Microscopy Notes PDF
Document Details
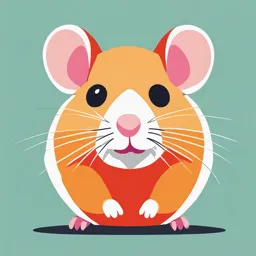
Uploaded by SimplestParadise
Tags
Summary
These notes provide a comprehensive overview of microscopy, including imaging techniques, history, factors that affect image quality, types of microscopes, and the relationship between magnification, resolution, and contrast. It details the various objective lenses, eyepieces, and filters.
Full Transcript
MICROSCOPY ANALYTICAL CHEMISTRY MICROSCOPY the most common technique used to study the structural details of cells, tissues and organelles present in plants and animals. useful in visualization of cells and microorga...
MICROSCOPY ANALYTICAL CHEMISTRY MICROSCOPY the most common technique used to study the structural details of cells, tissues and organelles present in plants and animals. useful in visualization of cells and microorganisms as the images appear much larger after magnification direct observation of bacteria under a microscope is the simplest and cheapest way for bacteria identification. Bacteria are identified and classified based on the various shapes, sizes, and arrangements. MICROSCOPY (IMAGING TECHNIQUES) MICROSCOPIC MICROSCOPE too small to be seen by the an instrument used to view small things naked/unaided eye. that cannot be seen with the unaided animal cell (20–30 μm) eyes. red blood cell (7.6 μm) erived from the word “micro” = small and cell membrane (10 nm) “scope” = to look at. bacterium (0.5–5 μm) magnifies objects so that specimens can virus (10–100 nm) easily be seen HISTORY OF MICROSCOPE 1267 - R. Bacon invented the eyeglasses (concept of magnification) 1538 - Girolamo Fracastoro observed the object appears larger under 2 glasses are superimposed with one another 1590 - the 1st microscope developed by Hans and Zacharias Jansen 1665 - Robert Hooke described the appearance of cork and used the term “cell” 1668 - Anton van Leeuwenhoek was the 1st to observe living cells MICROSCOPY used by scientists and healthcare professionals 1. diagnosis of infectious diseases, identification of microorganisms (microscopic organisms) in environmental samples (including food and water) 2. determination of the effect of pathogenic (disease-causing) microbes on human cells. FACTORS THAT MAY AFFECT THE IMAGE QUALITY Magnification a measure of how much bigger an object appears under the microscope. the number of times the object is enlarged when observed under the microscope Resolution the ability to show two adjacent objects as distinct units. the smallest distance by which two points can be separated and distinguished as individual objects and expressed micrometers (μm) Contrast ability to detect different regions of the specimen on the basis of intensity or color. MAGNIFICATION the apparent increase in size affected by a convex lens. a compound microscope uses two sets of lenses, with differing focal lengths, to facilitate magnification. Total magnification = objective magnification x eyepiece magnification Magnification (total) = magnification (obj. lens) x magnification (ocu. lens) Example: Mag (obj) = 40X ; Mag (ocular) = 10X Mag (total) = (40X) (10X) = 400X OBJECTIVE LENS Scanning Objective Lens (4x) provides the lowest magnification power of all objective lenses. - provide observers with about enough magnification for a good overview of the slide, essentially a “scan” of the slide Low Power Objective (10x) has more magnification power than the scanning objective lens one of the most helpful lenses when it comes to observing and analyzing glass slide samples. giving a closer view of the slide than a scanning objective lens without getting too close for general viewing purposes. High Power Objective Lens (40x) called “high dry” lens ideal for observing fine details within a specimen sample. giving a very detailed picture of the specimen in your slide. Oil Immersion Objective Lens (100x) provides the most powerful magnification, the refractive index of air and glass slide is slightly different, so a special immersion oil must be used to help bridge the gap. Without adding a drop of immersion oil, the oil immersion objective lens will not function correctly, the specimen will appear blurry cedar tree oil is the commonly used oil MAGNIFICATION - If the magnification and the image size are known, the actual size of the object can be determined. Magnification = Image Size ÷ Actual Size Actual Size = Image Size ÷ Magnification Image Size = Actual Size × Magnification Ex. if you are using a microscope at x1200 magnification, and can see a cell that is 50mm wide (50,000μm)*, Actual Size = 50,000 μm / 1200 = 41.6μm RESOLUTION the ability to observe two adjacent objects as distinct and separate objects The resolution can be increased by: using immersion oil reduces the refraction of light rays(through the air in the space between the specimen and the objective lens.) and increases the amount of light that enters the objective lens. only the highest power (100X) objective on the microscope is designed for use with immersion oil. decreasing the wavelength of light that is used to illuminate the specimen use blue light instead of white light RESOLUTION/RESOLVING POWER Resolving Power the limit of resolution Compound light microscope = max. resolution of 0.2 µm it can distinguish between two points ≥ 0.2 µm, any objects closer than 0.2um will be seen as 1 object Optical Instrument Resolving Power RP in Angstrom Human eye 0.2 mm 2,000,000 A° Light Microscope 0.20 µm 2000 A° Scanning Electron Microscope 5-10 nm 50-100 A° Transmission Electron Microscope 0.5 nm 5 A° Resolving Power - the limit of resolution LIMIT OF RESOLUTION/RESOLVING POWER the smallest distance by which two objects can be separated and still be distinguishable or visible as two separate objects. limit of resolution (LR) = wavelength 2 X numerical aperture (NA) Ex. At