Reinhard, A. Loboda, S. Kim, A. Aggarwal, Molecular analysis of gastric cancer identifies subtypes associated with distinct clinical outcomes, Nat. Med. 21 (2015) 449–456. M.-S. Chang, H. Uozaki, J.-M. Chong, T. Ushiku, K. Sakuma, S. Ishikawa, R. Hino, R.R. Barua, Y. Iwasaki, K. Arai, H. Fujii, H. Nagai, M. Fukayama, CpG island methylation status in gastric carcinoma with and without infection of EpsteinBarr virus, Clin. Canc. Res. : an official journal of the American Association for Cancer Research 12 (2006) 2995–3002. G.H. Kang, S. Lee, W.H. Kim, H.W. Lee, J.C. Kim, M.-G. Rhyu, J.Y. Ro, Epsteinbarr virus-positive gastric carcinoma demonstrates frequent aberrant methylation of multiple genes and constitutes CpG island methylator phenotype-positive gastric carcinoma, Am. J. Pathol. 160 (2002) 787–794. K. Wang, S.T. Yuen, J. Xu, S.P. Lee, H.H.N. Yan, S.T. Shi, H.C. Siu, S. Deng, K. M. Chu, S. Law, K.H. Chan, A.S.Y. Chan, W.Y. Tsui, S.L. Ho, A.K.W. Chan, J.L. K. Man, V. Foglizzo, M.K. Ng, A.S. Chan, Y.P. Ching, G.H.W. Cheng, T. Xie, J. Fernandez, V.S.W. Li, H. Clevers, P.A. Rejto, M. Mao, S.Y. Leung, Wholegenome sequencing and comprehensive molecular profiling identify new driver mutations in gastric cancer, Nat. Genet. 46 (2014) 573–582. K. Wang, J. Kan, S.T. Yuen, S.T. Shi, K.M. Chu, S. Law, T.L. Chan, Z. Kan, A.S. Y. Chan, W.Y. Tsui, S.P. Lee, S.L. Ho, A.K.W. Chan, G.H.W. Cheng, P.C. Roberts, P. A. Rejto, N.W. Gibson, D.J. Pocalyko, M. Mao, J. Xu, S.Y. Leung, Exome sequencing identifies frequent mutation of ARID1A in molecular subtypes of gastric cancer, Nat. Genet. 43 (2011) 1219–1223. N. Cancer, Genome Atlas Research, Comprehensive molecular characterization of gastric adenocarcinoma, Nature 513 (2014) 202–209. A.P. Burke, T.S. Yen, K.M. Shekitka, L.H. Sobin, Lymphoepithelial carcinoma of the stomach with Epstein-Barr virus demonstrated by polymerase chain reaction, Mod. Pathol. 3 (1990) 377–380. M. Tokunaga, C.E. Land, Y. Uemura, T. Tokudome, S. Tanaka, E. Sato, EpsteinBarr virus in gastric carcinoma, Am. J. Pathol. 143 (1993) 1250–1254. T. Shuto, J. Nishikawa, K. Shimokuri, A. Yanagi, T. Takagi, F. Takagi, O. Miura, M. Iida, H. Nagano, Y. Takemoto, E. Harada, Y. Suehiro, T. Yamasaki, T. Okamoto, I. Sakaida, Establishment of a screening method for epstein-barr virus-associated gastric carcinoma by droplet digital PCR, Microorganisms 7 (2019). M.A. Epstein, B.G. Achong, Y.M. Barr, Virus particles in cultured lymphoblasts from burkitt’s lymphoma, Lancet 1 (1964) 702–703. C. Shannon-Lowe, A. Rickinson, The global landscape of EBV-associated tumors, Front Oncol 9 (2019) 713. 6. Conclusions Tagged by unique clinicopathological and molecular features, EBVaGC is a distinct subtype of gastric carcinoma associated with EBV infection. Here, we discussed EBV infection, EBV viral genome expres sion, as well as clinical and histopathological features, especially the molecular characteristics of EBVaGC. However, the specific mechanism of EBV infection in gastric carcinogenesis and the characteristics of the genomic profile of cancer cells requires further exploration. Fig. 3 shows the roles of EBV and aberrant host genome in the oncogenesis of gastric cancer. Understanding the effects of the EBV viral genome on host cells will facilitate early-stage diagnosis and targeted treatment strategies. Traits of the inter-regulated relationships among EBV, tumor cells, extreme DNA hypermethylation profiles, and somatic genomic abnor malities can be traced to improve the prognosis of patients with EBVaGC. 