Comparative Study of the Effect of Cross-linking Degree on Chitosan Hydrogels PDF
Document Details
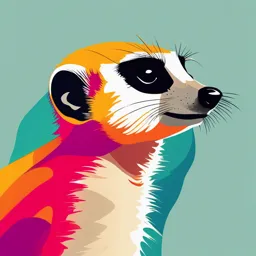
Uploaded by pepinos
Yalova University
Hanife Songül Kaçoğlu,Özgür Ceylan,Mithat Çelebi
Tags
Related
- Hydrogel Properties and Applications PDF
- Kinetics, Absorption, and Diffusion of Crosslinked Chitosan Hydrogels PDF
- Potassium Release Kinetics & Water Retention of Controlled-Release Fertilizers PDF
- Temperature-Sensitive Poly(N-isopropylacrylamide)-Chitosan Hydrogel for Fluorescence Sensors (PDF)
- Kinetics, Absorption, and Diffusion Mechanism of Crosslinked Chitosan Hydrogels PDF
- Synthesis and Evaluation of pH- and Temperature-Responsive Chitosan-p(MAA-co-NIPAM) Hydrogels PDF
Summary
This research article investigates the effect of cross-linking degree on chitosan hydrogels synthesized with low and medium molecular weight chitosan. The study examines properties like swelling, structure, morphology, thermal characteristics, and degradation in various mediums. The results highlight the impact of molecular weight on hydrogel properties and biodegradability.
Full Transcript
Received: 26 October 2023 Revised: 30 November 2023 Accepted: 28 December 2023 DOI: 10.1002/pen.26619 RESEARCH ARTICLE Comparative study of the effect of cross-linking degree on chitosan hydrogels synthesized with low and medium molecular weight chitosan lu 1 Hanife S...
Received: 26 October 2023 Revised: 30 November 2023 Accepted: 28 December 2023 DOI: 10.1002/pen.26619 RESEARCH ARTICLE Comparative study of the effect of cross-linking degree on chitosan hydrogels synthesized with low and medium molecular weight chitosan lu 1 Hanife Songül Kaçog | Özgür Ceylan 2 | Mithat Çelebi 3 1 Polymer Materials Engineering, Institute of Graduate Studies, Yalova University, Abstract Yalova, Turkey Chitosan (CS) is often used in hydrogels due to its natural origin, sustainability, 2 Central Research Laboratory Research non-toxicity, and biodegradability. However, the physicochemical properties of and Application Centre, Yalova CS hydrogels generated via physical cross-linking are relatively poor. CS hydro- University, Yalova, Turkey 3 Department of Polymer Materials gels were prepared by cross-linking with glutaraldehyde (GA) at different ratios Engineering, Faculty of Engineering, ranging from 1% to 10% (w/w), using two different molecular weight CS. The Yalova University, Yalova, Turkey swelling degree of hydrogels was investigated at pH 2.0, 5.6, and 7.4. The struc- Correspondence ture, morphology, and thermal characteristics of hydrogels were examined using Mithat Çelebi, Department of Polymer Fourier transform infrared, scanning electron microscopy, differential scanning Materials Engineering, Faculty of calorimetry, and thermogravimetric analysis, respectively. Compression tests Engineering, Yalova University, Yalova, Turkey. were conducted using Zwick/Roell universal testing equipment following ASTM Email: [email protected] D695. In vitro degradation studies were performed in enzyme and enzyme-free mediums at pH 7.4. The study demonstrated that low molecular weight CS Funding information Yalova University, Grant/Award Number: (L-CS) hydrogels have a lower sol content and higher swelling values than 2020/YL/0025 medium molecular weight CS (M-CS) hydrogels. Both L-CS and M-CS hydrogels cross-linked with 1% GA showed maximum swelling degrees at pH 2.0. Thermal analysis concluded that M-CS hydrogels have higher thermal stability than L-CS hydrogels. It was also found that the M-CS hydrogels have higher compressive moduli. The degradation experiments in both enzyme and enzyme-free media showed that L-CS hydrogels have higher degradation rates. Highlights Chemically cross-linked hydrogels synthesized using low and medium molecular weight chitosan. The mechanical and physical properties of medium and low molecular weight chitosan hydrogels were compared. Degradation experiments were performed in both enzyme and enzyme-free media. Swelling values decreased noticeably with the increasing molecular weight. Low molecular weight chitosan improved the biodegradability of hydrogels. This is an open access article under the terms of the Creative Commons Attribution-NonCommercial-NoDerivs License, which permits use and distribution in any medium, provided the original work is properly cited, the use is non-commercial and no modifications or adaptations are made. © 2024 The Authors. Polymer Engineering & Science published by Wiley Periodicals LLC on behalf of Society of Plastics Engineers. 1326 wileyonlinelibrary.com/journal/pen Polym Eng Sci. 2024;64:1326–1339. 15482634, 2024, 3, Downloaded from https://4spepublications.onlinelibrary.wiley.com/doi/10.1002/pen.26619 by Brock University, Wiley Online Library on [22/10/2024]. See the Terms and Conditions (https://onlinelibrary.wiley.com/terms-and-conditions) on Wiley Online Library for rules of use; OA articles are governed by the applicable Creative Commons License KAÇOGLU ET AL. 1327 KEYWORDS chitosan, compressive strength, degradation, molecular weight, sol content, swelling 1 | INTRODUCTION release, and tissue engineering systems. Modifying CS improves the intrinsic properties of polymers such as bio- Hydrogels are a type of polymeric material with a compatibility, chemical versatility, biodegradability, and hydrophilic character that allows them to retain enormous low toxicity. These adjustments can be made to fit a spe- volumes of water within their matrix.1 Chitosan (CS) is a cific application. CS's physical and chemical properties versatile semi-synthetic polymer that is employed in a vari- can be effectively and practically enhanced for use in ety of applications such as hydrogel and tissue engineering practical applications by cross-linking with cross-linking because of its unique features including biocompatibility, agents such as sodium tripolyphosphate, GA, N,N0 - biological degradation, and antibacterial activity.2–4 Cross- methylenebisacrylamide (MBA).3,20,21 linking mechanism of hydrogels occurs using chemical or Chemical cross-linked hydrogels that are stable have physical cross-linkers. So, the cross-linking reaction of CS superior mechanical properties and are resistant to disso- can be classified as chemical or physical based on how the lution even at high pH levels. These properties make CS cross-linking agents react with the CS.5 Glutaraldehyde applications more effective and popular in terms of physi- (GA) and genipin are the most frequently employed cross- cochemical stability.22,23 The effect of covalent cross- linking agents in the synthesizing of chemically cross-linked linking on CS modification has been the subject of CS-based hydrogels.6 numerous studies. The type and density of the cross- Hydrogels are three-dimensional cross-linked materials linker utilized have been