Membrane Transport - BCCB2004 (Curtin University) PDF
Document Details
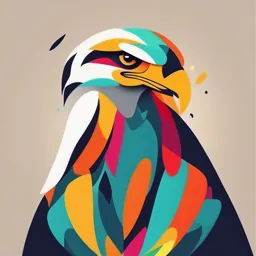
Uploaded by DecisiveMorningGlory
Curtin University
Carl Mousley
Tags
Summary
This document provides lecture notes on membrane transport for an undergraduate-level cell biology course at Curtin University. The notes cover various aspects of membrane transport mechanisms, including diffusion, facilitated diffusion, and active transport, with examples of different types of transport.
Full Transcript
3. Membrane Transport BCCB2004 – Foundations of Cell Biology Dr. Carl Mousley [email protected] 305.117 Ext 5617 WARNING This material has been reproduced and communicated to you by or on behalf of Curtin University in accordance with section 113P of the Copyright Act 1968 (the Act) The mat...
3. Membrane Transport BCCB2004 – Foundations of Cell Biology Dr. Carl Mousley [email protected] 305.117 Ext 5617 WARNING This material has been reproduced and communicated to you by or on behalf of Curtin University in accordance with section 113P of the Copyright Act 1968 (the Act) The material in this communication may be subject to copyright under the Act. Any further reproduction or communication of this material by you may be the subject of copyright protection under the Act. Do not remove this notice. Outline ◼ Understand the importance of membrane transport ◼ Understand the definition of diffusion, facilitated diffusion and active transport ◼ Understand ◼ Provide what is meant by uniport, symport and antiport biochemical examples of diffusion, facilitated diffusion and active transport The importance of membrane transport ◼ Acquire raw materials for biosynthesis and energy production ◼ Release by-products and waste products of metabolism ◼ Protect interior from potential external toxic surroundings ◼ Allow selective transport of important (polar and ionic) molecules through the semipermeable non-polar membrane Created with BioRender.com The importance of membrane transport Simple diffusion Passive (energy independent) Facilitated diffusion Active (energy dependent) Active transport The smaller the molecule & the less strongly it associates with H2O the more readily it will diffuse across a bilayer Simple diffusion ◼ Solute moves from region of high concentration to region of low concentration (down it’s concentration gradient) until concentration is equalised ◼ O2, CO2, lipid molecules, ethanol diffuse across most cell membranes ◼ Lipid (hydrophobic) solutes diffuse faster than polar solutes Created with BioRender.com Simple diffusion ◼ Extent of the concentration gradient: ◼ The greater the difference in concentration, the more rapid the diffusion. The closer the distribution of the material gets to equilibrium, the slower the rate of diffusion becomes. ◼ Mass of the molecules diffusing: ◼ ◼ Heavier molecules move more slowly; therefore, they diffuse more slowly and vice versa Temperature: ◼ Higher temperatures increase the energy and therefore the movement of the molecules, increasing the rate of diffusion and vice versa Simple diffusion ◼ Solvent density: ◼ As the density of a solvent increases, the rate of diffusion decreases. The molecules slow down because they have a more difficult time getting through the denser medium. ◼ Cells primarily use diffusion to move materials within the cytoplasm, any increase in the cytoplasm’s density will inhibit the movement of the materials. ◼ Solubility: ◼ Nonpolar or lipid-soluble materials pass through plasma membranes more easily than polar materials, allowing a faster rate of diffusion. ◼ ◼ Molecules must shed their solvation shell prior to dissolving in the core of the membrane Surface area and thickness of the membrane: ◼ Increased surface area increases the rate of diffusion, whereas a thicker membrane reduces it. Simple diffusion How has biology increased the rate of transport of highly polar molecules? Created with BioRender.com Concentration gradient Facilitated diffusion Created