a wavelength of 550 nm (0.55µm), the 40X objective lens with a N.A. of 0.65. (Visible light has of wavelength from about 400-750nm) LR = 0.55 / 2 x 0.65 = 0.42 µm. OBJECTIVE numerical aperture (NA) Color ring a measure of the light-gathering capacity of Red ring = 4x or 5x the objective. Yellow ring = 10x the higher the numerical aperture, other things Green = 20x being equal, the better the objective is able to Blue = 40x or 50x or 60x separate the details of the specimen in forming White = 100x an image; also the brighter the image. infinity-corrected objective (∞) light rays emerging from such an objective are in parallel bundles projected toward infinity 0.17 refers to the thickness, in millimeters, of the cover glass that was assumed by the lens designer in computing the corrections for the objective. for objectives with a numerical aperture higher than 0.45, a departure in this assumed thickness (or no cover glass at all) may result in the deterioration of the image. PLAN designates an objective that projects, at the eyepiece diaphragm plane, an image which is flat from edge to edge of the field of view PL or NH PL stand for positive low, a phase contrast in which the specimen appears darker than the background of the field of view. the letter NH stand for negative high, a type of phase contrast in which the specimen appears lighter than the background. Oil or oel or WI an immersion objective, requiring a drop of immersion oil (or water) between the front lens of the objective in contact with the cover glass or top of a smear. unless there is oil contact with such an objective, the image will be very poor. some manufacturers inscribe a black ring on the lower part of the barrel to enable the user to instantly recognize the need for oil contact. WI refers to an objective that requires water, rather than oil, as the immersion contact medium. To achieve a numerical aperture of 1.0 or above requires an immersion objective. WI objectives are especially useful in the observation of living biological specimens. EYEPIECE Field of View (FOV) the diaphragm diameter is actually seen through eyepiece the diameter of the circular area you see when you look through the eyepiece of the microscope. FOV (mm) = Eyepiece FN / Objective magnification WF or H WF means widefield; more of the specimen to be seen at a given time. H signifies high eyepoint which means that the user's eyes do not have to be placed very close to the top lens of the eyepiece during observation; a particular boon to eyeglass wearers. WAVELENGTH AND RESOLUTION limit of resolution (LR) = wavelength 2 X numerical aperture (NA) Resolution is directly proportional to the wavelength of the light source and limited by the frequency/wavelength of the light waves generally fitted over the illuminating device below the iris diaphragm. BLUE FILTER helps to increase resolution since its wavelength is the shortest in the visible light spectrum; shorter wavelengths of light provide greater resolution absorb all the light between the yellow and red spectrum thus bringing the temperature of the image much closer to day light if the image appears yellowish with low brightness, blue filter is used The left picture was made without a blue daylight filter, the right one with the filter placed ver the lamp. The filter reduces the red parts of the spectrum. COLOR FILTER generally fitted onto compound microscopes to allow only light waves of certain colors to pass through. the light source will emit light of all 7 colors - violet, indigo, blue, green, yellow, orange, and red. every color will produce an image of different temperatures, contrast, and brightness. block certain colors from passing through by absorption or interference of specific wavelengths. CONTRAST the ability of a detail to stand out against the background or other adjacent details the difference in light intensity between the image and the adjacent background relative to the overall background intensity. high magnification and resolution won’t guarantee that one can actually see the image of the specimen (if all of the light passes through a cell no details will be visible) some light frequencies must be absorbed to different degrees by structures inside the cell and this allows the specimen to be seen a narrow beam provides a higher contrast (adjusting the condenser or diaphragm) or staining the specimen may be necessary to obtain the contrast needed to view the details of the sample. Brightfield microscope Differential interference Phase contrast contrast microscope microscope Taste buds of a rabbit using microscopes with various contrast Although the optical systems found in modern microscopes may be capable of producing high-resolution images at high magnifications, such a capability is worthless without sufficient contrast in the image and the specimen remains essentially invisible, especially unstained or living material. determined by the interaction of the specimen with light (absorption, brightness, reflectance, birefringence, light scattering, diffraction, fluorescence, or color variations) TYPES OF SPECIMEN 1. Amplitude objects 2. Phase objects 3. Reflected light objects/specimen specimens that specimens that do specimen that effectively absorb light partially not absorb light opaque to transmitted or completely illumination thick specimen AMPLITUDE OBJECTS/SPECIMEN absorb light partially or completely, and can thus be readily observed using conventional brightfield microscopy includes naturally colored or stained objects Stains or natural colors absorb some part of the white light passing through and transmit or reflect other colors. Often, stains are combined to yield contrasting colors, e.g. blue hematoxylin stain for cell nuclei combined with pink eosin for cytoplasm. Staining - for specimens that do not readily absorb