proven to have a direct impact made of renewable (like, polysaccharides,7 carrageenan,8,9 on the properties of hydrogels, including mechanical CS5,6) or synthetic (like, polyacrylic acid,10,11 polyacryl- strength, mucoadhesiveness, porosity, swelling, and drug amide12,13) polymers. The extraordinary swelling character- release.24 J oźwiak et al. compared the properties of some istics make them useful in many fields.6 The widespread use chemical and physical cross-linkers to investigate the of hydrogel-based products in a variety of medical, indus- effect of the type of cross-linking agents on the swelling trial, and environmental applications is significant. Natural kinetics of Reactive Black 5 and the absorptive capacity hydrogels are progressively supplanting synthetic ones due of CS sorbents.25 Marija et al. investigated the photode- to their superior water absorption capacity, extended life gradation of textile dye C.I. Acid Orange 7 in an aqueous span, and diverse range of raw chemical resources.1 solution using synthesized colloidal and commercial tita- CS is synthesized by deacetylating chitin at alkali con- nium dioxide nanoparticles placed onto or into a hydro- ditions and contains an amine group.14,15 Chitin is exten- gel.26 Cui et al. produced a thermosensitive hydrogel sively found in crustaceans, insects, fungal organisms, using alginate-coated CS nanoparticles and hydroxypro- and yeast. CS offers significant benefits compared to con- pyl methylcellulose gel.27 Feyisa et al. developed a pH- ventional polymers derived from petroleum, including responsive dual-network hydrogel composed of sodium versatility, environmental friendliness, and non-toxic- alginate and CS, cross-linked using calcium chloride and ity.16 CS is defined as a deacetylation degree (DD) greater GA. The hydrogel was designed to release amoxicillin than 50%, while chitin is defined as DD less than 50%.17 specifically for the treatment of bacterial infections in the CS is biodegradable and can be metabolized in vivo by gastrointestinal system.15 Rodríguez-Loredo et al. pro- the lysozyme enzyme. As a result, CS has found applica- duced polyacrylic acid hydrogels using linseed mucilage, tions in pharmaceuticals and health care products such CS, and MBA as a chemical cross-linking agent. The as the water-based gel system.18 It has been discovered hydrogel composite was investigated for ketorolac that the use of CS/GA hydrogel systems as an adsorbent, adsorption and controlled release at varied pHs.21 particularly for wastewater treatment, holds great prom- Ovando-Medina et al. used acrylamide, CS, polypyrrole, ise.19 CS has been used in a variety of fields for many and MBA to obtain an electroactive hydrogel composed years, including agriculture, health, sensors, wastewater of polyacrylamide/CS/polypyrrole. This electroactive treatment, metal removal, and cosmetics. Furthermore, hydrogel was then used to deliver drugs controlled by CS has specific limitations when used as a scaffold, for electrical studies.20 controlled fertilizer release, or drug release. Chemical Dialdehydes are the most used chemical cross-linking modification can overcome these constraints. Cross- agents to synthesize CS-based hydrogels.6 Dialdehydes linked CS hydrogels have thus become increasingly allow spontaneous reactions under mild conditions. Also, important in recent research on drug release, fertilizer they make these reactions possible without any auxiliary 15482634, 2024, 3, Downloaded from https://4spepublications.onlinelibrary.wiley.com/doi/10.1002/pen.26619 by Brock University, Wiley Online Library on [22/10/2024]. See the Terms and Conditions (https://onlinelibrary.wiley.com/terms-and-conditions) on Wiley Online Library for rules of use; OA articles are governed by the applicable Creative Commons License 1328 KAÇOGLU ET AL. agents, which is beneficial for biocompatibility.28 GA is a CS cross-linking with GA. The formation of yellow color chemical cross-linking agent in hydrogel preparation due changed to orange as the GA concentration was increased. to its cross-linking capability and the benefits of being This is evidence of the CS and GA reaction.30 cheap and readily available. Because of its rapid reaction Also, it has been observed that increasing the molecu- and high cross-linking power, gelling occurs in less than lar weight of CS and the cross-linking concentration 1 min, depending on concentration. This is beneficial in increases color darkening as well as the cross-linking rate terms of biocompatibility.29 in the gels (Figure 2). As mentioned above, CS is one of the most used hydrogels due to its numerous advantages. However, the cross-linking degree and CS's molecular weight have a 2.3 | Sol determination critical effect on the CS gels' physical properties. It is the- oretically possible to predict that increasing the molecu- Sol amounts were determined by a previously reported lar weight of CS will negatively affect the swelling degree method.31 First, the gels were freeze-dried. The dry of the gels and positively affect their mechanical proper- weights, m0 , of hydrogel samples, were recorded. Then ties. However, the effects of both molecular weight and they were left in distilled water to swell at 37 C. The degree of cross-linking on the properties of hydrogels water medium was refreshed five times at 45-min inter- have not been investigated in detail and comparatively vals during swelling. Then gel samples were lyophilized before. We demonstrated that L-CS gels have higher again and the final dry weights (m1 ) were recorded. The swelling degrees, but lower compression moduli com- amount of CS that did not participate in the cross-linking pared to the medium molecular weight CS (M-CS) gels. reaction was determined using Equation (1). Both L-CS and M-CS gels showed higher resistance to degradation as the GA ratio increased. We also exhibited m 0 m1 Sol ¼ ð1Þ that M-CS gels are more resistant to enzymatic and non- m0 enzymatic degradation. where m0 : dry weights of hydrogels before swelling and m1 : dry weight of hydrogels after swelling and drying 2 | MATERIALS AND METHODS cycles. 