with BioRender.com Facilitated diffusion ◼ Protein ◼ Transporters (Carriers) transport 102 to 104 molecules per second ◼ Protein Channels (Pores / Permeases) ◼ transport 107 to 108 ions per second Created with BioRender.com Types of transport Transport one kind of solute Cotransport 2 solutes in the same direction Cotransport 2 solutes, at the same time, in opposite directions Created with BioRender.com Facilitated diffusion - transporters ◼ Carrier proteins change shape (conformational change) when molecules bind to them, and this change in shape enables the molecule to cross the membrane Facilitated diffusion - transporters ◼ Show specificity towards solute (substrate) molecule ◼ They bind ‘substrate’ via non-covalent interactions ◼ They exist in two conformations ◼ They can become saturated with substrate (Michaelis-Menton kinetics) ◼ The kinetics of carrier facilitated diffusion is analogous to kinetics of enzyme catalysed reactions ◼ They can be inhibited competitively Facilitated diffusion - transporters ◼ Membrane Vo = Vmax [S]out Kt + [S]out transporters / carriers become saturated if the concentration of molecules to be transported is too high Saturation = when all of the solute binding sites are occupied Created with BioRender.com Facilitated diffusion - transporters ◼ Hydrated solute represents the lowest energy state ◼ Breaking the solvation layer requires energy ◼ Significant energy is required to dissolve the solute in the hydrophobic core of the membrane ◼ Hydrated solute represents the lowest energy state ◼ The carrier forms non-covalent interactions with the solute to replace H2O ◼ Transporter / carrier provides a hydrophilic passage across the membrane Nelson & Cox, 2000 Facilitated diffusion - transporters ◼ Hydrated solute represents the lowest energy state ◼ Breaking the solvation layer requires energy ◼ Significant energy is required to dissolve the solute in the hydrophobic core of the membrane Transporters / carriers reduce the high Hydrated solute represents the activation energy for hydrophilic solute to lowest energy state cross hydrophobic core of lipid The bilayer carrier forms non-covalent ◼ ◼ interactions with the solute to replace H2O ◼ Transporter / carrier provides a hydrophilic passage across the membrane Nelson & Cox, 2000 Example – GLUT2 glucose transporter ◼ GLUT2 transports glucose from the intestinal epithelia into the capillary vessel beneath the basal membrane Created with BioRender.com Facilitated diffusion - channels Created with BioRender.com Facilitated diffusion - channels ◼ Protein ◼ Transporters (Carriers) transport 102 to 104 molecules per second ◼ Protein Channels (Pores / Permeases) ◼ transport 107 to 108 ions per second Rate of flux through channels is approximately 104 times greater than through transporters Created with BioRender.com Facilitated diffusion - channels ◼ Show limited specificity ◼ They discriminate between molecules on the basis of steric factors (size, volume, and orientation of solute molecule) ◼ They conduct mostly water and ions ◼ They exist in two conformations ◼ They are not saturated by solute ◼ They can be gated (open/closed) by ligand / voltage / touch ◼ Ionophores can behave as channels Facilitated diffusion - channels Open & closed by binding a specific ligand e.g. Neurotransmitter activated ion channels Open & closed by mechanical stress e.g. Cation channels of the stereocilliar (ear) Selectivity filter Open & closed by changes in membrane potential e.g. activated by membrane depolarisation in neurons Created with BioRender.com Facilitated diffusion - channels K+ channel Ionic radius Na+ 98 pm K+ 133 pm A Na+ ion is too small to get assistance from the carbonyl oxygens Transport of water Paracellular transport of water (Diffusion) Transcellular transport of water (three mechanisms) Transport of water Krane & Kishore 2003 Aquaporins important for: Diffusion (very slow) cell migration (provide water for lubrication), brain water transport and hence