197 J. Yang et al. Cancer Letters 495 (2020) 191–199 S. Pittaluga, S.L. Loke, K.C. So, K.N. Cheung, L. Ma, Clonal Epstein-Barr virus in lymphoepithelioma-like carcinoma of the stomach: demonstration of viral genome by in situ hybridization and Southern blot analysis, Mod. Pathol. 5 (1992) 661–664. M.-Z. Qiu, C.-Y. He, S.-X. Lu, W.-L. Guan, F. Wang, X.-J. Wang, Y. Jin, F.-H. Wang, Y.-H. Li, J.-Y. Shao, Z.-W. Zhou, J.-P. Yun, R.-H. Xu, Prospective observation: clinical utility of plasma Epstein-Barr virus DNA load in EBV-associated gastric carcinoma patients, Int. J. Canc. 146 (2020) 272–280. M.K. Smatti, D.W. Al-Sadeq, N.H. Ali, G. Pintus, H. Abou-Saleh, G.K. Nasrallah, Epstein-Barr virus epidemiology, serology, and genetic variability of LMP-1 oncogene among healthy population: an update, Front Oncol 8 (2018) 211. H. Yoshiyama, S. Imai, N. Shimizu, K. Takada, Epstein-Barr virus infection of human gastric carcinoma cells: implication of the existence of a new virus receptor different from CD21, J. Virol. 71 (1997) 5688–5691. S. Imai, J. Nishikawa, K. Takada, Cell-to-cell contact as an efficient mode of Epstein-Barr virus infection of diverse human epithelial cells, J. Virol. 72 (1998) 4371–4378. D. Shibata, L.M. Weiss, Epstein-Barr virus-associated gastric adenocarcinoma, Am. J. Pathol. 140 (1992) 769–774. S. Imai, S. Koizumi, M. Sugiura, M. Tokunaga, Y. Uemura, N. Yamamoto, S. Tanaka, E. Sato, T. Osato, Gastric carcinoma: monoclonal epithelial malignant cells expressing Epstein-Barr virus latent infection protein, Proc. Natl. Acad. Sci. U.S.A. 91 (1994) 9131–9135. C. Münz, Latency and lytic replication in Epstein-Barr virus-associated oncogenesis, Nat. Rev. Microbiol. 17 (2019) 691–700. A.M. Price, M.A. Luftig, To be or not IIb: a multi-step process for Epstein-Barr virus latency establishment and consequences for B cell tumorigenesis, PLoS Pathog. 11 (2015), e1004656. B. Luo, Y. Wang, X.F. Wang, H. Liang, L.P. Yan, B.H. Huang, P. Zhao, Expression of Epstein-Barr virus genes in EBV-associated gastric carcinomas, World J. Gastroenterol. 11 (2005) 629–633. M. Sugiura, S. Imai, M. Tokunaga, S. Koizumi, M. Uchizawa, K. Okamoto, T. Osato, Transcriptional analysis of Epstein-Barr virus gene expression in EBVpositive gastric carcinoma: unique viral latency in the tumour cells, Br. J. Canc. 74 (1996) 625–631. M.J. Strong, G. Xu, J. Coco, C. Baribault, D.S. Vinay, M.R. Lacey, A.L. Strong, T. A. Lehman, M.B. Seddon, Z. Lin, M. Concha, M. Baddoo, M. Ferris, K.F. Swan, D. E. Sullivan, M.E. Burow, C.M. Taylor, E.K. Flemington, Differences in gastric carcinoma microenvironment stratify according to EBV infection intensity: implications for possible immune adjuvant therapy, PLoS Pathog. 