2.1 | Materials 2.4 | Characterization Low (20–300 cP, 1 wt% in 1% acetic acid [25 C, Brookfield]) and medium-molecular (200–800 cP, 1 wt% in 1% acetic acid Fourier transform infrared (FTIR) spectra of hydrogels were [25 C, Brookfield]) weight CSs were obtained from Sigma– performed with Perkin-Elmer Spectrum 100 FTIR instru- Aldrich with ≥75 DD. Acetic acid, hydrochloric acid, sodium ment with ATR apparatus in the range of 4000–650 cm1, hydroxide, GA (25% w/w aqueous solution), potassium dihy- at a resolution of 4 cm1. drogen phosphate, disodium hydrogen phosphate, and lyso- The samples were cross-sectioned and coated with a zyme (70,000 U/mg) were obtained from Sigma–Aldrich. thin layer of gold to obtain topographic images on the The other chemicals are of analytical grade. ZEISS LEO-1430 VP model scanning electron microscopy (SEM) device at certain scales. The TA Instrument model DSC equipment was used 2.2 | Hydrogel preparation to conduct differential scanning calorimetry (DSC) ana- lyses. The temperature range was 10–400 C and the heat- The CS 2% (w/v) was dissolved in 2% (w/v) acetic acid ing rate of 10 C/min applied to determine the phase solution in a small beaker by mixing it in a magnetic stir- transition temperatures (Tg) of hydrogels. rer. Then the mixture was held in the ultrasonic bath for In a nitrogen gas atmosphere, thermogravimetric anal- 30 min to remove the air bubbles. A 1% (w/w) aqueous ysis (TGA) was carried out using the SDT 2960 Simulta- GA solution (w/w) was prepared by diluting from 25% neous DSC-TGA thermal analyzer. To examine hydrogel stock solution. Gels having different cross-linker ratios samples' thermal behavior the heating rate was 10 C/min were prepared by adding GA solution to CS solution at and the temperature range was between 20 and 600 C. the ratio of 1%, 3%, 5%, 7%, and 10% by weight respec- Compression tests were conducted with a Zwick/ tively. The mixtures were mixed rapidly to ensure a homo- Roell universal testing machine equipped with a 50 N geneous distribution and kept at room temperature for load cell according to the ASTM D695 standard. The sam- 24 h. Figure 1 shows the formation of imine bonds during ples which had 20 20 mm dimensions were prepared. 15482634, 2024, 3, Downloaded from https://4spepublications.onlinelibrary.wiley.com/doi/10.1002/pen.26619 by Brock University, Wiley Online Library on [22/10/2024]. See the Terms and Conditions (https://onlinelibrary.wiley.com/terms-and-conditions) on Wiley Online Library for rules of use; OA articles are governed by the applicable Creative Commons License KAÇOGLU ET AL. 1329 F I G U R E 1 Schematic representation of chitosan hydrogels prepared with glutaraldehyde. F I G U R E 2 Effect of increased glutaraldehyde (GA) concentration on the color of hydrogels with (A) (low molecular weight chitosan [L-CS]), (B) (medium molecular weight chitosan [M-CS]). Compression tests were carried out with three repeti- the gels and then they were put into the swelling tions. The test speed was selected as 10 mm/min. media again. This procedure was repeated until a con- stant weight was obtained, indicating that the swelling equilibrium had been reached. Swelling degrees were 2.5 | Swelling degree calculated using Equation (2).3 All swelling experi- ments were run on three samples for each GA The swelling degrees of the hydrogels were examined concentration. in three different media for simulating stomach, skin, and physiological pHs at pH 2.0, pH 5.6, and pH 7.4, Wd W0 S ð %Þ ¼ 100 ð2Þ respectively. First, the hydrogels were immersed in the W0 swelling media, then they were removed from these media at certain time intervals. The excess water was where S: swelling percentages (%), W d : swollen hydrogel carefully removed with filter paper before weighing weight, W 0 : the dry weight of the hydrogel. 15482634, 2024, 3, Downloaded from https://4spepublications.onlinelibrary.wiley.com/doi/10.1002/pen.26619 by Brock University, Wiley Online Library on [22/10/2024]. See the Terms and Conditions (https://onlinelibrary.wiley.com/terms-and-conditions) on Wiley Online Library for rules of use; OA articles are governed by the applicable Creative Commons License 1330 KAÇOGLU ET AL. 2.6 | In vitro degradation TABLE 1 % (w/w) sol contents of L-CS and M-CS hydrogels. L-CS Sol amounts M-CS Sol amounts L-CS and M-CS hydrogels with the lowest and highest 1% GA 31.0 ± 1.2 1% GA 45.5 ± 2.1 GA concentrations (1% and 10%) were used to determine the effectiveness of the increase in the cross-linker rate 3% GA 22.9 ± 1.5 3% GA 35.1 ± 2.0 on degradation. The hydrogels were lyophilized, cut into 5% GA 28.3 ± 1.9 5% GA 38.4 ± 2.2 equal sizes, and weighed. The samples were immersed 7% GA 20.1 ± 1.1 7% GA 52.8 ± 3.5 into 20 mL glass tubes containing lysozyme enzyme 10% GA 28.3 ± 1.3 10% GA 48.6 ± 2.9 (0.5 mg/mL) and non-enzymatically medium at phos- Abbreviation: GA, glutaraldehyde. phate buffer solution (pH 7.4, 100 mM) and incubated at 37 C in an ES-20 orbital shaker incubator. The presence of lysozyme enzyme in the phosphate buffer suggests an tissue scaffolds by facilitating cell metabolite input and enzymatic media, whereas the absence of lysozyme waste disposal through their pores.36 enzyme indicates a non-enzymatic environment. The hydrogels were transferred from the enzymatic and non- enzymatic solution after 4, 7, 15, 26, 50, and 84 days, 3.2 | Swelling washed with distilled water, dried on towel paper to elim- inate any excess water, and weighed. After each weigh- The CS hydrogels cross-linked with GA at 1%, 3%, 5%, ing, the samples were incubated again in the fresh 7%, and 10% by weight based on CS, reached maximum solution medium. The degradation (%) was calculated by swelling values at the end of 6 h as shown in Figure 3. Equation (3).32,33 In both L-CS and M-CS hydrogels, hydrogels with a GA concentration higher than 1% showed lower swelling W0 Wf values compared to hydrogels containing 1% GA. Silva D ð %Þ ¼ x100 ð3Þ W0 et al. mentioned that CS membranes with a low cross- linker ratio (1% GA) achieved a higher swelling com- where D (%): degradation percentage; W 0 : initial weight; pared to non-cross-linked CS, arguing that low crystal- W f : final weight. linity increases the accessibility of water molecules.37 On the other hand, it was seen that the swelling values decrease noticeably with the increasing molecular 3 | R ES U L T S A N D D I S C U S S I O N weight. The efficient and homogeneous mixture also increases the cross-linking efficiency. Therefore, the 3.1 | Sol content sol content of L-CS hydrogels is lower, and their swell- ing values are higher than M-CS hydrogels. Also, Ber- A sol fraction is the hydrogel's soluble portion, whereas ger et al. explained that increased molecular weight led a gel fraction is the hydrogel's insoluble component.34 to an improvement in the mechanical strength of CS The sol contents of the hydrogels are shown in Table 1. hydrogels and a decrease in swelling values.38 In this study, the average sol contents of L-CS and M-CS The swelling degrees of low and medium molecular hydrogels were calculated as 0.26 g/g and 0.44 g/g, weight CS hydrogels at pH 2.0 are shown in Figure 3A,B. respectively. When compared to M-CSs, L-CS hydrogels Low molecular weight CS hydrogels cross-linked with 1% showed more effective cross-linking because of lower GA displayed the highest swelling values. Also, it was molecular weight and relatively more comfortable observed that the increase in cross-linker concentration molecular mobility. Thus, this