function, Cotransport with solutes (Facilitated Diffusion) kidney tubule absorption of water, Specific Water Channels Aquaporins (Facillitated Diffusion) (Rapid and selective) Aquaporin Ionophores ‘Ion carriers’ ◼ A chemical species that reversibly binds ions ◼ Contains a hydrophilic centre and a hydrophobic portion that interacts with the membrane ◼ Many ionophores are lipid-soluble molecules that transport ions across membranes Ionophores Gramicidin ◼ Antimicrobial ◼ Structure ◼ peptide with alternating D and L amino acids! is a ‘b-helix’ A ‘hole’ produced from an arrangement of the polypeptide chain in a helix-like structure ◼ Forms a channel large enough for H+, Na+ and K+ ions to pass through, but not Ca2+ Gramicidin (side view) Gramicidin (top view) Ionophores Valinomycin ◼ Antimicrobial ◼ Binds peptide produced by Streptomyces spp. to K+ with high specificity ◼ Functions as a K+ transporter Thermal dynamics of diffusion C1 C2 Created with BioRender.com ◼ Diffusion is governed by the second law of thermal dynamics ◼ There is free energy stored in concentration gradients and it can be quantified ◼ Free energy of transporting material across membrane (ΔGt) depends on concentration gradient across membrane: ΔGt = R T ln C2 / C1 R = Ideal Gas Constant (8.314 x 10 -3 kJ.K-1.mol-1) T = Temp in K Created with BioRender.com Thermal dynamics of diffusion C1 C2 Created with BioRender.com For passive diffusion to proceed ΔGt must be -ve C1 C2 ΔGt = R T ln (C2 / C1) R = Ideal Gas Constant (8.314 x 10 -3 kJ.K-1.mol-1) T = Temp in K C1 > C2 C1 = C2 C1 < C2 C2/C1 < 1 ln < 1 = -ve C2/C1 = 1 ln 1 = 0 C2/C1 > 1 ln > 1 = +ve - ΔGt ΔGt = 0 ΔGt Created with BioRender.com Thermal dynamics of diffusion For passive diffusion to proceed ΔGt must be -ve ΔGt = R T ln (C2 / C1) R = Ideal Gas Constant (8.314 x 10 -3 kJ.K-1.mol-1) T = Temp in K Will Na+ diffuse from C1 C2? Will Cl - diffuse from C1 C2? Will K + diffuse from C1 C2? Created with BioRender.com Thermal dynamics of diffusion For passive diffusion to proceed ΔGt must be -ve ΔGt = R T ln (C2 / C1) Will K + diffuse from C1 R = Ideal Gas Constant (8.314 x 10 -3 kJ.K-1.mol-1) T = Temp in K C2? 2 gradients exist across this theoretical membrane; 1. Chemical & 2. Electrical The chemical gradient is favourable The electrical gradient is unfavourable (the +ve charge at C2 will repel K+) Thermal dynamics of diffusion Chemical gradient Electrical gradient e.g. Na+ For passive diffusion to proceed ΔGt must be -ve ΔGt = R T ln (C2 / C1) Will K + diffuse from C1 R = Ideal Gas Constant (8.314 x 10 -3 kJ.K-1.mol-1) T = Temp in K C2? 2 gradients exist across this theoretical membrane; 1. Chemical & 2. Electrical The chemical gradient is favourable No energy is needed The electrical gradient is unfavourable (the +ve charge at C2 will repel K+) Thermal dynamics of diffusion For passive diffusion to proceed ΔGt must be -ve ΔGt = R T ln (C2 / C1) Will K + diffuse from C1 R = Ideal Gas Constant (8.314 x 10 -3 kJ.K-1.mol-1) T = Temp in K C2? The unfavourable membrane potential makes ΔGt become +ve ΔGt = R T ln (C2 / C1) + Z F Δ V ΔGt = PASSIVE +ΔG t F = Faraday Constant (96.5 kJ.V-1.M-1) Z = Charge of transported species = ACTIVE (REQUIRES ENERGY) Created with BioRender.com Direct (Primary) Active Transport ◼ Uses metabolic energy to directly transport molecules / ions across a membrane (endergonic) ◼ Promotes the movement of molecules / ions against it’s electrochemical gradient ◼ Estimated 30-50% of cellular ATP fuels active transport processes Created with BioRender.com Direct (Primary) Active Transport Four general types of ATPase transporters ◼ P-type ATPases ◼ Various ◼ Some ◼ V-type Plasma membrane transporters of Na+, K+, Ca2+ and H+ bacterial ATPases transport Cd2+ , Hg2+ , Cu2+ ATPases ◼ Transports H+ to create low pH in Vacuoles Direct (Primary) Active Transport Four general types of ATPase transporters ◼ F-type ATPases ◼ Formation ◼ Transports ◼ ABC of ATP from ADP + Pi H+ Superfamily ◼ ‘ATP Binding