Citric Acid Cycle PDF
Document Details
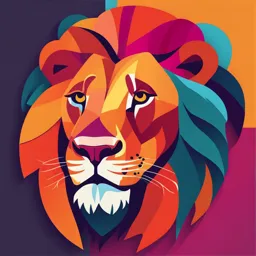
Uploaded by StylishAccordion
The University of Zambia
Shari Babu
Tags
Summary
This document provides comprehensive information about the Citric Acid Cycle, also known as the Krebs cycle. It details the cycle's biomedical importance in cellular respiration, explaining the reactions, regulation, and deficiencies. The document includes diagrams and explanations of different stages and components.
Full Transcript
CITRIC ACID CYCLE SHARI BABU [email protected] Biomedical Importance The citric acid cycle (Krebs cycle, tricarboxylic acid cycle) is the second stage of cellular respiration. It is a series of reactions in mitochondria that oxidize acetyl residues (as acetyl-CoA)....
CITRIC ACID CYCLE SHARI BABU [email protected] Biomedical Importance The citric acid cycle (Krebs cycle, tricarboxylic acid cycle) is the second stage of cellular respiration. It is a series of reactions in mitochondria that oxidize acetyl residues (as acetyl-CoA). The acetyl groups are fed into the citric acid cycle, which enzymatically oxidizes them to CO2. The energy released by oxidation is conserved in the reduced electron carriers NADH and FADH2. It is the final common pathway for the aerobic oxidation of carbohydrate, lipid, and protein because glucose, fatty acids, and most amino acids are metabolized to acetyl-CoA or intermediates of the cycle. It also has a central role in gluconeogenesis, lipogenesis, and interconversion of amino acids. Catabolism of proteins, fats, and carbohydrates in the three stages of cellular respiration. Transport and Production of Acetyl-CoA Pyruvate generated in the cytoplasm by glycolysis must be transported across the inner mitochondrial membrane via a pyruvate/H+ symport. This transport uses some of the energy stored in the mitochondrial inner membrane electrical potential gradient. Pyruvate derived from glucose and other sugars by glycolysis, is oxidized to acetyl-CoA and CO2 by the pyruvate dehydrogenase (PDH) complex, located in the mitochondria of eukaryotic cells and in the cytosol of prokaryotes. The overall reaction catalyzed by the pyruvate dehydrogenase complex is an oxidative decarboxylation, an irreversible oxidation process in which the carboxyl group is removed from pyruvate as a molecule of CO2 and the two remaining carbons become the acetyl group of acetyl-CoA. The Pyruvate Dehydrogenase Complex Consists of Three Distinct Enzymes The PDH complex contains three enzymes—pyruvate dehydrogenase (E1), dihydrolipoyl transacetylase (E2), and dihydrolipoyl dehydrogenase (E3). The Pyruvate Dehydrogenase Complex Requires Five Coenzymes They are thiamine pyrophosphate (TPP), flavin adenine dinucleotide (FAD), coenzyme A, nicotinamide adenine dinucleotide (NAD), and lipoate. The active site of E1 has bound TPP, E2 is the point of connection for the prosthetic group lipoate, and E3 has bound FAD. Deficiencies of thiamine can cause serious central nervous system problems. This is because brain cells are unable to produce sufficient ATP (via the TCA cycle) if the PDH complex is inactive. Beriberi, a disease that results from thiamine deficiency, is characterized by loss of neural function. Wernicke-Korsakoff, an encephalopathy-psychosis syndrome due to thiamine deficiency, may be seen with alcohol abuse. An elevated level of pyruvate in the blood is often an indicator of defects in pyruvate oxidation due to one of these causes. Regulation of acetyl-CoA production by the PDH complex When AMP, CoA, and NAD+ accumulate, they allosterically activate the pyruvate dehydrogenase complex. The PDH complex is inhibited by reversible phosphorylation of E1. A specific protein kinase called PDH kinase, phosphorylates and inactivates E1. PDH kinase is activated by ATP, acetyl-CoA and NADH. PDH kinase is inhibited by pyruvate. A specific phosphatase called PDH phosphatase, removes the phosphoryl group by hydrolysis and thereby activates E1. Calcium is a strong activator of PDH phosphatase Pyruvate dehydrogenase deficiency: A deficiency in the E1 component of the PDH complex is the most common biochemical cause of congenital lactic acidosis. This enzyme deficiency results in accumulation of pyruvate, causing it to be converted to lactic acid. This causes problems for the brain, which relies on the TCA cycle