Vaccine Antigens 2 PDF
Document Details
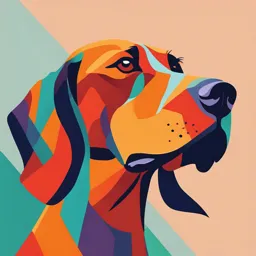
Uploaded by SpellboundLove
Universiteit Gent
Tags
Summary
This document provides an overview of vaccine antigens, exploring different types of vaccines and their underlying technologies. It examines strategies for selecting antigens, focusing on pathogen virulence determinants. The document explains the different methods utilized to produce vaccines, from inactivated to live-attenuated and subunit vaccines.
Full Transcript
2.2. Vaccine antigens Definitions: Antigen – immunogen Antigen: structure with “in vitro” immunologic reactivity; derived from “antibody-generator” as the ligands of antibodies. Idea developed by Paul Ehrlich from his work on toxin-antitoxin complexes in sera and the recognition of antibody in 189...
2.2. Vaccine antigens Definitions: Antigen – immunogen Antigen: structure with “in vitro” immunologic reactivity; derived from “antibody-generator” as the ligands of antibodies. Idea developed by Paul Ehrlich from his work on toxin-antitoxin complexes in sera and the recognition of antibody in 1890. Immunogen: structure that induces “in vivo” immune response Antigen + “defensive triggers” are needed for a subsequent efficient induction of an innate and adaptive immune response Paul Ehrlich Antigen discovery Evolving scientific expertise Empirical observationbased approach Jenner (variolation) Pathogen-based antigen Non-replicating (whole inactivated pathogen) Replicating (live-attenuated pathogen) Pathogen toxin Inactivated toxin (toxoids) No knowledge of the pathogen causing the disease Modified pathogens, dead pathogens, or inactivated pathogen toxins can be immunogenic Pathogen subunit antigen (split virus, fragments of pathogens) Purified antigens (protein antigens, recombinant proteins) Nucleic-acid based vaccines (DNA, viral vector, RNA) Pathogen-derived material can be immunogenic The immunogen can be produced by our own cells Most vaccine technologies are still in use today… Vaccine antigens: how to select an antigen? • Many (older) vaccines comprise whole viruses or bacteria • Newer vaccines are based on other, safer approaches • The novel technologies (viral vectors, mRNA) have important advantages with regard to production speed and cost. • Key pathogen virulence determinants are usually excellent antigens such as toxins, hemagglutinins, proteins involved in viral attachment/entry or bacterial polysaccharides • The final choice of antigen is often determined by what is technologically achievable and what is optimal from a safety and immunological perspective. Overview of vaccine types Pollard and Bijker. Nature Rev Immunol 2021; 21: 83-100 Whole pathogen antigens Passage in different cell lines Live-attenuated vaccine e.g. MMR(-V) vaccine, OPV, rotavirus vaccine MMR-V: measles mumps, rubella, varicella vaccine OPV: oral polio vaccine; Pw: whole cell pertussis vaccine IPV: inactivated (injectable) polio vaccine; HAV: hepatitis A vaccine Infectious pathogen Heat Chemicals (e.g. formaldehyde, glutaraldehyde) Killed/inactivated vaccine e.g. IPV, HAV, Pw Live-attenuated vaccine Infectious pathogen Wild virus is replicated in unfavourable conditions in cell culture The process is repeated several times … … to produce an attenuated strain that is unable to cause disease Live-attenuated vaccine e.g. BCG (Bacille Calmette-Guérin) vaccine: 231 passages over 13 years Variant: Reassortant live-attenuated vaccines 1990s: new vaccine design technologies including reassortment via co-infection with wild and attenuated virus strains allows ‘swapping’ of genome segments Bovine rotavirus Human rotavirus • Human/bovine reassortant rotavirus vaccine: human antigens on a bovine virus core • Influenza viruses: influenza virus of interest reassorted with the high yield PR8 backbone Human Human/bovine reassortment Killed/inactivated vaccines Infectious pathogen The virus is grown under suitable conditions It is purified … Killed/inactivated vaccine … and treated with heat or chemicals … … so that it is inactivated but still immunogenic Advantages and disadvantages of live-attenuated vs. inactivated vaccines Live-attenuated vaccines Killed/inactivated vaccines (e.g. OPV, MMR, VZV, BCG) (e.g. IPV, HAV, whole-cell pertussis) Mimic the natural infection and retain most defensive triggers/immunogenic elements; may retain immune evasion factors Strong priming usually achieved with 1–2 doses Long-term persistence of immunity May induce mild disease symptoms Rare reversion to virulence; unsuitable for immunocompromised subjects Potential for immunological interference with other live vaccines Less stable over time, heat labile Affected by recent administration of blood or blood-derived products or presence of maternal antibodies in infants • Usually require adjuvants due to reduced immunogenicity/missing defensive triggers • Multiple doses usually required for priming • Booster doses may be needed to maintain long-term immunity • Do not induce disease symptoms • No risk of re-activation, non-infectious; suitable for immunocompromised subjects • Low risk of immunological interference • Relatively stable over time, better resistance to cold chain deviation • Generally not affected by administration of blood or blood-derived products OPV, oral polio vaccine; MMR, measles-mumps-rubella; VZV, varicella zoster virus vaccine; BCG, Bacille Calmette–Guérin; IPV, inactivated polio vaccine; HAV, hepatitis A virus vaccine Toxoid vaccines - Toxoids are inactivated derivatives of toxins - Toxoid-based vaccines are considered