relatively prevents the significantly reduced the water absorption percentages of interactions between CHO and NH2 groups in the the gels. It is seen that the highest swelling values were matrix with the effect of high viscosity, low solubility, reached at pH 2.0 compared to pH 5.6 and pH 7.4. In the and ambient pH. The hydrogels we synthesized are acidic pH, the NH2 groups in the structure of CS are referred to be soft hydrogels due to their great water protonated and converted to the positively charged retention capacity and low gel content. Soft hydrogels NH3+ state. The presence of these ionic groups, are advantageous for soft tissue engineering applica- which increase flexibility and hydrophilicity along the tions, such as nerve regeneration guides.35 Their capac- CS chain, leads to the formation of repulsion between ity to hold high water content, preserve the porous them, and the expansion of the network structure. structure, and adapt to interchangeable sol-gel condi- Finally, solvent molecules penetrate easily into this tions have made them popular in recent years. Hydro- expanding structure. In this way, hydrogels that gels' structural properties enable them to function as absorb more solvent reach a higher swelling value. 15482634, 2024, 3, Downloaded from https://4spepublications.onlinelibrary.wiley.com/doi/10.1002/pen.26619 by Brock University, Wiley Online Library on [22/10/2024]. See the Terms and Conditions (https://onlinelibrary.wiley.com/terms-and-conditions) on Wiley Online Library for rules of use; OA articles are governed by the applicable Creative Commons License KAÇOGLU ET AL. 1331 F I G U R E 3 Time-dependent swelling percentage values of hydrogels at pH 2 for low molecular weight chitosan (L-CS) (A), pH 2 for medium molecular weight chitosan (M-CS) (B), pH 5.6 for L-CS (C), pH 5.6 for M-CS (D), pH 7.4 for L-CS (E), and pH 7.4 for M-CS (F). GA, glutaraldehyde. Protonation in the neutral or basic conditions is very increase in GA concentration, the number of amine limited and swelling degrees are much lower than groups in CS also decreases due to their participation that of pH 2.0 increased GA ratio. 39 With the in cross-linking. 15482634, 2024, 3, Downloaded from https://4spepublications.onlinelibrary.wiley.com/doi/10.1002/pen.26619 by Brock University, Wiley Online Library on [22/10/2024]. See the Terms and Conditions (https://onlinelibrary.wiley.com/terms-and-conditions) on Wiley Online Library for rules of use; OA articles are governed by the applicable Creative Commons License 1332 KAÇOGLU ET AL. A review of the data in Figure 4A shows a broad band of 3364–3225 cm1 characteristic band of N H and O H structures.41 An examination of the data in Figure 4A depicts a wide band at 2882 cm1 to the C H asymmetric stretching bond, 1677–1566 cm1 to the Amid-II groups of the sharp peaks detected in the range of N H bending vibration in the free amine ( NH2) groups,42 to the C H bending vibration of the peaks in the range 1373–1308 cm1 and the symmetrical deformation of CH3,43 to the asymmet- ric stretching of the C O C bonds of the small peak at 1155 cm1, to the asymmetric stretching of the C O C bonds at 1050–1026 cm1 its range is assigned to the C O stress, which is a characteristic of the polysaccharide struc- ture of the CS.44 When the FTIR spectra of hydrogels cross- linked with 1%, 5%, and 10% (w/w) GA are examined, small peak points are seen in the 1636 cm1 band. These bands are assigned to the being of C N of the imine bonds between CS and GA.45 The presence of this band confirms the Schiff reaction, that is, covalent cross-linking, of CS and GA. The intensity of the N H bending vibration band of the primary amines at 1647–1550 cm1 decreases and shifts to a lower wave number. This is attributed to the reduction of the severity of the N H band of the decreasing NH2 groups because of the reaction of CS with GA. The bands at 1058–1026 cm1 are stronger as the GA ratio rises, and the NH2 sway vibration band is seen at 619 cm1.46 The cross-linking reaction between CS and GA is demonstrated by all FTIR analysis results. When the FTIR spectra of M-CS pure CS and cross- linked CS hydrogels presented in Figure 4B are exam- F I G U R E 4 Fourier transform infrared spectra of low L-CS (A), ined, it can be seen that there is no noticeable shift in and M-CS (B) hydrogels. the spectra of gel samples with different Mw. Unlike L-CS, the C O stress peaks at 1050 cm1 and 913 cm1, attributed to the polysaccharide structure of the CS, lost The swelling values of L-CS hydrogels with a 10% GA their clarity and merged with the 1026 cm1 band. ratio were slightly higher than the swelling values of These results appear to coincide with L-CS spectra. L-CS hydrogels with a 7% GA ratio. This can be explained by the fact that 10% GA had the highest sol content. Increasing the sol content will reduce the cross-link yield 3.4 | SEM imaging and allow the gel to absorb more solvent. Similarly, 7% GA, which has the highest sol content among M-CS SEM analysis was accomplished to observe the cross- hydrogels, has shown more swelling value than gels with section morphologies of CS hydrogels cross-linked with 5% GA content (Figure 3). Chemical cross-linking 1% and 10% (w/w) GA, which have the highest and low- increased the degree of crystallinity, and hydrophobic- est swelling values, respectively. SEM images of L-CS and ity, and decreased swelling in CS hydrogels.40 M-CS hydrogels with different GA content are shown in Figure 5. It is seen that the hydrogel matrix has a porous structure, and with the increase in the amount of GA, a 3.3 | Fourier transform infrared analysis significant reduction in the pore sizes occurs. Compared to M-CS, L-CS hydrogels appear to have a relatively FTIR studies were conducted with non-cross-linked CS smoother, more homogeneous pore structure. It is and CS hydrogels cross-linked with GA at specified thought that this situation, low Mw, and low viscosity ratios. The FTIR spectra of L-CS and M-CS hydrogels are values allow more effective cross-linking and accordingly shown in Figure 4. the formation of a more homogeneous pore structure. It 15482634, 2024, 3, Downloaded from https://4spepublications.onlinelibrary.wiley.com/doi/10.1002/pen.26619 by Brock University, Wiley Online Library on [22/10/2024]. See the Terms and Conditions (https://onlinelibrary.wiley.com/terms-and-conditions) on Wiley Online Library for rules of use; OA articles are governed by the applicable Creative Commons License KAÇOGLU ET AL. 1333 F I G U R E 5 Scanning electron microscopy images of chitosan hydrogels cross-linked by (A) 1% glutaraldehyde (GA) for L-CS, (B) 10% GA for L-CS, (C) 1% GA for M-CS, (D) 10% GA for M-CS. was stated that the pore sizes of gels with low cross-link ratio were significantly larger and denser, and their sur- face appearance was smoother.47,48 reported that gels cross-linked with GA at different rates decreased pore sizes from 500 to 100 μm and swelling values decreased from 1200% to 600% with an increase in the amount of cross-linkers. 