Pressure Measuring Devices PDF
Document Details
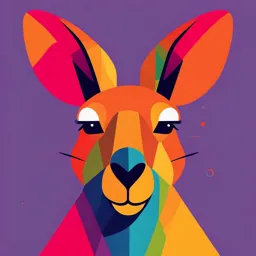
Uploaded by Reusl
Tags
Summary
This document is an educational resource explaining the various types of pressure measuring devices, including manometers (U-tube and inclined), and barometers. It describes how these devices measure pressure and their practical applications in energy plants.
Full Transcript
Unit A-9 • Energy Plant Instrumentation and Controls Objective 1 Describe the types of pressure sensing and measuring devices. Pressure Measuring Devices Pressure is one of the most important measured variables in power plant operation. It includes measurement of steam pressure in a boiler, fuel p...
Unit A-9 • Energy Plant Instrumentation and Controls Objective 1 Describe the types of pressure sensing and measuring devices. Pressure Measuring Devices Pressure is one of the most important measured variables in power plant operation. It includes measurement of steam pressure in a boiler, fuel pressure entering a burner, feedwater pressure, and many others. Pressure is also involved directly or indirectly in the measurement of other variables, such as level, flow, and density. Since these applied pressures (located in various parts of a plant) can range from a few to many thousand kilopascals, the same type of pressure sensing element cannot be used in all applications. Manometers A manometer is one of the most accurate and useful devices for measuring pressure in the lower ranges. There are three general types that are used in industry: the U-tube manometer, the inclined manometer, and the barometer. U-Tube Manometer The main purpose of a manometer is to measure the difference between two pressures (differential pressure measurement). Manometers can be used to measure low pressures, or even vacuums. A common plant application is the measurement of boiler furnace pressure or draft. A U-tube manometer can be a simple glass or other transparent material bent into the shape of a letter “U” as shown in Figure 1. Figure 1 – U-Tube Manometer P2 P1 4 4 3 3 2 2 1 1 0 0 1 1 2 2-4 Low Pressure Side P2 B 2 3 3 4 4 4th Class Edition 3 • Part A High Pressure Side P1 h A Introduction to Process Measurement • Chapter 2 The U-tube manometer contains a liquid, often referred to as the manometer fluid. This liquid must not mix or react chemically with the fluid whose pressure is being measured. Typical manometer fluids are water, mercury, and light oils. Mercury was a common manometer fluid in the past, but has been replaced due to its environmental and health hazards. With both sides of the manometer open to the atmosphere, the surface level on one side will be the same as the level on the other side, or P1 = P2 as in Figure 1(a). Suppose one end of the U-tube manometer is connected to an unknown pressure source, P1, while the other end is connected to another unknown pressure, P2. If P1 is greater than P2, the fluid on the right side of the manometer (Figure 1 (b)) will be displaced from its previous position, and forced into the left side of the manometer until a balance in pressure is created at points A and B, so the differential pressure can be read from the attached scale. Pressure at A = Pressure at B, or P1 = P2 + the pressure due to the height (or “head”) of liquid “h” P1 = P2 + hρg and P1 − P2 = hρg Where: h = height, meters ρ = density kg/m3 (Greek letter “rho”) ρ g = gravitational acceleration (9.81 m/s2) If P2 is the atmospheric pressure, then the pressure represented by the liquid head, hρg, would be equal to the amount that P1 exceeds atmospheric pressure. P2 + hρg would be equal to the absolute pressure applied on the right side of the manometer. If the pressure P1 is below atmospheric, and P2 is still the atmospheric pressure, the manometer fluid will be higher on the right side. Inclined Manometer Figure 2 is a special design of manometer. Its tube is inclined at an angle to the horizontal while the vertical part contains a well or fluid reservoir. This inclined manometer has a longer scale of measurement than the U-tube for the same differential in pressure, so a more accurate reading can be obtained. It is used where very small pressure differentials must be measured, such as boiler draft and furnace pressure. The inclined manometer is also used to calibrate other pressure measuring devices. The pressure due to the vertical height of a manometer, h, is equivalent to the difference in pressure between P1 and P2, where P2 often represents the atmospheric pressure. Figure 2 – Inclined Manometer P2 To Pressure Under Test P1 h Well Angle Column 4th Class Edition 3 • Part A 2-5 Unit A-9 • Energy Plant Instrumentation and Controls Barometers The earth’s atmosphere consists of a mixture of gases extending to an altitude of about 75 km. This atmospheric gas mixture has a mass that exerts a pressure of approximately 101.3 kPa on the earth’s surface, at sea level. Atmospheric pressure varies with the elevation above sea level, and daily, depending on weather conditions. One way