Cassette’ transporters ◼ Also called Multidrug transporters ◼ transports various hydrophobic type drugs ◼ Responsible for flipping phospholipids from one side of membrane to the other Direct (Primary) Active Transport Maintaining the membrane potential at the resting state (-70mV) is key to the ability to generate an action potential Created with BioRender.com Direct (Primary) Active Transport Maintaining the membrane potential at the resting state (-70mV) is key to the ability to generate an action potential Created with BioRender.com Direct (Primary) Active Transport Created with BioRender.com Direct (Primary) Active Transport ◼ Na+ and K+ gradients (established by Na+-K+ ATPase) are used to drive other cellular processes ◼ Na+ and K+ gradients: ◼ control ◼ make ◼ drive cell volume neurons and muscle cells electrically excitable active transport of other solutes like some sugars and amino acids ◼ Na+ and K+ gradients so important that more than 1/3 of the ATP consumed by resting animal is used to pump these ions! ◼ Drugs that treat congestive heart failure, Digitalis and Ouabain, inhibit Na+-K+ ATPase Indirect (Secondary) Active Transport ◼ Co-transport (symport or antiport) ◼ where the transport of one solute molecule (ion) is coupled to the transport of another solute molecule (ion) ◼ Simultaneous ◼ the transport of two molecules across a membrane coupling of the solute molecules is obligatory ◼ transport absent cannot occur if one of the co-transported solute molecules is Indirect (Secondary) Active Transport ◼ Na+ driven glucose symport channel (restricted to certain cell types) ◼ Uses the energy from this downhill sodium ion gradient created by the ATPase pump to transport glucose across the apical membrane, against an uphill glucose gradient. From Na+ K+ ATPase Indirect (Secondary) Active Transport Gut epithelium and Na+ symport via the Na+ driven glucose symport channel ◼ Glucose ◼ Passive transport of Glucose via GluT2 into extracellular fluid (blood) transport of Na+ via the Na+ K+ pump ◼ Active Indirect (Secondary) Active Transport ◼ E. coli lactose permease (galactoside permease), utilises proton + gradient generated by H+ pumps to symport lactose and HCreated with BioRender.com. Indirect (Secondary) Active Transport H+ binding triggers conformational change revealing the lactose binding site ◼ E. Berg, Tymoczko and Stryer, 6th ed. Fig. 13.12: coli lactose permease (galactoside permease), utilises proton gradient generated by H+ pumps to symport lactose and H+. Indirect (Secondary) Active Transport The Cl- - HCO-3 exchanger allows the entry and exit of HCO-3 without changes in electrical potential. The role of this shuttle system is to increase the CO2-carrying capacity of the blood. Respiring tissue ◼ The CO2 produced by respiration enters erythrocytes via diffusion ◼ CO2 is converted to HCO3- (more soluble) by carbonic anhydrase ◼ HCO3- is exchanged with Cl- by the Band3 antiport channel. Created with BioRender.com Indirect (Secondary) Active Transport The Cl- - HCO-3 exchanger allows the entry and exit of HCO-3 without changes in electrical potential. The role of this shuttle system is to increase the CO2-carrying capacity of the blood. ◼ HCO3 - is re-imported into erythrocytes, Lungs at the expense of Cl-, by the Band3 antiport channel ◼ HCO3- is converted to CO2 by carbonic anhydrase ◼ CO2 diffuses out of the erythrocyte and is exhaled. Created with BioRender.com Bulk transport ◼ Diffusion, facilitated diffusion and active transport allow the movement of small molecules and ions ◼ Bulk transport allows the movement of large macromolecules. Fusion and secretion processes Endocytosis and exocytosis non-specific receptor mediated Exocytic Endocytic Summary ◼ Understand the importance of membrane transport ◼ Define diffusion, facilitated diffusion and active transport ◼ Define what is meant by uniport, symport and antiport ◼ Discuss biochemical examples of diffusion, facilitated diffusion and active transport