3.5 | Thermal analysis The glass transition (Tg) and decomposition temperatures of CS hydrogels cross-linked with 1%, 5%, and 10% (w/w) GA were determined by DSC analysis, and the results of the analysis are presented in Figure 6. The DSC thermo- gram shows small endothermic peaks between 68 and 82 C. Due to the crystalline structure of CS and the F I G U R E 6 Differential Scanning Calorimetry curves (Run 1) strong intramolecular and intermolecular hydrogen of L-CS and M-CS hydrogels with different glutaraldehyde (GA) ratios. bonds it contains, it is very difficult to determine the Tg value. Its hygroscopic structure, on the other hand, directly influences DSC analyses in particular.49,50 Dong cross-linked gels, with these values being 218 and 301 C, et al. (2004)49 determined the Tg value of CS at 140– respectively.51 DSC thermograms in Figure 6 show that 150 C utilizing four distinct strategies. However, Dragan with an increase in the GA ratio, the degradation temper- et al. determined this value to be 207 C using the DSC atures of the gels decrease slightly for both L-CS and method.50 The Tg value of CS hydrogels was not observed M-CS gels. TGA results also support these results. TG in this study. This may be due to the strong hydrogen and DTG results of hydrogels with 1%, 5%, and 10% bonds in the hydrogel matrix that make the gel compact. (w/w) GA are given in Figure 7. The first peaks observed Ostrowska-Czubenko et al. reported that the thermal sta- indicate the removal of the water bound to CS and the bility of cross-linked CS gels was lower than that of non- functional groups of GA, and the peaks observed in 15482634, 2024, 3, Downloaded from https://4spepublications.onlinelibrary.wiley.com/doi/10.1002/pen.26619 by Brock University, Wiley Online Library on [22/10/2024]. See the Terms and Conditions (https://onlinelibrary.wiley.com/terms-and-conditions) on Wiley Online Library for rules of use; OA articles are governed by the applicable Creative Commons License 1334 KAÇOGLU ET AL. Airoldi stated that a more disordered structure is formed because of the reaction of CS with GA and that this irregu- lar structure, which is formed by increasing GA concentra- tion, reduces the temperature of thermal decomposition by reducing crystallinity.30 Furthermore, hydrogels with M-CS were found to have slightly higher thermal strength com- pared to L-CS. Therefore, it is seen that the increased molec- ular weight has a favorable outcome on the thermal strength of CS hydrogels. According to the findings of Neto et al., the cross- linked sample is less stable than the un-crosslinked one since it starts to break down at lower temperatures.54 TGA results of hydrogels are depicted in Table 2. The decomposition temperature rose as the cross-linking agent concentration rose above a particular point, and the very low cross-linking degrees tended to reduce sam- ple stability. 3.6 | Compression test Compressive stress and strain values are shown in Figure 8. It is seen that the molecular weight of CS and the degree of cross-linking had a strong effect on the mechanical proper- ties of hydrogels. As the GA ratio increased, a significant decrease in the compressive strength of L-CS hydrogels was observed in Figure 8A. This decrease is more pronounced, especially after the GA ratio is higher than 3%. Most likely this is because of F I G U R E 7 Thermogravimetric analysis (TGA) (A) and the homogeneity problem. When preparing CS gels, there Derivative of thermogravimetric (DTG) (B) curves of L-CS and is very limited time to obtain a homogeneous mixture, M-CS hydrogels with different glutaraldehyde ratios (GA). since the viscosity increases rapidly (after about 18–20 s). When the molecular weight and GA cross-linker ratio are the range of 250–300 C indicate the decomposition of the low, it is easier to obtain a homogeneous mixture right CS main chain. before the viscosity build-up. It was concluded that According to the TG/DTG thermograms presented in homogeneity decreased after the GA ratio was higher Figure 7, CS hydrogels heated from 25 to 600 C appear to than 3% due to the dramatic diminish in maximum degrade in three stages. The first stage is the removal of strength values as depicted in Figure 8. These conclusions water and solvent in hydrogel samples at 50–85 C, which are in line with those of Arianita et al.55 Compressive cannot be completely removed by pre-drying; the second modulus values of the hydrogels are shown in Table 3. stage is at 140–180 C, where the depolymerization of the Increased GA ratio results in decreased elasticity of the CS backbone takes place; the third stage is the one attrib- gels and increased compression modulus. uted to the final decomposition at 260–300 C.24,52 The same behavior is seen in M-CS CS hydrogels. The decomposition temperatures of L-CS hydrogels were However, compared to L-CS hydrogels, M-CS hydrogels found to be 287.3, 286.5, and 268.7 C respectively, while have higher compression modulus. This can be explained M-CS hydrogels were 289.9, 285.3, and 281.1 C. The DTG as a result of M-CS hydrogels having a tougher structure results depict that the decomposition temperatures drop than L-CS. In addition, the increase in the GA ratio slightly with the increase in the cross-linkers ratio. As a mat- causes the cross-linking reaction to take place more ter of fact, by increasing the GA ratio from 1% to 10%, it is intensely and faster than L-CS gels. Therefore, viscosity seen that the decomposition temperature decreases from increases more rapidly (after 8–10 s), and obtaining a 287.3 to 268.5 C. These outcomes are in line with the find- homogeneous mixture is more difficult. Thus, it can be ings of Ostrowska-Czubenko et al.51 and Beppu et al.,53 who assumed that this may cause a problem of homogeneity studied the thermal behavior of CS-GA gels. Monteiro and that is not visible in hydrogels. 15482634, 2024, 3, Downloaded from https://4spepublications.onlinelibrary.wiley.com/doi/10.1002/pen.26619 by Brock University, Wiley Online Library on [22/10/2024]. See the Terms and Conditions (https://onlinelibrary.wiley.com/terms-and-conditions) on Wiley Online Library for rules of use; OA articles are governed by the applicable Creative Commons License KAÇOGLU ET AL. 1335 TABLE 2 Thermogravimetric analysis results of chitosan hydrogels. Tmax 1st decomposition 2nd decomposition temperature ( C) and temperature ( C) and decomposition decomposition Specimen T5 ( C) rate (%/min) rate (%/min) Residue (%) L-CS/1% GA 62.31 159.8/9.8 287.3/49.3 28.3 L-CS/5% GA 56.22 171.5/9.0 286.5/47.6 29.8 L-CS/10% GA 55.78 172.6/8.9 268.7/49.1 28.0 M-CS/1% GA 57.77 179.1/9.2 289.9/51.4 26.1 M-CS/5% GA 56.40 168.6/8.7 285.3/52.3 25.7 M-CS/10% GA 58.57 143.1/6.8 281.1/54.6 27.6 Abbreviations: L-CS, low molecular weight chitosan; M-CS, medium molecular weight chitosan; T5, The temperature at 5% mass loss; Tmax, The temperature for the onset of maximum degradation. FIGURE 8 Stress-strain curves of L-CS (A) and M-CS (B) hydrogels cross-linked by different glutaraldehyde ratios (GA). 