to measure atmospheric pressure is with a mercury barometer. It consists of a glass tube of uniform bore, approximately 900 mm long, with one end sealed. Refer to Figure 3. The tube is first completely filled with mercury. It is then inverted with the open end submerged in an open dish of mercury. The pressure exerted by the column of mercury, h, is balanced by the pressure of the atmosphere. Figure 3 – Inverted Mercury Column Sealed Glass Tube Mercury h Well Figure 4 shows the components of an actual mercury barometer. As seen, the mercury barometer is essentially a manometer. Atmospheric pressure is exerted on the surface of the mercury in the well. The surface of the mercury in the sealed glass tube is exposed to a vacuum. In this manner, a barometer measures absolute atmospheric pressure. 2-6 4th Class Edition 3 • Part A Introduction to Process Measurement • Chapter 2 Figure 4 – Mercury Barometer Slide with Vernier Scale Top of Mercury Scale on Barometer Zero Point Mercury Barometer Bowl of Barometer Bourdon Tube Gauge The bourdon tube pressure gauge consists of an oval tube in the form of the letter “C,” with an arc of about 270°. The free end of the tube in Figure 5 is sealed. The other end is connected to the pressure source through a socket. When pressure is applied to the inside of the tube, the tube becomes slightly more circular in cross section, as shown by the dotted lines in Section A-A. As the tube becomes more circular in cross section, it straightens out slightly, causing the free end to move. This somewhat linear motion of the free end is transmitted through a link to a geared sector and pinion that causes rotation of the pointer. If the applied pressure decreases, the tube will act like a spring, and return to its original shape. Bourdon tube pressure gauges are available in many pressure ranges. Gauges are selected so that normal process pressures occur mid-scale on the gauge, where the gauge has its greatest accuracy. As well, when a typical process pressure is shown mid-range on a pressure gauge, its needle points directly upward. This gives a quick visual indication that the pressure is “normal.” 4th Class Edition 3 • Part A 2-7 Unit A-9 • Energy Plant Instrumentation and Controls Figure 5 – Bourdon Tube Gauge Cross Section of Pressurized Tube Normal Cross-section of Tube Scale (E) Section A-A 0 100 Pointer (D) A Tip (C) Free End A Link Bourdon Tube (B) Geared Sector (rack and pinion) Fixed End Socket (A) Pressure A bourdon tube can also be shaped in the form of a spiral or helix, as illustrated in Figure 6. These types are used to develop sufficient power and rotation to position a pen directly on a chart, without the use of gears. A greater degree of rotation is achieved as more windings are added. Figure 6 – Circular Chart Recorder with Spiral and Helical Bourdon Tubes Helix Bourdon Spiral Bourdon 12 Hour Chart Pens Coloured Indication The bourdon tube gauge is generally a stand-alone device. It is more versatile and rugged than the manometer, and is probably the most commonly used instrument in industry. 2-8 4th Class Edition 3 • Part A Introduction to Process Measurement • Chapter 2 Diaphragm Elements Bourdon tube gauges are not well suited to low pressure ranges because of the stiffness of their metal parts. A diaphragm element gauge, using more flexible material, can be used in lowpressure applications. Diaphragms are made of materials such as leather, cotton lined rubber, silk, copper, and stainless steel, depending upon the pressure applied and the temperature of the fluid. The diaphragm is a flat flexible disc. Some discs have concentric corrugations to increase motion and sensitivity. Non-metallic or “limp” diaphragms are used for pressures from 0 to 0.5 kPa. The metallic types are suitable for pressures of 0 to 2500 kPa. Figure 7 shows an example of a corrugated diaphragm. If the applied pressure is above atmospheric, the diaphragm is forced upwards, and its motion is transmitted to the pushrod that causes the pointer to rise on the scale. When the applied pressure is below atmospheric, the motion of the diaphragm is reversed, so the pointer moves downward. The motion of the pointer can be increased with a link lever mechanism. Figure 7 – Diaphragm Type Pressure Indicator Calibration Counter Weight Pivot Corrugated Diaphragm Pointer Front Mounted Scale Solid Disc Pressure or Vaccum Connection Zero Ajusting Screw Zero Adjusting Spring Bellows Element The bellows element is often used to measure pressure from approximately 250 mm to 35.7 m water head (2.5 to 350 kPa). It consists of a corrugated metal tube that expands in length when pressure is applied internally. Some designs of this device use special calibrating springs (Figure 8) to provide greater accuracy. The expansion and contraction of a bellows is often used to drive a transducer (i.e. to convert different types of signals). Bellows are commonly used in pneumatic devices to position nozzle and flapper clearances, and in electronic devices to position variable resistors and solenoid cores. Unlike the bourdon tube elements, bellows are often incorporated in control devices rather than as stand-alone devices. 