light, thus rendering such images visible to the eye 1. Eyepiece/Ocular lens: Lens in which the final magnification occurs. Often is at 10X magnification, but can be different (5X, 10X, 15X and 20X) 2. Revolving nose piece: Holds multiple objective lenses in place. The base of the nose piece can rotate, allowing each of the lenses to be rotated into alignment with the ocular lens. 3. Objective lenses: initial magnification of the specimen occurs here. most brightfield light microscopes have 3 objective lenses seated into the resolving nose piece base. Low power objective (10x) High dry objective (45x) Oil immersion objective (100x) 1. Low Objective: Magnification: has a lower magnification power (2x to 10x). provides a wide field of view, allowing one to see a larger area of the specimen. Field of View: the area visible through the microscope is relatively large helpful for getting an overall view of the specimen, especially when one need to locate specific areas of interest. Working Distance: the space between the objective lens and the specimen is usually greater making it easier to manipulate the specimen or perform tasks like focusing and staining. Resolution: while the low objective provides a wide view, it may have lower resolution compared to higher magnification objectives. This means that fine details may not be as clear. Common Use: low objective lenses are commonly used for initial specimen observation, finding areas of interest, and for a general overview. ideal for locating specific structures or regions before switching to higher magnification objectives. 2. High Objective: Magnification: have a higher magnification power (20x to 100x or more). used to view fine details and structures not visible at lower magnifications. Field of View: the field of view is narrower with high objectives due to the higher magnification. one see a smaller area but with greater detail. Working Distance: much shorter with high objectives. - It is challenging to manipulate the specimen or apply techniques like staining. Resolution: offer higher resolution, allowing one to see fine structures and details with greater clarity. Common Use: used for detailed examination of specific areas of interest within the specimen. crucial for tasks like identifying cellular structures, counting cells, and studying fine morphological features. 4. Coarse focusing knob: larger of the two 5. Fine focusing knob: smaller of the two knobs, the coarse adjustment knob moves the knobs, the fine adjustment knob brings the stage up or down to bring the specimen into specimen into sharp focus under low power and focus. It is very sensitive, even small partial is used for all focusing when using high-power rotation of this knob can bring about a big lenses such as the 100x oil immersion lens change in the vertical movement of the stage. Note: ONLY use coarse focusing at the 6/9. Stage & Mechanical stage: beginning with the 4X, 10X low powered the horizontal surface where the slide objectives in place. If you use it with the higher specimen is placed (stage). powered objectives, it can damage the the slide is held in place by spring-loaded objective if you crash the lens through your clips and moved around the stage by glass specimen slide turning the geared knobs on the mechanical stage. 7. Illuminator: contains the light source, a has two perpendicular scales that can be lamp made either of an incandescent tungsten- used to record the position of an object on halogen bulb or an LED. There is normally a a slide, useful to quickly relocate an object. switch to turn on/off or a rheostat located on the side that you can use to adjust the brightness of the light 8. Diaphragm/Iris and Condenser: the diaphragm controls the amount of light passing from the illuminator through the bottom of the slide, there is a small lever used to achieve the optimal lighting. The condenser is a lens system that focuses the light coming up from the illuminator onto objects on the slide. REFRACTIVE INDEX (R.I) - affects the resolving power of the microscope the capacity/power of light to bend while passing through air from the glass slide to the objective lens. The RI of air is lower than that of glass. When the light rays pass from the glass into the air, they bent or refract so that they do not pass into the objective lens. Loss of refracted light can be compensated by mineral oil which has the same refractive index as glass. The loss of light reduces the numerical aperture and resolving power of the objective lens. The decrease in light refraction produces more light rays which enter directly into the objective lens, producing a clear image with high resolution. MICROSCOPIC TECHNIQUES 1. Light Microscopy 1. Scanning Tunneling Microscopy (STM) 2. Flourescence Microscopy 2. Super-resolution Microscopy 3. Confocal Microscopy 3. Cryo-Electron Microscopy 4. Electron Microscopy 4. Digital Holographic Microscopy 5. Atomic Force Microscopy (AFM) 5. Raman Microscopy HOW MICROSCOPY WORKS: Visible light consists of electromagnetic waves Amplitude – the height of a wave, indicator of brightness of light Wavelength (λ) – the distance between two peaks. Frequency (v) - the number of waves that pass a fixed point in unit time INTERACTIONS OF LIGHT Transmission – light passes through an object Light waves interact with Reflection – light bounces back from an object materials by being transmitted, Refraction – light bends when it hit the object reflected, refracted, diffracted, Diffraction – light spreading out when it passes absorbed or scattered. an aperture (gap/hole) Absorption – light is captures by the object Scattering – light is scattered in different direction Change of speed REFRACTION different transparent materials transmit light the bending of light at different speeds; thus, light can change the most important