9 (2013), e1003341. W. Tang, D.R. Morgan, M.O. Meyers, R.L. Dominguez, E. Martinez, K. Kakudo, P. F. Kuan, N. Banet, H. Muallem, K. Woodward, O. Speck, M.L. Gulley, Epstein-barr virus infected gastric adenocarcinoma expresses latent and lytic viral transcripts and has a distinct human gene expression profile, Infect. Agents Canc. 7 (2012) 21. D. Iwakiri, Y. Eizuru, M. Tokunaga, K. Takada, Autocrine growth of Epstein-Barr virus-positive gastric carcinoma cells mediated by an Epstein-Barr virus-encoded small RNA, Canc. Res. 63 (2003) 7062–7067. A.S. Banerjee, A.D. Pal, S. Banerjee, Epstein-Barr virus-encoded small non-coding RNAs induce cancer cell chemoresistance and migration, Virology 443 (2013) 294–305. T.-C. Cheng, S.-S. Hsieh, W.-L. Hsu, Y.-F. Chen, H.-H. Ho, L.-F. Sheu, Expression of Epstein-Barr nuclear antigen 1 in gastric carcinoma cells is associated with enhanced tumorigenicity and reduced cisplatin sensitivity, Int. J. Oncol. 36 (2010) 151–160. F. Lu, I. Tempera, H.T. Lee, K. Dewispelaere, P.M. Lieberman, EBNA1 binding and epigenetic regulation of gastrokine tumor suppressor genes in gastric carcinoma cells, Virol. J. 11 (2014) 12. K. Hayashi, W.G. Chen, Y.Y. Chen, I. Murakami, H.L. Chen, N. Ohara, S. Nose, K. Hamaya, S. Matsui, M.M. Bacchi, C.E. Bacchi, K.L. Chang, L.M. Weiss, Deletion of Epstein-Barr virus latent membrane protein 1 gene in Japanese and Brazilian gastric carcinomas, metastatic lesions, and reactive lymphocytes, Am. J. Pathol. 152 (1998) 191–198. S.T. Cheung, K.W. Lo, S.F. Leung, W.Y. Chan, P.H. Choi, P.J. Johnson, J.C. Lee, D. P. Huang, Prevalence of LMP1 deletion variant of Epstein-Barr virus in nasopharyngeal carcinoma and gastric tumors in Hong Kong, Int. J. Canc. 66 (1996) 711–712. J. Li, X. Liu, M. Liu, K. Che, B. Luo, Methylation and expression of Epstein-Barr virus latent membrane protein 1, 2A and 2B in EBV-associated gastric carcinomas and cell lines, Dig. Liver Dis. 48 (2016) 673–680. Y. Wang, B. Luo, P. Zhao, B.-H. Huang, [Expression of Epstein-Barr virus genes in EBV-associated gastric carcinoma], Ai Zheng 23 (2004) 782–787. L.W. Wang, S. Jiang, B.E. Gewurz, Epstein-Barr virus LMP1-mediated oncogenicity, J. Virol. 91 (2017). L.F. Sheu, A. Chen, Y.H. Wei, K.C. Ho, J.Y. Cheng, C.L. Meng, W.H. Lee, EpsteinBarr virus LMP1 modulates the malignant potential of gastric carcinoma cells involving apoptosis, Am. J. Pathol. 152 (1998) 63–74. J. Nishikawa, S. Imai, T. Oda, T. Kojima, K. Okita, K. Takada, Epstein-Barr virus promotes epithelial cell growth in the absence of EBNA2 and LMP1 expression, J. Virol. 73 (1999) 1286–1292. R. Hino, H. Uozaki, Y. Inoue, Y. Shintani, T. Ushiku, T. Sakatani, K. Takada, M. Fukayama, Survival advantage of EBV-associated gastric carcinoma: survivin up-regulation by viral latent membrane protein 2A, Canc. Res. 68 (2008) 1427–1435. J. Wang, W. Liu, X. Zhang, Y. Zhang, H. Xiao, B. Luo, LMP2A induces DNA methylation and expression repression of AQP3 in EBV-associated gastric carcinoma, Virology 534 (2019) 87–95. F. Wang, W. Chen, P. Liu, J. Zhou, B. Liu, W. Ye, W. Wang, X. Shen, Lentivirusmediated RNAi knockdown of inhibits the growth of the Epstein-Barr-associated gastric carcinoma cell line GT38, Exp Ther Med 13 (2017) 187–193. J.S. Seo, S.M. Jun, S.W. Kwon, I.-H. Oh, T.