Thermodynamics Notes PDF
Document Details
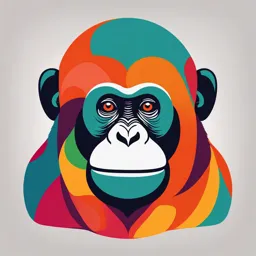
Uploaded by ThrillingTsilaisite
UAEU College of Medicine and Health Sciences
Farah Mustafa
Tags
Summary
These notes detail thermodynamics, covering definitions, forms of energy (kinetic and potential), and associated concepts. The document also includes a lecture outline, outlining topics such as laws of thermodynamics, enthalpy, entropy, and free energy.
Full Transcript
Thermodynamics Farah Mustafa Department of Biochemistry Rm: IE-179; Office: 03-713-7509 Mobile: 050-760-5516 Definition of Thermodynamics • Greek: thermos = heat; dynamic = change • Study of the relationship between energy, heat, and work. Thermodynamics • All living organisms require energy En...
Thermodynamics Farah Mustafa Department of Biochemistry Rm: IE-179; Office: 03-713-7509 Mobile: 050-760-5516 Definition of Thermodynamics • Greek: thermos = heat; dynamic = change • Study of the relationship between energy, heat, and work. Thermodynamics • All living organisms require energy Energy • Energy is defined as the ability to do work • Heat is energy in transfer between a system and its surroundings other than by work • Thermodynamics is the study of: laws that govern the conversion of energy from one form to another The direction in which heat will flow and The availability of energy to do work Work Heat Lecture Outline • • • • • Definition of Thermodynamics Forms of Energy: Potential and Kinetic Energy Conversion Thermodynamic Parameters and Systems Laws of Thermodynamics First Law: Enthalpy and Hess’s Law Second Law: Entropy and Order • Gibb’s Free Energy • Exothermic and Endothermic Reactions • Favorable and Unfavorable Reactions Forms of Energy • Found in different forms, such as light, heat, sound and motion, etc. • There are many forms of energy, but they can all be put into two categories: 1. Kinetic 2. Potential Kinetic verses Potential Energy • Kinetic energy. Energy in action, energy of motion––of waves, electrons, atoms, molecules, substances, objects, etc. • Potential energy is stored energy and the energy of position. Forms of Kinetic and Potential Energy Form of Energy Kinetic/Potential Object in Motion /Stored Energy Heat Kinetic Atoms/Molecules Chemical Potential Chemical Bonds Electrical Kinetic Electrons/Ions Gravitational Potential Gravity Optical (Radiant) Kinetic Photons Electrostatic Potential Coulomb Wind Kinetic Atoms/Molecules Nuclear Potential Nuclear binding force http://chemistry.osu.edu/~woodward/ch121/ch5_work.htm Energy Conversion • Potential energy can be converted to kinetic energy • It is kinetic energy that does work Thermodynamics Systems • A Thermodynamic System is that part of the universe that is under consideration • A real or imaginary boundary separates the system from the rest of the universe, which is referred to as the surrounding System: the process/reaction whose thermodynamic change is being studied Surrounding: the part of the universe that interacts with the system. Thermodynamic Potential • The Thermodynamic Parameters define the thermodynamic potential of a system. Enthalpy Entropy Free Energy • The Laws of Thermodynamics deal with these parameters Thermodynamic Parameters • Some commonly considered parameters are: Mechanical parameters: Pressure: p Volume: V Thermodynamic parameters: Temperature: T Enthalpy: H Entropy: S First Law of Thermodynamics • Conservation of Energy Energy cannot be created or destroyed, merely transformed from one form to another • Therefore, for any physical or chemical change, the total amount of energy in the universe should remains constant • Energy may change form or it may be transferred from one region to another, but it cannot be created or destroyed Enthalpy (H) • Enthalpy = Heat content (H) • Is property of a system that reflects its capacity to exchange heat with the surrounding • The total enthalpy of a system cannot be measured directly • However, the enthalpy change of a system can be measured • Enthalpy change is defined by the following equation: ΔH = H final –H initial If Hf > Hi ΔH + If Hf < Hi ΔH - Directionality of Heat Transfer • Heat always transfers from hotter object to cooler one. • EXOthermic: heat transfers from SYSTEM to SURROUNDINGS T(system) goes down T(surr) goes up • Heat always transfers from hotter object to cooler one. • ENDOthermic: heat transfers from SURROUNDINGS to the SYSTEM. T(system) goes up T (surr) goes down Joule: Unit of Energy, Work, or Amount of Heat • 1 calorie = Heat required to raise the temperature of 1 gm of H2O by 1 oC • 1000 cal = 1 kilocalorie = 1 kcal • 1 kcal = 1 Calorie (a food “calorie”) • The SI unit for energy, work, or heat is Joule 1 cal = 4.184 joules James P. Joule 1818-1889 Hess's Law • If a reaction is carried out in a series of steps, ΔH for the reaction will be equal to the sum of the enthalpy changes for the individual steps. • It is often possible to calculate ΔH of a reaction