4th Class Edition 3 • Part A 2-9 Unit A-9 • Energy Plant Instrumentation and Controls Figure 8 illustrates a bellows used as a low-pressure device. Pressure exerted on the outside of the bellows tends to compress it, stretching the calibration spring. The pressure moves the pen linkage on a chart an amount that is proportional to the applied pressure. When the maximum design pressure is reached, the bellows contacts the over-range tube. This protects the linkage and recording parts from damage. Figure 8 – Low-Range Bellows Element Pen Linkage Over-Range Tube Pivot Bellows Zero Adjustment Pressure Tension Calibrating Spring Strain Gauges A strain gauge is an extremely sensitive transducer that can be used to measure pressure or force exerted on a structure or another type of equipment. This pressure or force is converted into a proportional electrical signal. The most common type of strain gauge is the resistance type similar to the one in Figure 9(a). It has a fine wire grid, about the size of a small postage stamp, which is cemented to a paper or plastic base. This base is then bonded firmly to the column or shaft on which the force is measured. When a tensile force is applied, the wire grid will increase in length, while its cross-sectional area will decrease. These two physical changes cause the electrical resistance of the conductor to increase. If the conductor is part of the Wheatstone bridge circuit, the voltage imbalance across the bridge will be proportional to the pressure or force. Since the output of a strain gauge is electrical, these devices are well suited as sensing elements for control loops that use electronic signals. Figure 9(b) shows a strain gauge that can be used to measure the pressure in a cylinder. A change in pressure on the flush diaphragm varies the compressive force on the tube on which the strain gauge is bonded. This causes a proportional change in the electrical resistance of the strain gauge. The imbalance of voltage across the bridge is amplified to provide sufficient power to operate a controller or a recorder. 2-10 4th Class Edition 3 • Part A Introduction to Process Measurement • Chapter 2 Figure 9 – Strain Gauges To Electrical Bridge Force Cooling Air Strain Tube Base Strain Wire Strain Gauge Grid Flush Diaphragm Force Pressure (a) (b) Pressure Sensors An electronic pressure sensor is usually a transmitter that converts pressure changes into a variable voltage, current, or resistance that can be used by an electronic controller. The bourdon tubes, diaphragms, and bellows already discussed can be combined with variable resistors, piezoelectric devices, or strain gauges to transmit pressure variations to controllers. Figure 10 shows a pressure sensitive flexible diaphragm with an attached strain gauge. The strain gauge resistance changes when pressure causes the diaphragm to flex. The slight changes in resistance are detected, amplified, and transmitted to a control device. The sensor thus becomes a transmitter. Figure 10 – Resistance Type Pressure Sensor Pressure Inlet Flexible Diaphram Strain Gauge Assembly Amplifier 4th Class Edition 3 • Part A 2-11 Unit A-9 • Energy Plant Instrumentation and Controls Protecting Pressure Sensing Elements from Process Conditions Special devices are used to protect pressure-sensing elements against such conditions as high temperature, cyclic pressures, vibrations, and corrosive or congealing fluids. The inexpensive device shown in Figure 11 has a U-shaped tube filled with a sealing fluid that prevents process fluid from entering the pressure-sensing pipe and the sensor. The seal fluid must have a greater density than the process fluid for this system to work. Figure 11 – Pressure Sensor Liquid Seal Process Piping Pressure Gauge Seal Liquid Steam pressure gauges are installed with siphons (commonly called pigtails) (Figure 12). Siphons function the same as liquid seals, like that shown in Figure 11. However, siphons are shaped in a spiral pattern instead of a “U”. Figure 12 – Pressure Gauge Mounted on a “Pigtail” Siphon Isolation Valve Pigtail The pigtail siphon maintains a water seal between the steam in the boiler and the bourdon tube in the pressure gauge. This seal prevents steam from entering the bourdon tube: the high temperature of the steam affects the accuracy of the gauge, and can have a detrimental effect on the gauge material. When installing a gauge and siphon on a boiler, it is important to first fill the siphon with water. This prevents steam from reaching the bourdon tube. 2-12 4th Class Edition 3 • Part A Introduction to Process Measurement • Chapter 2 A chemical seal containing a diaphragm (Figure 13) can also be used to protect a pressure-sensing device from process fluids. The chemical seal is made of a diaphragm sandwiched between two flanges. The space above the diaphragm contains sealing fluid. The diaphragm prevents