-G. Kim, S.K. Lee, Establishment and characterization of gastric carcinoma cell clones expressing LMP2A of EpsteinBarr virus, Int. J. Mol. Med. 25 (2010) 11–16. S. Pfeffer, M. Zavolan, F.A. Grässer, M. Chien, J.J. Russo, J. Ju, B. John, A. J. Enright, D. Marks, C. Sander, T. Tuschl, Identification of virus-encoded microRNAs, Science (New York, N.Y.) 304 (2004) 734–736. O. Klinke, R. Feederle, H.-J. Delecluse, Genetics of Epstein-Barr virus microRNAs, Semin. Canc. Biol. 26 (2014) 52–59. A. Shinozaki-Ushiku, A. Kunita, M. Isogai, T. Hibiya, T. Ushiku, K. Takada, M. Fukayama, Profiling of virus-encoded MicroRNAs in epstein-barr virusassociated gastric carcinoma and their roles in gastric carcinogenesis, J. Virol. 89 (2015) 5581–5591. B.G. Jang, E.J. Jung, W.H. Kim, Expression of BamHI-A rightward transcripts in epstein-barr virus-associated gastric cancers, Cancer Res Treat 43 (2011) 250–254. A. zur Hausen, A.A. Brink, M.E. Craanen, J.M. Middeldorp, C.J. Meijer, A.J. van den Brule, Unique transcription pattern of Epstein-Barr virus (EBV) in EBVcarrying gastric adenocarcinomas: expression of the transforming BARF1 gene, Canc. Res. 60 (2000) 2745–2748. A.R. Marquitz, A. Mathur, P.E. Chugh, D.P. Dittmer, N. Raab-Traub, Expression profile of microRNAs in Epstein-Barr virus-infected AGS gastric carcinoma cells, J. Virol. 88 (2014) 1389–1393. X. Zheng, J. Wang, L. Wei, Q. Peng, Y. Gao, Y. Fu, Y. Lu, Z. Qin, X. Zhang, J. Lu, C. Ou, Z. Li, X. Zhang, P. Liu, W. Xiong, G. Li, Q. Yan, J. Ma, Epstein-Barr virus MicroRNA miR-BART5-3p inhibits p53 expression, J. Virol. (2018) 92. M. Wang, F. Yu, W. Wu, Y. Wang, H. Ding, L. Qian, Epstein-Barr virus-encoded microRNAs as regulators in host immune responses, Int. J. Biol. Sci. 14 (2018) 565–576. M.X. Wei, M. de Turenne-Tessier, G. Decaussin, G. Benet, T. Ooka, Establishment of a monkey kidney epithelial cell line with the BARF1 open reading frame from Epstein-Barr virus, Oncogene 14 (1997) 3073–3081. E. Sakka, A. Zur Hausen, K. Houali, H. Liu, S. Fiorini, T. Ooka, Cellular localization of BARF1 oncoprotein and its cell stimulating activity in human epithelial cell, Virus Res. (2013) 174. N. Tarbouriech, F. Ruggiero, M. de Turenne-Tessier, T. Ooka, W.P. Burmeister, Structure of the Epstein-Barr virus oncogene BARF1, J. Mol. Biol. 359 (2006) 667–678. A. Sall, S. Caserta, P. Jolicoeur, L. Franqueville, M. de Turenne-Tessier, T. Ooka, Mitogenic activity of Epstein-Barr virus-encoded BARF1 protein, Oncogene 23 (2004) 4938–4944. E. Seto, L. Yang, J. Middeldorp, T.-S. Sheen, J.-Y. Chen, M. Fukayama, Y. Eizuru, T. Ooka, K. Takada, Epstein-Barr virus (EBV)-encoded BARF1 gene is expressed in nasopharyngeal carcinoma and EBV-associated gastric carcinoma tissues in the absence of lytic gene expression, J. Med. Virol. 76 (2005) 82–88. A. zur Hausen, A.A. Brink, M.E. Craanen, J.M. Middeldorp, C.J. Meijer, A.J. van den Brule, Unique transcription pattern of Epstein-Barr virus (EBV) in EBVcarrying gastric adenocarcinomas: expression of the transforming BARF1 gene, Canc. Res. 60 (2000) 2745–2748. Q. Wang, S.W. Tsao, T. Ooka, J.M. Nicholls, H.W. Cheung, S. Fu, Y.C. Wong, X. Wang, Anti-apoptotic role of BARF1 in gastric cancer cells, Canc. Lett. 238 (2006) 90–103. M.X. Wei, T. Ooka, A transforming function of the BARF1 gene encoded by Epstein-Barr virus, EMBO J. 8 (1989) 2897–2903. M.S. Chang, D.H. Kim, J.K. Roh, J.M. Middeldorp, Y.S. Kim, S. Kim, S. Han, C. W. Kim, B.L. Lee, W.H. Kim, J.H. Woo, Epstein-Barr virus-encoded BARF1 promotes proliferation of gastric carcinoma cells through regulation of NF-κB, J. Virol. 