3.7 | In vitro degradation T A B L E 3 Compressive moduli of low molecular weight chitosan (L-CS) and medium molecular weight chitosan (M-CS) Lysozyme enzyme causes degradation by hydrolyzing the hydrogels. β-(1,4) glycoside bonds between D-glucosamine and Glutaraldehyde concentration Module (kPa) N-acetylglucosamine groups of CS in vivo.56 The lyso- zyme was chosen to mimic the biological environment. % (w/w) mM L-CS M-CS The rate of degradation of biomaterials is critical in 1 2 2.2 2.1 deciding their application. For example, the material uti- 3 6 4.2 4.8 lized in tissue engineering must have a low degree of deg- 5 10 7.1 11.0 radation to have an extended period of action. The 7 14 10.1 12.8 degradation rates of low and medium molecular weight 10 20 15.1 15.6 CS hydrogels crosslinked with 1% and 10% GA incubated in enzymatic and non-enzymatic environments are shown in Figure 9A,B. Hydrogels exposed to non-enzymatic degradation pre- degradation rates have weight losses that reach approxi- served their structural integrity compared to hydrogels mately 75% degradation values at the end of the 84-day subjected to enzymatic degradation. The increase in GA incubation period. The degradation rate of CS hydrogels concentration appears to have a direct effect on the is slightly higher in gels containing 1% GA. Gels contain- weight loss of CS hydrogels. Gels that exhibit low ing 10% GA were found to be the most resistant to 15482634, 2024, 3, Downloaded from https://4spepublications.onlinelibrary.wiley.com/doi/10.1002/pen.26619 by Brock University, Wiley Online Library on [22/10/2024]. See the Terms and Conditions (https://onlinelibrary.wiley.com/terms-and-conditions) on Wiley Online Library for rules of use; OA articles are governed by the applicable Creative Commons License 1336 KAÇOGLU ET AL. FIGURE 9 Enzymatic and non-enzymatic degradation of L-CS (A) and M-CS (B) hydrogels. degradation. This shows that the cross-linker ratio in the 4 | CONCLUSION hydrogel has a significant effect on enzymatic and non- enzymatic degradation, and it appears that the interac- The effects of different GA concentrations (1%, 3%, 5%, tion between the lysozyme enzyme and the polymer 7%, and 10% w/w) and CS with two different molecular chains can be prevented by high cross-link density. In weights on the cross-linking degree, swelling, mechani- addition, it can be explained by the strong intramolecular cal, thermal, morphological, and degradation properties interactions of the CS biopolymer that slow down the of hydrogels were thoroughly investigated. L-CS hydro- degradation process. When L-CS and M-CS hydrogels are gels were found to have smoother and more homoge- compared, it is thought that the increased molecular nous pore structures than M-CS hydrogels. M-CS weight reduces biodegradability, which is due to the CS hydrogels have lower swelling values than expected structure that becomes more stable with the increased because of their higher molecular weight. In general, molecular weight. Lončarevic et al. explained that high both L-CS and M-CS hydrogels' water absorption per- crystallinity and high molecular weight reduce the rate of centages were greatly reduced by increased GA (cross- degradation, as well as CS decomposition is affected by linker) concentration. The lowest swelling percentages other parameters such as ambient pH, cross-linking were observed at pH 7.4 compared to pH 2.0 and pH 5.6. agent, and enzyme concentration.57 Cui et al. prepared The study showed that the molecular weight and cross- CS/sodium alginate nanoparticle-based thermosensitive linker ratio have a great effect on the mechanical hydrogels. Hydroxypropyl methylcellulose (HPMC) mix- strength of hydrogels. The cross-linker ratio of more ture solution was mixed with bovine serum albumin- than 3% dramatically led the hydrogels to lose flexibility, loaded nanoparticles to make hydrogel composites. They become tougher, and their maximum strength decreased. concluded a significant weight loss difference between Increasing the GA ratio resulted in decreased elasticity HPMC hydrogel and hydrogel-lysozyme suggests faster and and thus increased compressive modulus. This is also an higher degradation in lysozyme enzyme media compared to expected phenomenon for hydrogels. M-CS hydrogels have spontaneous degradation. The HPMC hydrogel in lyso- a higher compression modulus than L-CS hydrogels. It zyme enzyme media reached near-complete dissolution was found that the decomposition temperatures of M-CS at 7 days, with a degradation percentage of 82.66%.27 In hydrogels were slightly higher than L-CS hydrogels. The our study, the enzymatic and non-enzymatic degrada- degradation of L-CS hydrogel cross-linked with 1% GA tion rate of the M-CS hydrogels was very slow with was found to be higher than MCS hydrogel for lysozyme below 25% at 7 days. enzyme and enzyme-free medium respectively. Degrada- These developed CS hydrogels can be used in tissue tion experiments clearly showed that the degradation rate engineering because of their highwater retention capac- decreases as the molecular weight increases. ity, and low gel contents, the controlled release of antitu- mor and antibiotic-type drugs, fertilizers, pesticides, and AUTHOR CONTRIBUTIONS similar chemicals, and agricultural productivity due to Hanife Songül Kaço glu: Investigations; experiments. their slow and controlled degradation properties. Özgür Ceylan: Methodology; reviewing; Writing original 15482634, 2024, 3, Downloaded from https://4spepublications.onlinelibrary.wiley.com/doi/10.1002/pen.26619 by Brock University, Wiley Online Library on [22/10/2024]. See the Terms and Conditions (https://onlinelibrary.wiley.com/terms-and-conditions) on Wiley Online Library for rules of use; OA articles are governed by the applicable Creative Commons License KAÇOGLU ET AL. 1337 draft; Supervision; Editing. Mithat Çelebi: Reviewing; suspension polymerization. Polym Eng Sci. 2019;59(1):162-169. Writing original draft; Supervision; Project administration. doi:10.1002/pen.24883 11. Doan THY, Pham TD, Yamashita Y, Adachi Y. Adsorption of poly(acrylic acid) onto negatively charged polystyrene sulfate A C K N O WL E D G M E N T S latex particles by means of particle tracking of Brownian The authors would like to express their gratitude to motion, electrophoretic mobility and Fourier transform infra- Yalova University Scientific Research Projects Coordina- red spectroscopy. Polym Sci Ser A. 2020;62(4):321-329. doi:10. tors for providing financial support for this study (Project 1134/S0965545X20040045 No: 2020/YL/0025). 