process fluid from entering the pressure sensor. Figure 13 – Diaphragm-Style Chemical Seal Chemical Seal (Courtesy of Winters) In many different processes, equipment such as reciprocating and metering pumps may produce damaging pressure pulsations. This effect can be lessened by a pulsation dampener (or snubber), as shown in Figure 14. Figure 14 – Line Pulsation Dampeners – Felt Plug & Needle Valve Felt Plug Rubber Bulb Felt Plug (a) Needle Valve (b) The pulsation dampener in Figure 14(a) consists of a glycerin-filled rubber bulb and a felt plug that connect to a pressure gauge. When the pressure from the process fluid increases, the rubber bulb is compressed. This causes the glycerin to be forced through the felt plug, which restricts the flow. The degree of damping depends on the compression of the felt plug that is adjusted before the bulb is filled with glycerin. 4th Class Edition 3 • Part A 2-13 Unit A-9 • Energy Plant Instrumentation and Controls Another practical means of restricting pulsations in the line leading to the pressure gauge can be achieved by means of a needle valve (Figure 14 (b)), which is placed between the pressure source and the gauge. Pressure Gauge Testing Pressure gauges may lose their accuracy due to wear and tear in the moving parts, metal fatigue of the bourdon tube, excessive vibration, or abuse. In many cases, loss of accuracy becomes obvious when the pointer does not return to the zero mark after the pressure is released. Some of the methods used to check the accuracy of pressure gauges include: • Test gauge (master gauge) comparison • Dead-weight tester • Hydraulic comparator Test Gauge Comparison A boiler pressure gauge may be tested while the boiler is in service by connecting an accurate test (or master) pressure gauge to the test connection, and comparing the reading of the boiler gauge to that of the test gauge. This is done by boiler inspectors during their inspections. However, this method only compares the gauges at a particular boiler operating pressure, not over the entire measuring range of the gauge. Figure 15 shows a test gauge for pressures up to 200 kPa. Figure 15 – Master Pressure Gauge 2-14 4th Class Edition 3 • Part A Introduction to Process Measurement • Chapter 2 Dead Weight Tester Dead weight testers (Figure 16) check the accuracy of pressure gauges through their entire measurement ranges. A dead weight tester consists of a hydraulic cylinder filled with oil, a hand pump, and a piston and platform assembly. A number of accurately machined weights are supplied, which fit upon the platform. Figure 16 – Dead Weight Tester Gauge being tested Oil Pump Piston Platform Weights Cylinder The gauge to be tested must be removed from the boiler and connected to the dead weight tester. The oil pump is then operated to increase the pressure in the cylinder sufficiently to raise the piston platform assembly off its bottom stop. The force exerted on the oil by the weight of the piston-platform assembly is equal to the force exerted by the pressurized oil acting upon the piston. In other words, the oil under pressure balances the platform assembly. This pressure will be indicated on the gauge. By placing weights (which are stamped in equivalent units of pressure, rather than mass) on the platform, the pressure in the cylinder may be increased. The pressure shown by the gauge should increase accordingly, if the gauge is accurate. For example, if the weight of the piston-platform assembly has a pressure equivalent of 50 kPa, the gauge will indicate 50 kPa at the beginning of the test. If weights with a 100 kPa pressure equivalent are placed one-by-one on the platform, the gauge pressure should increase by 100 kPa steps until the end of the pressure range is reached. When performing the test, the platform and piston assembly should be rotated slightly to reduce the effect of friction between piston and cylinder. 4th Class Edition 3 • Part A 2-15 Unit A-9 • Energy Plant Instrumentation and Controls Hydraulic Comparator A less accurate, but still valid method of testing a pressure gauge is by using a hydraulic comparator (Figure 17). Both the gauge to be tested and a master gauge are attached to a cylinder. Pressure is produced within the cylinder with a hand pump, and the readings of the two gauges are compared. The accuracy of this method depends on the accuracy of the master gauge. Figure 17 – Hydraulic Comparator Master Gauge Gauge being tested Isolation Valve Handle for hydraulic pressure In both the dead weight tester and the hydraulic comparator, the liquid used to transmit the pressure is usually a medium-weight oil. Any plant that is in a remote location, or uses a large number of pressure gauges, should have a comparator or a dead weight tester for pressure instrument calibration. However, many smaller plants do not have this testing equipment. In this case, the operator is advised to send the gauges out for regular calibration to a reputable firm that specializes in this type of work. 2-16 4th Class Edition 3 • Part A