behavior exhibited speed when passing from one material to by light waves another. occurs when light waves change the change in speed usually also causes a direction as they enter a new medium change in direction (refraction), with the degree of change dependent on the angle REFRACTIVE INDEX (n) of the incoming light a measure of how much the speed of light changes when it passes through that material compared to Refractive index (n) of common its speed in a vacuum. medium the ratio of the speed of light in a vacuum to its Medium n speed in a specific medium. Air 1.0 n=c/v Water 1.333 c - speed of light in a vacuum (3 x 108 m/s) Glass 1.47 – 1.60 v – velocity of light in a medium Oil 1.40 – 1.55 The slower the speed of light, the higher the refractive index and the higher the optical density. If medium 2 is denser than medium 1 If medium 2 is less dense than medium 1 The wave slows down The wave speeds up The wave bends towards the normal The wave bends away from the normal The ∠ of refraction < the ∠ of incidence The ∠ of refraction > the ∠ of incidence FUNCTION OF OIL IN OIL IMMERSION OBJECTIVE Higher magnification lens bends the light more and needs to be brought to the specimen closer a) Oil immersion lenses are used to improve resolution because immersion oil and glass have very similar refractive indices, thus there is a minimal amount of refraction before the light reaches the lens. b) Without immersion oil, light scatters as it passes through the air above the slide, degrading the resolution of the image. TYPES OF LENSES Convex lens Concave light light passing through is refracted toward light passing through is refracted away a focal point on the other side of the from a focal point in front of the lens lens. HOW MICROSCOPE WORKS Condenser - a lens that gathers light and focuses it into a cone directed at the specimen. Diaphragm controls the diameter of the light beam before it finally passes into the specimen. It control the amount of light that passed through the specimen Objective lenses gather and focus the light transmitted from the specimen. DIAPHRAGM control the width of the light beam passing through to the specimen, thereby determining how much of the specimen is being illuminated TYPES OF MICROSCOPE Electron Microscope Light microscope a system of electromagnetic lenses and a magnification is obtained by a system short beam of electrons are used to obtain of optical lenses using light waves. magnification (e.g. Transmission electron (e.g. Bright field, Dark field, microscope (TEM) and Scanning electron Fluorescence, Phase contrast and UV microscope (SEM). Microscope) Compound Microscope TYPES OF LIGHT MICROSCOPE employs two separate lens systems, the objective Simple Microscope and the ocular (eye piece). consists of only one bi-convex lens along can magnify images up to 2000X. It has a with a stage to keep the specimen. higher magnification as it uses two sets of can magnify an image up to 300X. It has lenses. low magnification as it uses a single lens. with resolution of about 0.2 µm. - the resolution is below 1 µm. can be monocular (1 eyepiece) or binocular (2 eyepieces) MICROSCOPIC TECHNIQUES Light Microscopy employs visible light to detect small objects Bright Field the most basic form of light microscopy, where specimens are viewed against a bright background. two lens system are used for magnification suitable for observing stained (prepare slides) or naturally pigmented samples. Limitations: living cells cannot be observed due to the absence of contrast between the specimen and the surrounding medium. limited by weakly absorbing samples b. Dark Field Microscopy the entire field appears dark when there is no sample on the microscope stage used to illuminate unstained samples causing them to appear brightly lit against a dark background has a special condenser that scatters light and causes it to reflect off the specimen at an angle blocking most of the light that ordinarily passes through and around the specimen, allowing only oblique rays (light is projected at the specimen from a sideways) to interact with the specimen best for revealing outlines, edges, boundaries, and refractive index gradients. Ideal candidates for darkfield illumination include minute living aquatic organisms, diatoms, small insects, bone, fibers, hair, unstained bacteria, yeast, cells in tissue culture, and protozoa. an opaque disc is placed underneath the condenser lens, so that only light that is scattered by objects on the slide can reach the eye. instead of coming up through the specimen, the light is reflected by particles on the slide. - everything is visible regardless of color, usually bright white against a dark background. pigmented objects are often seen in "false colors," that is, the reflected light is of a color different than the color of the object. BRIGHT FIELD VS DARK FIELD a. Brightfield b. Darkfield c. Darkfield with red filter silica skeletons from a small marine protozoan (radiolarian) in a whole mount specimen LIGHT MICROSCOPY c. Phase Contrast developed by Dutch physicist Frits Zernike in the 1930s used to enhance the visibility of transparent or semi- transparent specimens by exploiting differences in phase (i.e., differences in the speed of light) caused by variations in specimen thickness preferable to bright field microscopy when high magnifications (400x, 1000x) are needed and the specimen is colorless/transparent (phase objects- specimen that do not absorb light) or the details are so fine that color does not show up well. most living microscopic organisms are much more obvious in phase contrast. living cells can be examined in their natural state without previously being killed, fixed, and stained. Phase Contrast/Phase Plate Microscopy a. Phase Shift: When light passes through a transparent specimen, it experiences a