for most of its energy, and is particularly sensitive to acidosis. Symptoms are variable and include neurodegeneration, muscle spasticity and, in the neonatal onset form, early death. The E1 defect is X-linked it affects both males and females, and is classified as X- linked dominant. There is no proven treatment for PDH deficiency: however, dietary restriction of carbohydrate and supplementation with TPP may reduce symptoms in select patients. Reactions of the Citric Acid Cycle The Citric Acid Cycle Has Eight Steps 1. Formation of Citrate: The first reaction of the cycle is the condensation of acetyl-CoA with oxaloacetate to form citrate, catalyzed by citrate synthase: The CoA liberated in this reaction is recycled to participate in the oxidative decarboxylation of another molecule of pyruvate by the PDH complex. 2. Formation of Isocitrate via cis-Aconitate: Citrate is isomerized to isocitrate by the enzyme aconitase. Aconitase is inhibited by fluoroacetate. 3. Oxidation of Isocitrate to α-Ketoglutarate and CO2: In the next step, isocitrate dehydrogenase catalyzes oxidative decarboxylation of isocitrate to form α-ketoglutarate. This is one of the rate-limiting steps of the TCA cycle. The enzyme is allosterically activated by ADP and Ca2+, and is inhibited by ATP and NADH. 4. Oxidation of -Ketoglutarate to Succinyl-CoA and CO2: The next step is another oxidative decarboxylation, in which α- ketoglutarate is converted to succinyl-CoA and CO2 by the action of the α-ketoglutarate dehydrogenase complex. α-Ketoglutarate dehydrogenase complex is inhibited by its products, NADH and succinyl CoA, and activated by Ca2+. 5. Conversion of Succinyl-CoA to Succinate: Succinate thiokinase (succinyl CoA synthetase) cleaves the high-energy thioester bond of succinyl CoA. This reaction is coupled to phosphorylation of guanosine diphosphate (GDP) to guanosine triphosphate (GTP). The GTP formed can donate its phosphoryl group to ADP to form ATP, in a reversible reaction catalyzed by nucleoside diphosphate kinase. 6. Oxidation of Succinate to Fumarate: The succinate formed from succinyl-CoA is oxidized to fumarate by the flavoprotein succinate dehydrogenase: In eukaryotes, succinate dehydrogenase is tightly bound to the inner mitochondrial membrane, and is the only enzyme of the citric acid cycle that is membrane-bound. Malonate, an analog of succinate, is a strong competitive inhibitor of succinate dehydrogenase and therefore blocks the citric acid cycle. 7. Hydration of Fumarate to Malate: The reversible hydration of fumarate to L-malate is catalyzed by fumarase. 8. Oxidation of Malate to Oxaloacetate: In the last reaction of the citric acid cycle, NAD+-linked L-malate dehydrogenase catalyzes the oxidation of L-malate to oxaloacetate: Products of one turn of the citric acid cycle. Three NADH, one FADH2, one GTP (or ATP), and two CO2 are released in oxidative decarboxylation reactions. The cycle generates the equivalent of 12 ATPs from one acetyl- CoA (3 NADH = 9 ATPs, 1 FADH2 = 2 ATPs, 1 ATP (GTP) directly). Arsenic Poisoning Arsenic poisoning” is primarily due to inhibition of enzymes that require lipoic acid as a coenzyme, including E2 of the PDH complex and α-ketoglutarate dehydrogenase. Arsenic forms a stable complex with the thiol (–SH) groups of lipoic acid, making that compound unavailable to serve as a coenzyme. When it binds to lipoic acid in the PDH complex, pyruvate (and consequently lactate) accumulates. Like pyruvate dehydrogenase deficiency, this particularly affects the brain, causing neurologic disturbances and death. Citric Acid Cycle Components Are Important Biosynthetic Intermediates α-Ketoglutarate and oxaloacetate can, for example, serve as precursors of the amino acids aspartate and glutamate by simple transamination. Through aspartate and glutamate, the carbons of oxaloacetate and α-ketoglutarate are then used to build other amino acids, as well as purine and pyrimidine nucleotides. Oxaloacetate is converted to glucose in gluconeogenesis. Succinyl-CoA is a central intermediate in the synthesis of the porphyrin ring of heme groups, which serve as oxygen carriers (in hemoglobin and myoglobin) and electron carriers (in cytochromes). And the citrate produced in some organisms is used commercially for a variety of purposes. Biosynthetic precursors produced by Citric Acid Cycle