87 (2013) 10515–10523. R. Turrini, A. Merlo, D. Martorelli, D.A. Faè, R. Sommaggio, I.M. Montagner, V. Barbieri, O. Marin, P. Zanovello, R. Dolcetti, A. Rosato, A BARF1-specific mAb as a new immunotherapeutic tool for the management of EBV-related tumors, OncoImmunology 6 (2017), e1304338. I. Borozan, M. Zapatka, L. Frappier, V. Ferretti, Analysis of epstein-barr virus genomes and expression profiles in gastric adenocarcinoma, J. Virol. (2018) 92. K. Matsusaka, A. Kaneda, G. Nagae, T. Ushiku, Y. Kikuchi, R. Hino, H. Uozaki, Y. Seto, K. Takada, H. Aburatani, M. Fukayama, Classification of Epstein-Barr virus-positive gastric cancers by definition of DNA methylation epigenotypes, Canc. Res. 71 (2011) 7187–7197. Z. Lei, I.B. Tan, K. Das, N. Deng, H. Zouridis, S. Pattison, C. Chua, Z. Feng, Y. K. Guan, C.H. Ooi, T. Ivanova, S. Zhang, M. Lee, J. Wu, A. Ngo, S. Manesh, E. Tan, B.T. Teh, J.B.Y. So, L.K. Goh, A. Boussioutas, T.K.H. Lim, H. Flotow, P. Tan, S. G. Rozen, Identification of molecular subtypes of gastric cancer with different responses to PI3-kinase inhibitors and 5-fluorouracil, Gastroenterology 145 (2013) 554–565. H. Geddert, A. zur Hausen, H.E. Gabbert, M. Sarbia, EBV-infection in cardiac and non-cardiac gastric adenocarcinomas is associated with promoter methylation of p16, p14 and APC, but not hMLH1, Cell. Oncol. 34 (2011) 209–214. K. Sakuma, J.-M. Chong, M. Sudo, T. Ushiku, Y. Inoue, J. Shibahara, H. Uozaki, H. Nagai, M. Fukayama, High-density methylation of p14ARF and p16INK4A in Epstein-Barr virus-associated gastric carcinoma, Int. J. Canc. 112 (2004) 273–278. 198 J. Yang et al. Cancer Letters 495 (2020) 191–199 M.L. Gulley, Genomic assays for Epstein-Barr virus-positive gastric adenocarcinoma, Exp. Mol. Med. 47 (2015) e134. H. Uozaki, M. Fukayama, Epstein-Barr virus and gastric carcinoma–viral carcinogenesis through epigenetic mechanisms, Int. J. Clin. Exp. Pathol. 1 (2008) 198–216. J. Zhao, Q. Liang, K.-F. Cheung, W. Kang, R.W.M. Lung, J.H.M. Tong, K.F. To, J.J. Y. Sung, J. Yu, Genome-wide identification of Epstein-Barr virus-driven promoter methylation profiles of human genes in gastric cancer cells, Cancer 119 (2013) 304–312. Q. Liang, X. Yao, S. Tang, J. Zhang, T.O. Yau, X. Li, C.M. Tang, W. Kang, R. W. Lung, J.W. Li, T.F. Chan, R. Xing, Y. Lu, K.W. Lo, N. Wong, K.F. To, C. Yu, F. K. Chan, J.J. Sung, J. Yu, Integrative identification of Epstein-Barr virusassociated mutations and epigenetic alterations in gastric cancer, Gastroenterology 147 (2014) 1350–1362. A. Kaneda, K. Matsusaka, H. Aburatani, M. Fukayama, Epstein-Barr virus infection as an epigenetic driver of tumorigenesis, Canc. Res. 72 (2012) 3445–3450. Q. Tao, K.D. Robertson, Stealth technology: how Epstein-Barr virus utilizes DNA methylation to cloak itself from immune detection, Clin. Immunol. 