phase shift due to differences in the refractive index of various parts of the specimen. Refractive index is a measure of how much light is bent as it passes through a material. b. Phase Plate: In a phase-contrast microscope, a phase plate is inserted into the optical path of the microscope. This phase plate causes a controlled phase shift in the light passing through it. This phase difference is turned into an intensity difference (thick parts of the specimen appears darker while thin parts appear brighter) a) Organelles are nearly invisible in the bright field although they have different refractive indexes b) Light is bent and retarded more by objects with a high refractive index; c) In phase contrast a phase plate is placed in the light path. Barely refracted light passes through the center of the plate and is not retarded. Highly refracted light passes through the plate farther from the center and is held back another onequarter wavelength.; d) The microscope field shows a darker background (in this case the cell cytoplasm has a higher refractive index than the contractile vacuole), with the organelles in sharp contrast. Phase Contrast Microscopy c. Interference: Light that has passed through the specimen and the phase plate encounters another beam of light that has not interacted with the specimen (2 light beams). These two beams of light interfere with each other, creating areas of constructive and destructive interference. d. Enhanced Contrast: The interference patterns produce variations in brightness in the final image. regions of the specimen that caused a significant phase shift (e.g., thick parts of a cell) appear darker, while regions with little or no phase shift (e.g., thin parts or background) appear brighter. This enhanced contrast allows for better visualization of internal cellular structures, such as cell nuclei, organelles, and other transparent elements PHASE PLATE speeds up the unrefracted light by ¼ λ Note: amplitudes are the same between the difference in wavelength between the unrefracted and refracted rays, however direct and deviated light for a phase refracted rays are delayed by ¼ λ specimen would now be 1/2 wavelength INTERFERENCE OF LIGHT Observed when two waves are combined The resultant wave may have greater intensity/amplitude (in phase/constructive) or lower intensity /amplitude (out of phase/destructive) Undeviated and diffracted light collected by the objective is segregated at the rear focal plane by a phase plate. A reduction in the brightness of the object is observed. The degree of reduction in brightness depends on the refractive index of the object. Lights pass through undeviated or are diffracted and retarded in phase by structures and phase gradients present in the specimen Wavefronts pass through the condenser annulus (instead of diaphragm) and illuminate the specimen A comparison of the diagrams (on the right) illustrates that extra delay enhances the interference obtained when the unrefracted (U) and refracted (R) rays are focused back together (U+R). PHASE CONTRAST - “converting” phase specimens to amplitude specimens 1. Light passes through the condenser annulus. 2. Light experiences refraction when passes through the specimen, with a degree of refraction depending on the thickness of the specimen, resulting to a ¼ λ delayed. LIMITATIONS OF PHASE CONTRAST 3. The refracted and unrefracted light passes through the phase plate, wherein 1. Phase contrast images often have halos the unrefracted light is speed up by ¼ λ, surrounding the outline of details that have a making a total difference of 1/2 λ high phase shift (thick specimen). These halos between the refracted and unrefracted are optical artifacts and can make it hard to light. see the boundaries of details. 4. The refracted and unrefracted are 2. Phase contrast doesn’t work well with thick combined and the difference in λ results specimens as these can appear distorted. to destructive interference. LIGHT MICROSCOPY commonly used for a specimen that is colorless/transparent and relatively thick d. Differential Interference Contrast Images produced in differential interference (DIC)/Nomarski microscopy contrast microscopy have a distinctive developed by Georges Nomarski in 1952 as an shadow-cast appearance improvement over phase contrast microscopy unlike phase contrast, DIC has no halo effect and it can be used to produce very clear images of thick specimens. provide 3-D image of a specimen use of polarizer and a prism to split light into two rays (waves) DIFFERENCTIAL INTERFERENCE CONTRAST 1. Light passes through the polarizer 2. Polarized light is split into two beams 3. Two beams pass through the sample and changes speed based on the sample thickness 4. The beams recombine and undergo interference (with constructive interference, the image appears brighter while with destructive interference, the image appears darker) 1. Light from an incandescent source is passed through a polarizer, so that all of the light getting through must vibrate in a single plane. 2. The beam is then passed through a prism that separates it into components that are separated by a very small distance - equal to the resolution of the objective lens. 3. The beams pass through the condenser, then the specimen 4. The two beams are delayed or refracted differently in any part of the specimen in which adjacent regions differ in refractive index 5. Recombined by a second prism in the objective lens, the differences in brightness correspond to differences in RI or thickness in the specimen FLUORESCENCE MICROSCOPY Luminescence uses fluorescence and the emission of light by a substance that has not phosphorescence instead of, or in been heated addition to, reflection and the energy released by a substance in the form of absorption. light a. relies on the property of certain Photoluminescence - caused by absorption of light molecules to absorb shorter Fluorescence – short-lived, emission of light wavelength (higher E) (UV /blue light) stops as soon as the light source is switched off light and emit light of a longer (e.g. fluorescent bulb) wavelength (lower E level) Phosphorescence – long-lived, emission of light continuous even after the light source is switched off (e.g. glow in the dark) b. Chemiluminescence - a result of a chemical reaction (e.g. glow stick) c. Bioluminescence - due to enzymatic reaction (e.g. firefly) This process occurs in steps:. 