12. Nyyssölä A, Ahlgren J. Microbial degradation of polyacryl- amide and the deamination product polyacrylate. Int Biodeter CONFLICT OF INTEREST STATEMENT Biodegr. 2019;139:24-33. doi:10.1016/j.ibiod.2019.02.005 13. Yang C, Yin T, Suo Z. Polyacrylamide hydrogels. I. Network The authors declare no conflicts of interest. imperfection. J Mech Phys Solids. 2019;131:43-55. doi:10.1016/j. jmps.2019.06.018 DATA AVAILABILITY STATEMENT 14. Terzic I, Ivanovic J, Žižovic I, et al. A novel chitosan gels: The data presented in this study are obtained on request supercritical CO2 drying and impregnation with thymol. Polym from the corresponding authors. Eng Sci. 2018;58(12):2192-2199. doi:10.1002/pen.24834 15. Feyisa Z, Gupta NK, Edossa GD, Sundaramurthy A, Kapoor A, ORCID Inki LG. Fabrication of pH-sensitive double cross-linked sodium Mithat Çelebi https://orcid.org/0000-0002-2013-5354 alginate/chitosan hydrogels for controlled release of amoxicillin. Polym Eng Sci. 2023;63(8):2546-2564. doi:10.1002/pen.26395 16. Kulikouskaya V, Hileuskaya K, Kraskouski A, et al. Chitosan- R EF E RE N C E S capped silver nanoparticles: a comprehensive study of polymer 1. Ahmed EM. Hydrogel: preparation, characterization, and appli- molecular weight effect on the reaction kinetic, physicochemi- cations: a review. J Adv Res. 2015;6(2):105-121. doi:10.1016/j.jare. cal properties, and synergetic antibacterial potential. SPE 2013.07.006 Polym. 2022;3(2):77-90. doi:10.1002/pls2.10069 2. Lu Z, Zou L, Zhou X, Huang D, Zhang Y. High strength chito- 17. Gamiz-Gonzalez MA, Correia DM, Lanceros-Mendez S, san hydrogels prepared from NaOH/urea aqueous solutions: Sencadas V, G omez Ribelles JL, Vidaurre A. Kinetic study of the role of thermal gelling. Carbohydr Polym. 2022;297:120054. thermal degradation of chitosan as a function of deacetylation doi:10.1016/j.carbpol.2022.120054 degree. Carbohydr Polym. 2017;167:52-58. doi:10.1016/j.carbpol. 3. Akakuru O, Isiuku BO. Chitosan hydrogels and their 2017.03.020 glutaraldehyde-crosslinked counterparts as potential drug 18. Hee DH, Da EN, Dong HS, Tae WK, Byung CS, Ho SC. Prepa- release and tissue engineering systems – synthesis, characteri- ration and biodegradation of thermosensitive chitosan hydrogel zation, swelling kinetics and mechanism. J Phys Chem Biophys. as a function of pH and temperature. Macromol Res. 2004; 2017;7(3):1-7. doi:10.4172/2161-0398.1000256 12(5):507-511. doi:10.1007/bf03218435 _ Demirel E. Modification of PVDF membranes using 4. Üçel IS, 19. Ruiz M, Sastre AM, Guibal E. Palladium sorption on dopamine/zinc oxide for lead removal from aqueous media. glutaraldehyde-crosslinked chitosan. React Funct Polym. 2000; Open J Nano. 2022;7(2):53-73. doi:10.56171/ojn.1058222 45(3):155-173. doi:10.1002/app.1425 5. Hamedi H, Moradi S, Hudson SM, Tonelli AE. Chitosan based 20. Ovando-Medina VM, Reyes-Palacios GA, García-Montejano LA, hydrogels and their applications for drug delivery in wound Antonio-Carmona ID, Martínez-Gutiérrez H. Electroactive poly- dressings: a review. Carbohydr Polym. 2018;199:445-460. doi:10. acrylamide/chitosan/polypyrrole hydrogel for captopril release 1016/j.carbpol.2018.06.114 controlled by electricity. J Vinyl Addit Technol. 2021;27(4):679- 6. Wei B, Zou J, Pu Q, Shi K, Xu B, Ma Y. One-step preparation 690. doi:10.1002/vnl.21842 of hydrogel based on different molecular weights of chitosan 21. Rodríguez-Loredo NA, Ovando-Medina VM, Pérez E, et al. with citric acid. J Sci Food Agric. 2022;102(9):3826-3834. doi:10. Preparation of poly(acrylic acid)/linseed mucilage/chitosan 1002/jsfa.11732 hydrogel for ketorolac release. J Vinyl Addit Technol. 2023; 7. Shahabi-Ghahfarrokhi I, Almasi H, Babaei-Ghazvini A. Char- 29(5):890-900. doi:10.1002/vnl.22023 acteristics of biopolymers from natural resources. Processing 22. Fu J, Yang F, Guo Z. The chitosan hydrogels: from structure to and Development of Polysaccharide-Based Biopolymers for Pack- function. New J Chem. 2018;42(21):17162-17180. doi:10.1039/ aging Applications. Elsevier; 2020:49-95. doi:10.1016/B978-0-12- C8NJ03482F 818795-1.00003-4 23. Woźniak A, Biernat M. Methods for crosslinking and stabili- 8. Distantina S, Rochmadi FM, Wiratni W. Preparation and char- zation of chitosan structures for potential medical applica- acterization of glutaraldehyde-crosslinked kappa carrageenan tions. J Bioact Compat Polym. 2022;37(3):151-167. doi:10. hydrogel. Eng J. 2013;17(3):57-66. doi:10.4186/ej.2013.17.3.57 1177/08839115221085738 9. Aydınoglu D, Karaca N, Ceylan Ö. Natural carrageenan/psyllium 24. Szyma nska E, Winnicka K. Stability of chitosan – a challenge composite hydrogels embedded montmorillonite and investiga- for pharmaceutical and biomedical applications. Mar Drugs. tion of their use in agricultural water management. J Polym Envi- 2015;13(4):1819-1846. doi:10.3390/md13041819 ron. 2021;29(3):785-798. doi:10.1007/s10924-020-01914-5 25. Joźwiak T, Filipkowska U, Filipkowska M. Effect of ionic and 10. Ceylan Ö, Kaya MA, Sarac A. Preparation of partially neutral- covalent crosslinking of hydrogel chitosan beads on the adsorp- ized poly(acrylic acid) microspheres via inverse Pickering tion efficiency of basic violet 10 and basic green 4 dyes from 15482634, 2024, 3, Downloaded from https://4spepublications.onlinelibrary.wiley.com/doi/10.1002/pen.26619 by Brock University, Wiley Online Library on [22/10/2024]. See the Terms and Conditions (https://onlinelibrary.wiley.com/terms-and-conditions) on Wiley Online Library for rules of use; OA articles are governed by the applicable Creative Commons License 1338 KAÇOGLU ET AL. aqueous solutions. Prog Chem Appl Chitin Deriv. 2022;27:116- microspheres. Polym Adv Technol. 2008;19:432-441. doi:10. 134. doi:10.15259/pcacd.27.009 1002/pat 26. Marija L, Nedeljko M, Maja R, Zoran Š, Marija R, Melina KK. 41. Nangia S, Katyal DN, Warkar SG. Kinetics, absorption and dif- Photocatalytic degradation of C.I. Acid Orange 7 by TiO2 nano- fusion mechanism of crosslinked chitosan kinetics, absorption particles immobilized onto/into chitosan-based hydrogel. and diffusion mechanism of crosslinked chitosan hydrogels. Polym Compos. 2014;35(4):806-815. doi:10.1002/pc.22724 Indian J Eng Mater Sci. 2021;28:374-384. 27. Cui CL, Gan L, Lan XY, et al. Development of sustainable car- 42. Queiroz MF, Melo KRT, Sabry DA, Sassaki GL, Rocha HAO. rier in thermosensitive hydrogel based on chitosan/alginate Does the use of chitosan contribute to oxalate kidney stone for- nanoparticles for in situ delivery system. Polym Compos. 2019; mation? Mar Drugs. 