109 (2003) 53–63. B.J. Schneider, M.A. Shah, K. Klute, A. Ocean, E. Popa, N. Altorki, M. Lieberman, A. Schreiner, R. Yantiss, P.J. Christos, R. Palmer, D. You, A. Viale, P. Kermani, J. M. Scandura, Phase I study of epigenetic priming with azacitidine prior to standard neoadjuvant chemotherapy for patients with resectable gastric and esophageal adenocarcinoma: evidence of tumor hypomethylation as an indicator of major histopathologic response, Clin. Canc. Res. : an official journal of the American Association for Cancer Research 23 (2017) 2673–2680. C. Böger, S. Krüger, H.M. Behrens, S. Bock, J. Haag, H. Kalthoff, C. Röcken, Epstein-Barr virus-associated gastric cancer reveals intratumoral heterogeneity of PIK3CA mutations, Ann. Oncol. 28 (2017) 1005–1014. M. Dong, H.-Y. Wang, X.-X. Zhao, J.-N. Chen, Y.-W. Zhang, Y. Huang, L. Xue, H.G. Li, H. Du, X.-Y. Wu, C.-K. Shao, Expression and prognostic roles of PIK3CA, JAK2, PD-L1, and PD-L2 in Epstein-Barr virus-associated gastric carcinoma, Hum. Pathol. 53 (2016) 25–34. M. Ashizawa, M. Saito, A.K.T. Min, D. Ujiie, K. Saito, T. Sato, T. Kikuchi, H. Okayama, S. Fujita, H. Endo, W. Sakamoto, T. Momma, S. Ohki, A. Goto, K. Kono, Prognostic role of ARID1A negative expression in gastric cancer, Sci. Rep. 9 (2019) 6769. N. Han, M.A. Kim, H.S. Lee, W.H. Kim, Loss of ARID1A expression is related to gastric cancer progression, epstein-barr virus infection, and mismatch repair deficiency, Appl. Immunohistochem. Mol. Morphol. 24 (2016) 320–325. H.-B. Yan, X.-F. Wang, Q. Zhang, Z.-Q. Tang, Y.-H. Jiang, H.-Z. Fan, Y.-h. Sun, P.Y. Yang, F. Liu, Reduced expression of the chromatin remodeling gene ARID1A enhances gastric cancer cell migration and invasion via downregulation of Ecadherin transcription, Carcinogenesis 35 (2014) 867–876. D. Lee, E.J. Yu, I.-H. Ham, H. Hur, Y.-S. Kim, AKT inhibition is an effective treatment strategy in ARID1A-deficient gastric cancer cells, OncoTargets Ther. 10 (2017) 4153–4159. M. Sasaki, F. Chiwaki, T. Kuroda, M. Komatsu, K. Matsusaki, T. Kohno, H. Sasaki, H. Ogiwara, Efficacy of glutathione inhibitors for the treatment of ARID1Adeficient diffuse-type gastric cancers, Biochem. Biophys. Res. Commun. 522 (2020) 342–347. A. Astolfi, M. Fiore, F. Melchionda, V. Indio, S.N. Bertuccio, A. Pession, BCOR involvement in cancer, Epigenomics 11 (2019) 835–855. Z. Wang, M.D. Gearhart, Y.-W. Lee, I. Kumar, B. Ramazanov, Y. Zhang, C. Hernandez, A.Y. Lu, N. Neuenkirchen, J. Deng, J. Jin, Y. Kluger, T.A. Neubert, V.J. Bardwell, N.B. Ivanova, A non-canonical BCOR-prc1.1 complex represses differentiation programs in human ESCs, Cell stem cell 22 (2018). A. Dobashi, N. Tsuyama, R. Asaka, Y. Togashi, K. Ueda, S. Sakata, S. Baba, K. Sakamoto, K. Hatake, K. Takeuchi, Frequent BCOR aberrations in extranodal NK/T-Cell lymphoma, nasal type, Genes Chromosomes Cancer 55 (2016) 460–471. J. Ribeiro, M. Malta, A. Galaghar, F. Silva, L.P. Afonso, R. Medeiros, H. Sousa, P53 deregulation in Epstein-Barr virus-associated gastric cancer, Canc. Lett. 404 (2017) 37–43. S. Moritani, H. Sugihara, R. Kushima, T. Hattori, Different roles of p53 between Epstein-Barr virus-positive and -negative gastric carcinomas of matched histology, Virchows Arch. : an international journal of pathology 440 (2002) 367–375. M. Dong, H.-y. Wang, X.-x. Zhao, J.-n. Chen, Y.-w. Zhang, Y. Huang, L. Xue, H.g. Li, H. Du, X.-y. Wu, Expression and prognostic roles of PIK3CA, JAK2, PD-L1, and PD-L2 in Epstein-Barr virus–associated gastric carcinoma, Hum. Pathol. 