from listed ΔH values of other reactions. CH4(g) + 2O2(g) 2H2O(g) CH4(g) + 2O2 (g) CO2(g) + 2H2O(g) 2H2O(l) CO2 (g) + 2H2O(l) ΔH = -802 kJ ΔH = -88 kJ ΔH = -890 kJ Second Law of Thermodynamics The Entropy Lab http://www.cartoonstock.com/directory/t/thermodynamics.asp Second Law of Thermodynamics • The entropy, S, of the universe increases (real, spontaneous processes). • Thus, highly ordered systems have low entropy and with time, they move towards more disorder (more entropy) • The more random the distribution of molecules, the greater the entropy • Which has the greatest entropy? Solids, liquids, or gasses S gas> S liquid> S solid Entropy • Entropy can be thought of as a measure of the randomness of a system. • It is related to the various modes of motion in molecules. • Like total energy, E, and enthalpy, H, entropy is a state function. • Therefore, S = Sfinal Sinitial Entropy on the Molecular Scale Implications: • more particles -> more states • higher T -> more energy states • less structure (gas vs solid) -> more states -> more entropy -> more entropy -> more entropy Entropy on the Molecular Scale • What happens to entropy when you increase: Temperature Volume (gases) The number of independently moving molecules • Increase in these factors increases entropy Entropy Changes • In general, entropy increases with the freedom of motion of molecules. For e.g., when – the number of gas molecules increase – The number of moles of a substance increase Predict the Sign of ΔS Predict the sign of S PROCESS ice -> liquid water water vapor -> liquid water cold water -> hot water 3 H2(g) + N2(g) -> 2 NH3(g) pressure of a gas increases volume of a gas increases S ? ? ? ? ? ? Entropy Change in the Universe • The universe is composed of the system and the surroundings. Suniverse = Ssystem + Ssurroundings • Entropy change for a process: Suniv = Ssys + Ssurr > 0 process is spontaneous Suniv = Ssys + Ssurr = 0 process is at equilibrium Suniv = Ssys + Ssurr < 0 process is non-spontaneous Thermodynamic Free Energy • It is the maximum amount of chemical energy derived from a spontaneous reaction that can be utilized to do work or to drive a nonspontaneous process. Or • It is the minimum amount of energy that must be supplied to make a non-spontaneous reaction occur. Gibbs Free Energy • The amount of energy a system has available to do useful work is called the Gibbs Free Energy (G) • This change is symbolized as ΔG: ΔG = ΔH - TΔS • Predicts the direction of a spontaneous reaction • By knowing the sign (+ or -) of S and H, one can get the sign of G and determine if a reaction is spontaneous Reactions Exothermic or Negative ΔG Negative lies G Equilibrium towards completion Endothermic or Positive ΔG Positivewill G Reaction not occur without energy input Gibbs Free Energy 1. If G is negative, the forward reaction is spontaneous. 2. If G is 0, the system is at equilibrium. 3. If G is positive, the reaction is spontaneous in the reverse direction. Characteristics of Reactions Exothermis Reactions Endothermic Reactions • ΔG is negative • ΔG is positive • The final state has less energy than the starting state • The final state has more energy than the starting state • Energy is released Enthalpy Decreases • Energy is absorbed Enthalpy Increases • The process occurs spontaneously, although it may occur slowly • Non-spontaneous. Without energy input, the process will not occur • Often the starting state (reactants) is more ordered and complex than the final state (products) Entropy Increases • Often the final state (products) is more ordered and complex than the starting state (reactants) Entropy Decreases • In living systems, exergonic processes often decrease order or release potential energy • Living systems generally use endergonic processes to do work and to maintain order • Some energy is lost as heat • Some energy is lost as heat Types of Work Done by Cells • Chemical Work – Anabolism – The formation of many molecules needed by the cell requires energy. • Mechanical Work – Change physical locations of organisms, cells and internal structures • Transport Work – Movement of molecules and ions into and out of cells against electrical and concentration gradients Thermodynamics Laws Apply to Biological Systems Energy Used by Living Things • Energy is stored in the chemical bonds of biological molecules. E.g., glucose catabolism (exothermic) • Stored energy is released so work can be accomplished. E.g., glucose anabolism (endothermic) 2nd Law Application • Increase in Entropy, i.e. – Useful energy is lost when energy changes forms Energetically Favorable and Unfavorable Reactions An Unfavorable Metabolic Reaction is Driven by a Favorable One A B G = +10kJ/mol (Endergonic reaction) C D G = -20kJ/mol (Exergonic reaction) A+C B + D G = -10kJ/mol (coupling reaction) Example Glucose + Pi ATP + H2O Glucose + ATP Glucose-6-phosphate ADP+ Pi G = +14kJ/mol G = -31kJ/mol Glucose-6-phosphate G = -17kJ/mol