1. Absorption: An atom or molecule absorbs an incoming photon. Usually, this is visible or ultraviolet light because x-rays and other energetic radiation are more likely to break chemical bonds than get absorbed. 2. Excitation: The photons boost the atoms or molecules into a higher energy level, which is called an excited state. 3. Excited State Lifetime: The molecules do not remain excited for long. They immediately begin to decay from the excited state toward a relaxed state. But, there may be smaller energy drops from within the excited state called non-radiative transitions. 4. Emission: The molecule drops all the way to one of the ground states, emitting a photon. The photon has a longer wavelength (less energy) than the absorbed photon. PRINCIPLES Fluorescent dyes (fluorophores or Most cellular components are colorless and fluorochromes) are molecules that absorb cannot be clearly distinguished under a excitation light at a given wavelength microscope. The basic premise of fluorescence (generally UV), and after a short delay emit microscopy is to stain the components with light at a longer wavelength. The delay dyes. between absorption and emission is negligible, generally on the order of nanoseconds. COMMONLY USED FLUORESCENCE DYE 1. Fluorescein Isothiocyanate (FITC): 1.. DAPI (4',6-diamidino-2-phenylindole): a. Excitation/Emission Wavelength: 488 nm / a. Excitation/Emission Wavelength: 358 nm / 520 nm 461 nm b. Applications: DAPI binds to DNA and is b. Applications: used to label antibodies and commonly used for nuclear staining in fixed proteins in immunofluorescence assays. It is cells. It helps visualize the cell nucleus in blue also used in cell viability assays, cell tracking, fluorescence. and DNA labeling. 2. GFP (Green Fluorescent Protein): 2. Rhodamine (Rhodamine 123): a. Excitation/Emission Wavelength: 488 nm / a. Excitation/Emission Wavelength: 532 nm / 507 nm b. Applications: GFP is a genetically encoded 560 nm fluorophore used for labeling specific b. Applications: used to label mitochondria, proteins and tracking their localization in live actin filaments, and cell membranes. cells. It is widely used in molecular and cell 3. Texas Red: biology research. a. Excitation/Emission Wavelength: 595 nm / 3. Propidium Iodide (PI) 615 nm a. Excitation/Emission Wavelength: 493 nm / b. Applications: for nucleic acid labeling, flow 636 nm b. Applications: used to stain cells and nucleic cytometry, and multicolor imaging when acids combined with other fluorophores. Fluorescence microscopy uses a much higher intensity light to illuminate the sample. This light excites fluorescence species in the sample, which then emits light of a longer wavelength. The image produced is based on the emission wavelength of the fluorescent species rather than from the light originally used to illuminate, and excite, the sample. PARTS OF A FLUORESCENCE MICROSCOPE 2. Excitation filter only allows to pass the wavelengths absorbed 1. Light source by the fluorophore, thus minimizing the Four main types of light sources are excitation of other sources of fluorescence used, including xenon arc lamps or selects the wavelengths of the light from the mercury-vapor lamps with an excitation light source to match the fluorophore’s filter, lasers, and high-power LEDs. absorbance spectrumstly used for complex Lasers are mostly used for complex fluorescence microscopy techniques, while fluorescence microscopy techniques, xenon lamps, and mercury lamps, and LEDs while xenon lamps, and mercury lamps, with a dichroic excitation filter are commonly and LEDs with a dichroic excitation used for wide-field epifluorescence filter are commonly used for wide-field microscopes. epifluorescence microscopes. Note: If a single-wavelength LED light source is used, the excitation filter can be omitted. 3. Dichroic mirror/thin-film filter a very accurate color filter used to 4. Emission filter selectively pass light of a small The emitter is typically a bandpass filter that range of colors while reflecting other passes only the wavelengths emitted by the colors. fluorophore and blocks all undesired light outside reflects the selected excitation light this band – especially the excitation light. towards the sample, and transmits By blocking unwanted excitation energy the longer wavelengths emitted from (including UV and IR) or sample and system the sample. autofluorescence, optical filters ensure the to distinguish excitation light and darkest background. emission light selects only the wavelengths corresponding to the fluorophore’s emission profile, while also increasing contrast by blocking autofluorescence, stray light from the room or reflected light Note: If there is only one kind of fluorescence in the sample and no auto-fluorescence interference, the emission filter can be omitted.) APPLICATIONS OF FLUORESCENCE MICROSCOPY to identify structures in fixed and live biological samples. allows the use of multicolor staining, labeling of structures within cells, and the measurement of the physiological state of a cell. Advantage: Different molecules can now be stained with different colors, allowing multiple types of the molecules to be tracked simultaneously. ADVANTAGES OF FLUORESCENCE MICROSCOPY High Sensitivity: Fluorescence microscopy can detect extremely low concentrations of fluorescent molecules, making it ideal for studying rare or weakly emitting samples. Specific Labeling: Fluorophores can be chemically attached to specific molecules (e.g., antibodies binding to proteins) for precise targeting and visualization. Multicolor Imaging: Different fluorophores can be used simultaneously with distinct emission spectra, enabling the study of multiple components within a sample. Different molecules can now be stained with different colors, allowing multiple types of the molecules to be tracked simultaneously LIMITATIONS OF FLUORESCENCE MICROSCOPY Photobleaching: Fluorophores can degrade over time due to exposure to light. Phototoxicity: High-intensity illumination can harm living samples. Unlike transmitted and reflected light microscopy techniques fluorescence microscopy only allows observation of the specific structures which have been labeled for fluorescence TYPES OF FLUORESCENCE MICROSCOPY Widefield Fluorescence Microscopy: Illuminates the entire sample at once and is suitable for fast imaging. Confocal Microscopy: Uses a pinhole to eliminate out-of-focus light, providing optical sectioning and improved resolution; laser scan a particular point of the specimen (illumination point); generates 3D image Total Internal Reflection Fluorescence Microscopy (TIRF): Illuminates only the nearsurface region, ideal for studying processes at cell membranes. Advantages of low photobleaching, and low photodamage Super-Resolution Microscopy: Techniques like Stimulated Emission Depletion Microscopy (STED), Structured Illumination Microscopy (SIM), and Photoactivated Localization Microscopy (PALM) can achieve resolutions beyond the diffraction limit, allowing researchers to see cellular structures at nanometer scales ELECTRON MICROSCOPY uses a beam of accelerated electrons as an illumination source instead of light. the wavelength of an electron can be up to 100,000 times shorter than that of visible light photons, therefore it has a higher resolving power than light microscopes and can reveal the structure of smaller objects. when the high-energy electrons strike the specimen, they interact with its atoms. These interactions include scattering, absorption, and transmission of electrons through the specimen. the interactions result in the loss of energy by the electrons and the emission of various signals that are used to create the image ADVANTAGES OF ELECTRON MICROSCOPY DISADVANTAGES OF ELECTRON MICROSCOPY 1. Magnification and higher resolution – as 1. Live specimens cannot be analyzed as electrons rather than light waves are used, electrons are easily scattered by other it can be used to analyze structures that molecules in the air, thus samples must be cannot otherwise be seen. The resolution analyzed in a vacuum. of electron microscopy images is in the 2. Only produce black and white images. range of up to 0.2 nm, which is 1000x 3. Presence of artefacts which are left more detailed than light microscopy. over from sample preparation and require 2. Produce highly detailed images of specialized knowledge of sample structures which are of a high quality, preparation techniques to avoid. revealing complex and delicate structures 4. Highly expensive that other techniques may struggle to 5. Bulky reproduce. 6. Requires years of training to conduct ELECTRON MICROSCOPY - uses an electron beam as an illumination source instead of light. a. Transmission Electron Microscope (TEM) b. Scanning Electron Microscope (SEM) c. Scanning Transmission Electron Microscope (STEM) combination of TEM and SEM d. Cryo-Electron Microscopy (Cryo-EM) samples are rapidly frozen in vitreous ice to preserve their natural structure and then imaged with an electron microscope at cryogenic temperatures. MAJOR TYPES OF ELECTRON MICROSCOPY Scanning Electron Microscopy (SEM) Transmission Electron Microscopy (TEM), the a beam of electrons moves back and forth sample is cut into extremely thin slices before across the surface of a cell or tissue, imaging, and the electron beam passes through creating a detailed image of the 3D surface. the slice rather than skimming over its surface. TEM is often used to obtain detailed images of the (TEM VERSUS SEM) internal structures of cells. LIGHT MICROSCOPY VS ELECTRON DIGITAL HOLOGRAPHIC MICROSCOPY (DHM) TYPES OF DHM combines digital holography with Transmission DHM measures the microscopy to allow transparent cells to be difference in the optical path of a beam visualized with classic cell culture plates that travels through the sample being produces a digital hologram from the light analyzed. This type of microscopy is often waves that have passed by the specimen. A useful when measuring micro-optical numerical reconstruction algorithm in a components, microfluidic devices, and computer is used to create the hologram. defects inside transparent samples. originally invented as a means to expand upon the uses of electron microscopy. Reflection DHM creates an image based a phase contrast microscopy technique on the reflected wavefront from the that uses the interference of light waves to sample. This provides a view of the create an image topography of the sample surface by way of reflection PRINCIPLES OF DHM ADVANTAGES OF DHM Holography uses the concept that light waves create interference patterns similar to those Static and dynamic 3D characterization of created by water waves. sample structures to be determined, even Two beams of laser light are used to create a for transparent objects such as living hologram. One beam, called the sample beam, biological cells illuminates the sample, while the other, known Auto digital focus allowing for very quick as the reference beam, does not pass through scanning of surfaces, without the need to the sample. vertical