53 (2016) 25–34. K. Qamar, M. Saboor, Jak 2 and stat proteins; a mini review, Biomedica 34 (2018) 232. J.W. Moon, S.-K. Kong, B.S. Kim, H.J. Kim, H. Lim, K. Noh, Y. Kim, J.-W. Choi, J.H. Lee, Y.-S. Kim, IFNγ induces PD-L1 overexpression by JAK2/STAT1/IRF-1 signaling in EBV-positive gastric carcinoma, Sci. Rep. 7 (2017) 17810. K.M. Zak, P. Grudnik, K. Magiera, A. Dömling, G. Dubin, T.A. Holak, Structural biology of the immune checkpoint receptor PD-1 and its ligands PD-L1/PD-L2, Structure 25 (2017) 1163–1174. S. Sasaki, J. Nishikawa, K. Sakai, H. Iizasa, H. Yoshiyama, M. Yanagihara, T. Shuto, K. Shimokuri, T. Kanda, Y. Suehiro, T. Yamasaki, I. Sakaida, EBV- 199 associated gastric cancer evades T-cell immunity by PD-1/PD-L1 interactions, Gastric cancer, official journal of the International Gastric Cancer Association and the Japanese Gastric Cancer Association 22 (2019) 486–496. J.W. Moon, S.K. Kong, B.S. Kim, H.J. Kim, H. Lim, K. Noh, Y. Kim, J.W. Choi, J. H. Lee, Y.S. Kim, IFNgamma induces PD-L1 overexpression by JAK2/STAT1/IRF1 signaling in EBV-positive gastric carcinoma, Sci. Rep. 7 (2017) 17810. K. Muro, H.C. Chung, V. Shankaran, R. Geva, D. Catenacci, S. Gupta, J.P. Eder, T. Golan, D.T. Le, B. Burtness, A.J. McRee, C.-C. Lin, K. Pathiraja, J. Lunceford, K. Emancipator, J. Juco, M. Koshiji, Y.-J. Bang, Pembrolizumab for patients with PD-L1-positive advanced gastric cancer (KEYNOTE-012): a multicentre, openlabel, phase 1b trial, the Lancet, Oncology 17 (2016) 717–726. T. Doi, S. Iwasa, K. Muro, T. Satoh, S. Hironaka, T. Esaki, T. Nishina, H. Hara, N. Machida, Y. Komatsu, Y. Shimada, S. Otsu, S. Shimizu, M. Watanabe, Phase 1 trial of avelumab (anti-PD-L1) in Japanese patients with advanced solid tumors, including dose expansion in patients with gastric or gastroesophageal junction cancer: the JAVELIN Solid Tumor JPN trial, Gastric Cancer 22 (2019) 817–827. X. Ni, Y. Xing, X. Sun, J. Suo, The Safety and Efficacy of Anti-PD-1/anti-PD-L1 Antibody Therapy in the Treatment of Previously Treated, Advanced Gastric or Gastro-Oesophageal Junction Cancer: A Meta-Analysis of Prospective Clinical Trials, Clin Res Hepatol Gastroenterol, 2019. Y.B. Kim, J.M. Ahn, W.J. Bae, C.O. Sung, D. Lee, Functional loss of ARID1A is tightly associated with high PD-L1 expression in gastric cancer, Int. J. Canc. 145 (2019) 916–926. A. Panda, J.M. Mehnert, K.M. Hirshfield, G. Riedlinger, S. Damare, T. Saunders, M. Kane, L. Sokol, M.N. Stein, E. Poplin, Immune activation and benefit from avelumab in EBV-positive gastric cancer, JNCI, J. Natl. Cancer Inst. 110 (2018) 316–320. S. Takaishi, T. Okumura, S. Tu, S.S. Wang, W. Shibata, R. Vigneshwaran, S. A. Gordon, Y. Shimada, T.C. Wang, Identification of gastric cancer stem cells using the cell surface marker CD44, Stem Cell. 27 (2009) 1006–1020. J.M. Chong, M. Fukayama, Y. Hayashi, N. Funata, T. Takizawa, M. Koike, M. Muraoka, R. Kikuchi-Yanoshita, M. Miyaki, S. Mizuno, Expression of CD44 variants in gastric carcinoma with or without Epstein-Barr virus, Int. J. Canc. 74 (1997) 450–454. A.M. Janisiewicz, J.H. Shin, O. Murillo-Sauca, S. Kwok, Q.