2015;13(1):141-158. doi:10.3390/md13010141 40(6):2187-2196. doi:10.1002/pc.25024 43. Krishna Rao KSV, Vijaya Kumar Naidu B, Subha MCS, 28. Noble L, Gray AI, Sadiq L, Uchegbu IF. A non-covalently Sairam M, Aminabhavi TM. Novel chitosan-based pH-sensitive cross-linked chitosan based hydrogel. Int J Pharm. 1999;192(2): interpenetrating network microgels for the controlled release 173-182. doi:10.1016/S0378-5173(99)00306-3 of cefadroxil. Carbohydr Polym. 2006;66(3):333-344. doi:10. 29. Migneault I, Dartiguenave C, Bertrand MJ, Waldron KC. Glu- 1016/j.carbpol.2006.03.025 taraldehyde: behavior in aqueous solution, reaction with pro- 44. Gorgieva S, Kokol V. Preparation, characterization, and teins, and application to enzyme crosslinking. Biotechniques. in vitro enzymatic degradation of chitosan-gelatine hydrogel 2004;37(5):790-802. doi:10.2144/04375rv01 scaffolds as potential biomaterials. J Biomed Mater Res A. 2012; 30. Monteiro O, Airoldi C. Some studies of crosslinking chitosan– 100A(7):1655-1667. doi:10.1002/jbm.a.34106 glutaraldehyde interaction in a homogeneous system. Int J Biol 45. Martínez-Mejía G, Vazquez-Torres NA, Castell-Rodríguez A, Macromol. 1999;26:119-128. doi:10.4183/aeb.2010.521 del Río JM, Corea M, Jiménez-Juarez R. Synthesis of new 31. Zhou Y, Zhang C, Liang K, et al. Photopolymerized water-soluble chitosan-glutaraldehyde scaffolds for tissue engineering using maleilated chitosan/methacrylated poly (vinyl alcohol) hydrogels Schiff reactions. Colloids Surf A Physicochem Eng Asp. 2019; as potential tissue engineering scaffolds. Int J Biol Macromol. 579:123658. doi:10.1016/j.colsurfa.2019.123658 2018;106:227-233. doi:10.1016/j.ijbiomac.2017.08.002 46. Kamari A, Pulford ID, Hargreaves JSJ. Chitosan as a potential 32. Tenorio FS, do Amaral Montanheiro TL, dos Santos AMI, dos amendment to remediate metal contaminated soil – a charac- Santos Silva M, Lemes AP, Tada DB. Chitosan hydrogel cova- terization study. Colloids Surf B Biointerfaces. 2011;82(1):71-80. lently crosslinked by gold nanoparticle: eliminating the use of doi:10.1016/j.colsurfb.2010.08.019 toxic crosslinkers. J Appl Polym Sci. 2021;138(6):1-13. doi:10. 47. Ritonga H, Nurdin M, Rembon F, Ramadhan LOAN, 1002/app.49819 Fitriana F. Preparation of hydrogel chitosan co-polyacrilamide 33. Ren D, Yi H, Wang W, Ma X. The enzymatic degradation and crosslinked glutaraldehyde. Paper presented at: 1st Interna- swelling properties of chitosan matrices with different degrees tional Conference on Science and Technology, Makassar, of N-acetylation. Carbohydr Res. 2005;340(15):2403-2410. doi: Indonesia; 2019. doi:10.4108/eai.2-5-2019.2284680 10.1016/j.carres.2005.07.022 48. Mirzaei B., E., Ramazani A., Shafiee M., & Danaei M. Stud- 34. Suhail M, Shao YF, Vu QL, Wu PC. Designing of pH-sensitive ies on glutaraldehyde crosslinked chitosan hydrogel proper- hydrogels for colon targeted drug delivery; characterization and ties for drug delivery systems. International Int. J. Polym. in vitro evaluation. Gels. 2022;8(3):155. doi:10.3390/gels8030155 Mater. 2013;62(11):605–611. 35. Kłosinski KK, Wach RA, Girek-Bąk MK, Rokita B, Kolat D, 49. Dong Y, Ruan Y, Wang H, Zhao Y, Bi D. Studies on glass tran- Kaluzinska-Kolat Z, Klosinska B, Duda L, Pasieka ZW. Bio- sition temperature of chitosan with four techniques. J Appl compatibility and mechanical properties of carboxymethyl chit- Polym Sci. 2004;93(4):1553-1558. doi:10.1002/app.20630 osan hydrogels. Polymers (Basel). 2022;15(1):144-160. doi:10. 50. Dragan ES, Perju MM, Dinu MV. Preparation and characteriza- 3390/polym15010144 tion of IPN composite hydrogels based on polyacrylamide and 36. Mantha S, Pillai S, Khayambashi P, et al. Smart hydrogels in chitosan and their interaction with ionic dyes. Carbohydr tissue engineering and regenerative medicine. Materials. 2019; Polym. 2012;88(1):270-281. doi:10.1016/j.carbpol.2011.12.002 12(20):3323-3356. doi:10.3390/ma12203323 51. Ostrowska-Czubenko J, Gierszewska M, Pier og M. pH- 37. Silva, R., Silva, G., Coutinho, O., Mano, J., & Reis, R. L.. responsive hydrogel membranes based on modified chitosan: Preparation and characterisation in simulated body condi- water transport and kinetics of swelling. J Polym Res. 2015;22 tions of glutaraldehyde crosslinked chitosan membranes. J. (8):1-12. doi:10.1007/s10965-015-0786-3 Mater. Sci.: Mater, 2004;15(10):1105-1112. doi:10.1023/B: 52. Rahman L, Goswami J, Choudhury D. Assessment of physical JMSM.0000046392.44911.46 and thermal behaviour of chitosan-based biocomposites rein- 38. BergerJ,ReistM,MayerJM,FeltO,PeppasNA,GurnyR.Structure forced with leaf and stem extract of Tectona grandis. Polym and interactions in covalently and ionically crosslinked chitosan Polym Compos. 2022;30:1-12. doi:10.1177/09673911221076305 hydrogels for biomedical applications. Eur J Pharm Biopharm. 53. Beppu MM, Vieira RS, Aimoli CG, Santana CC. Crosslinking 2004;57(1):19-34.doi:10.1016/S0939-6411(03)00161-9 of chitosan membranes using glutaraldehyde: effect on ion per- 39. Budianto E, Muthoharoh SP, Nizardo NM. Effect of meability and water absorption. J Membr Sci. 2007;301(1–2): crosslinking agents, pH and temperature on swelling behav- 126-130. doi:10.1016/j.memsci.2007.06.015 ior of cross-linked chitosan hydrogel. Asian J Appl Sci. 2015; 54. Neto CGT, Giacometti JA, Job AE, Ferreira FC, Fonseca JLC, 3(5):2321-2893. Pereira MR. Thermal analysis of chitosan based networks. Car- 40. Gupta KC, Jabrail FH. Effect of molecular weight and degree of bohydr Polym. 2005;62(2):97-103. doi:10.1016/j.carbpol.2005. deacetylation on controlled release of isoniazid from chitosan 02.022 15482634, 2024, 3, Downloaded from https://4spepublications.onlinelibrary.wiley.com/doi/10.1002/pen.26619 by Brock University, Wiley Online Library on [22/10/2024]. See the Terms and Conditions (https://onlinelibrary.wiley.com/terms-and-conditions) on Wiley Online Library for rules of use; OA articles are governed by the applicable Creative Commons License KAÇOGLU ET AL. 1339 55. Arianita A, Cahyaningtyas, Amalia B, Pudjiastuti W, Mela- scaffolds. J Tissue Repair Regen. 2017;1(1):12-22. doi:10.14302/ nie S, Fauzia V, Imawan C. Effect of glutaraldehyde to the issn.2640-6403.jtrr-17-1840 mechanical properties of chitosan/nanocellulose. J Phys Conf Ser. 2019;1317(1):012045. doi:10.1088/1742-6596/ 1317/1/012045 How to cite this article: Kaçoglu HS, Ceylan Ö, 56. Collins MN, Birkinshaw C. Comparison of the effectiveness of Çelebi M. Comparative study of the effect of cross- four different crosslinking agents with hyaluronic acid hydro- gel films for tissue-culture applications. J Appl Polym Sci. 2007; linking degree on chitosan hydrogels synthesized 104(5):3183-3191. doi:10.1002/app.25993 with low and medium molecular weight chitosan. 57. Lončarevic A, Ivankovic M, Rogina A. Lysozyme-induced deg- Polym Eng Sci. 2024;64(3):1326‐1339. doi:10.1002/ radation of chitosan: the characterization of degraded chitosan pen.26619