mechanical movement that is Depending on the type of DHM being used, the required by other types of microscopy to sample will imprint the sample beam through focus on the subject. either reflection or transmission. The sample Relatively inexpensive due to fewer lenses beam and the reference beam then come and objectives needed together again to create an interference Non-invasively image living cells, and study pattern that enables the sample imprint to be dynamic processes at the nanoscale. recorded in the hologram SCANNING TUNNELING MICROSCOPY (STM) based on the scanning of a sharp (1-10 nm) probe that is electrically conductive just above the surface of an electrically conductive sample. the principle of STM is based on the tunneling of electrons between this conductive sharp probe and the sample. SCANNING TUNNELING MICROSCOPY (STM) it relies on the quantum mechanical tunneling of electrons between the tip and the sample. The tip is brought very close to the sample surface, and a voltage is applied between them. Electrons can tunnel through the vacuum gap between the tip and sample, and the tunneling current is measured. Changes in the current as the tip scans the surface are used to create images. TUNNELING a phenomenon that describes how electrons flow (or tunnel) across two objects of differing electric potentials when they are brought into close proximity to each other. When a voltage is applied between the probe and the surface, electrons will flow across the gap (probe -sample distance) generating a measurable current. the amplitude of the current exhibits an exponential decay with the distance (d) very small changes in the probe-sample separation induce large changes in the tunneling current the probe in SPM does not make direct contact with the surface. Constant Current A constant tunneling current is maintained during scanning (typically 1 nA). This is done by vertically (z) moving the probe at each (x,y) data point until a “setpoint” current is reached. The vertical position of the probe at each (x,y) data point is stored by the computer to form the topographic image of the sample surface. This method is most common in STM. Constant Height In this approach the probe-sample distance is fixed. A variation in tunneling current forms the image. This approaching allows for faster imaging, but only works for flat samples ADVANTAGES High Spatial Resolution: Non-destructive Imaging: Versatility: AFM can achieve nanometer- AFM is non-destructive and AFM can be used to level spatial resolution, non-invasive. It does not investigate a wide range of allowing researchers to require special sample samples, including solid visualize and study the surface preparation techniques, such surfaces, polymers, morphology and structures of as staining or coating, and can biomolecules, nanoparticles, materials, molecules, and be used to study samples in and more. It is applicable to biological specimens at the their natural state, including both conducting and non- atomic and molecular scale. biological cells and tissues. conducting materials. ADVANTAGES 3D Imaging: Environmental Control: Unlike many other microscopy techniques, AFM can be used under different AFM provides three-dimensional imaging environmental conditions, including in air, capabilities. It can generate topographic liquid, and vacuum environments, making it maps of sample surfaces, allowing suitable for a wide range of sample types researchers to study features like height and experimental conditions. variations, roughness, and defects. LIMITATIONS 1. Complex and expensive instrumentation - especially (UHV) version 2. Subject to noise (electrical, vibration) 3. Must fabricate probes - dull probes or multiple tips at the end of probe can create serious artifacts 4. Only works for conductive samples: metals, semiconductors ATOMIC FORCE MICROSCOPY (AFM) / SCANNING FORCE MICROSCOPY (SFM) it generates images by scanning a small cantilever over the surface of a sample. The sharp tip on the end of the cantilever contacts the surface, bending the cantilever and changing the amount of laser light reflected into the photodiode. The height of the cantilever is then adjusted to restore the response signal, resulting in the measured cantilever height tracing the surface. it operates by scanning a sharp probe tip, usually at the end of a cantilever, over the surface of a sample. The interaction forces between the tip and the sample, including van der Waals forces and electrostatic forces, cause the cantilever to deflect. This deflection is measured to create a topographic map of the sample surface AFM VS STM Sample Types: Sample Preparation: AFM: AFM can be used to image a wide AFM: Sample preparation for AFM is range of samples, including conductive and relatively straightforward. It does not require non-conductive materials, biological special sample conductivity or coating. samples, and insulators. It is not limited to STM: STM requires the sample to be conducting surfaces. conductive or semiconductive. Sample STM: STM is primarily used on conducting or preparation can be more challenging, often semiconducting materials. It relies on the involving processes like cleaving a crystal or flow of electrons, which is limited to depositing a conductive layer conductive materials. AFM VS STM Environmental Conditions: - both powerful techniques for imaging AFM: AFM can operate in various surfaces and studying materials at the environments, including air, liquid, and atomic and molecular scales. vacuum, making it versatile for studying AFM and STM are complementary different types of samples. techniques, with AFM providing STM: STM is typically operated in ultrahigh highresolution topographic information and vacuum conditions due to the need for a STM revealing electronic properties vacuum gap for electron tunneling.