-T. Le, C. Kong, M. J. Kaplan, J.B. Sunwoo, CD44(+) cells have cancer stem cell-like properties in nasopharyngeal carcinoma, Int Forum Allergy Rhinol 2 (2012) 465–470. S.W.-M. Lun, S.T. Cheung, P.F.Y. Cheung, K.-F. To, J.K.-S. Woo, K.-W. Choy, C. Chow, C.C.-M. Cheung, G.T.-Y. Chung, A.S.-H. Cheng, C.-W. Ko, S.-W. Tsao, P. Busson, M.H.-L. Ng, K.-W. Lo, CD44+ cancer stem-like cells in EBV-associated nasopharyngeal carcinoma, PloS One 7 (2012), e52426. F. Meric-Bernstam, A.M. Johnson, E.E.I. Dumbrava, K. Raghav, K. Balaji, M. Bhatt, R.K. Murthy, J. Rodon, S.A. Piha-Paul, Advances in HER2-targeted therapy: novel agents and opportunities beyond breast and gastric cancer, Clin. Canc. Res. : an official journal of the American Association for Cancer Research 25 (2019) 2033–2041. A. Yanagi, J. Nishikawa, K. Shimokuri, T. Shuto, T. Takagi, F. Takagi, Y. Kobayashi, M. Yamamoto, O. Miura, H. Yanai, Y. Suehiro, T. Yamasaki, H. Yoshiyama, I. Sakaida, Clinicopathologic characteristics of epstein-barr virusassociated gastric cancer over the past decade in Japan, Microorganisms (2019) 7. J. van Beek, A. zur Hausen, E. Klein Kranenbarg, C.J.H. van de Velde, J. M. Middeldorp, A.J.C. van den Brule, C.J.L.M. Meijer, E. Bloemena, EBV-positive gastric adenocarcinomas: a distinct clinicopathologic entity with a low frequency of lymph node involvement, J. Clin. Oncol. : official journal of the American Society of Clinical Oncology 22 (2004) 664–670. N. Yamamoto, M. Tokunaga, Y. Uemura, S. Tanaka, H. Shirahama, T. Nakamura, C.E. Land, E. Sato, Epstein-Barr virus and gastric remnant cancer, Cancer 74 (1994) 805–809. M.C. Camargo, W.-H. Kim, A.M. Chiaravalli, K.-M. Kim, A.H. Corvalan, K. Matsuo, J. Yu, J.J.Y. Sung, R. Herrera-Goepfert, F. Meneses-Gonzalez, Y. Kijima, S. Natsugoe, L.M. Liao, J. Lissowska, S. Kim, N. Hu, C.A. Gonzalez, Y. Yatabe, C. Koriyama, S.M. Hewitt, S. Akiba, M.L. Gulley, P.R. Taylor, C.S. Rabkin, Improved survival of gastric cancer with tumour Epstein-Barr virus positivity: an international pooled analysis, Gut 63 (2014) 236–243. B.H. Sohn, J.E. Hwang, H.J. Jang, H.S. Lee, S.C. Oh, J.J. Shim, K.W. Lee, E. H. Kim, S.Y. Yim, S.H. Lee, J.H. Cheong, W. Jeong, J.Y. Cho, J. Kim, J. Chae, J. Lee, W.K. Kang, S. Kim, S.H. Noh, J.A. Ajani, J.S. Lee, Clinical Significance of Four Molecular Subtypes of Gastric Cancer Identified by the Cancer Genome Atlas Project, Clin Cancer Res, 2017. H.-J. Song, K.-M. Kim, Pathology of epstein-barr virus-associated gastric carcinoma and its relationship to prognosis, Gut Liver 5 (2011) 143–148. Y. Uemura, M. Tokunaga, J. Arikawa, N. Yamamoto, Y. Hamasaki, S. Tanaka, E. Sato, C.E. Land, A unique morphology of Epstein-Barr virus-related early gastric carcinoma, Cancer epidemiology, biomarkers & prevention : a publication of the American Association for Cancer Research, cosponsored by the American Society of Preventive Oncology 3 (1994) 607–611. A. Shinozaki-Ushiku, A. Kunita, M. Fukayama, Update on Epstein-Barr virus and gastric cancer (review), Int. J. Oncol. 46 (2015) 1421–1434. H.-J. Song, A. Srivastava, J. Lee, Y.S. Kim, K.-M. Kim, W. Ki Kang, M. Kim, S. Kim, C.K. Park, S. Kim, Host inflammatory response predicts survival of patients with Epstein-Barr virus-associated gastric carcinoma, Gastroenterology (2010) 139.