as a subtype of subunit vaccines - Tetanus and diphtheria vaccines only target the toxin and do not prevent the bacterium from infecting the host Toxic groups Toxin Toxoid - Toxins are the disease-mediating factors Antigenic determinants Clostridium tetani Corynebacterium diphteriae Antigenic determinants induce antibody formation Examples: Tetanus toxoid < tetanus toxin (= tetanospasmin) Diphteria toxoid < diphteria toxin Pertussis toxoid < pertussis toxin (Bordetella pertussis) Subunit vaccines Whole pathogen vaccine • Highly focused, specific response • Non-infectious, low reactogenicity • Often reduced immunogenicity vs. whole pathogen • Adjuvants often needed to compensate for lower immunogenicity • Various types: • Purified protein (split or subunit, see figure) • Recombinant protein • Polysaccharide • Peptide (T-cell, B-cell): experimental (infectious diseases, cancer, chronic diseases, e.g. Alzheimer’s disease) Pathogen fragmentation Split vaccine e.g. some influenza vaccines Subunit/ purified vaccine e.g. Pa, some influenza vaccines Pa: acellular pertussis vaccine Recombinant protein vaccines: HBV (1980s) DNA recombinant protein technology enabled a great leap in vaccine development Hepatitis B surface antigen Insert gene into yeast expression system Purification of recombinant protein encoding antigen & natural assembly into spheres Administration as vaccine Sequence gene encoding HBsAg Protein expression HBV, hepatitis B virus; HBsAg, hepatitis B surface antigen HBV Recombinant protein vaccines: HPV (2006) Self-assembly into viral capsids (virus-like particles) HPV viral capsid Self-assembly into pentamers Purification from expression system DNA encoding L1 Antigen DNA Yeast DNA HPV, human papillomavirus Transcription L1 capsid proteins Translation Elicit immune response Polysaccharide-based vaccines Antibodies against capsular polysaccharides (Ps) are protective for most encapsulated bacteria, e.g. • Neisseria meningitidis (meningococcus) • Streptococcus pneumoniae (pneumococcus) • Haemophilus influenzae type b (Hib) Polysaccharide vaccines • Natural polysaccharides are usually immunogenic in adults and children over 2 years of age because they are capable of mounting a T cellindependent B cell response • Only Ps vaccine available is 23-valent pneumococcal vaccine (PPV-23, PneumovaxTM) Peptide-based vaccines using synthetic peptide antigens Effective structure of epitopes Conformational epitopes recognised by B-cells Original antigen Recombinant peptide including conformational and linear epitopes Conformational (nonlinear) epitopes Administration of recombinant peptide vaccine to host Linear epitopes recognised by Tcells Primary structure Linear epitopes Peptide-based vaccines: B-cell epitopes - Non conformational B cell epitopes Peptides of 6-20 amino acids Easy to produce Poorly immunogenic Increase immunogenicity: several techniques, e.g. • • • • Polymerize Produce aggregates Add T cell epitope(s) = conjugation Insert in a carrier molecule (HBsAg, HBcAg): < e.g. malaria vaccine (Mosquirix TM): fusion of CSP with HBsAg* * FYI: https://www.frontiersin.org/articles/10.3389/fimmu.2019.02931/full CSP: circumsporozoite protein Peptide-based vaccines: T-cell epitopes T cell epitopes • CTL epitopes • E.g. SARS-CoV-2 vaccine candidates for selected patient populations (e.g. patients under anti-CD20 treatment) T cell receptor • TCR is target antigen • To reduce harmful T cells causing autoimmune disease (multiple sclerosis) Recombinant virus-like particles (VLPs) • VLPs are a class of structures formed by the self-assembly of viral capsid protein subunits and contain no infective viral genetic material • Particles are more immunogenic than individual proteins • Highly ordered structures induce a good immune response (antibodies) • Examples: • HBV vaccine < HBsAg • HPV vaccine < L1 capsid protein of human papilloma virus • HBcAg used as a platform to present other epitopes FYI: interesting review on Hep B core-based VLP’s, also in plant expression systems: https://www.ncbi.nlm.nih.gov/pmc/articles/PMC7937989/ Outer membrane vesicles (OMV) vaccines • Gram negative bacteria release vesicular structures from their outer membrane, calles outer membrane vesicles (OMV) which have various functions • OMV’s are promising structures for vaccine development because they carry many surface antigens that are identical to the bacterial surface • Meningococcal B vaccine (Bexsero) • Candidate vaccines against other gram negative bacteria, e.g. Bordetella pertussis, E. coli, Shigella, Pseudomonas aeruginosa, …. • Low production yield → different induction methods (e.g. detergent, stress such as heat shock) • Low cost Balhuizen et al. Front Microbiol 2021; https://doi.org/10.3389/fmicb.2021.629090 B-cell T-cell quantity Polysaccharide vaccine Antibody, no memory Conjugated protein Polysaccharideconjugate vaccine B-cell + antibody quantity Polysaccharide vs. conjugated polysaccharide vaccines T-cell Antibody and memory Polysaccharideconjugate vaccine Polysaccharide vaccine Polysaccharideconjugate vaccine No T-cell response to polysaccharide alone Pollard et al. Nat Rev Immunol 2009; 9: 213-220 Conjugated polysaccharide vaccines • Ps covalently linked to carrier protein, e.g. tetanus or diphteria toxoid (CRM197) • Against H. influenzae type B: no monovalent vaccine anymore, only as a component of the multivalent vaccine (in BE: hexavalent) • Against meningococci: MenC and MenACWY vaccine (MenB is the predominant serotype in BE, but MenB vaccine is a recombinant protein-OMV vaccine developed by reverse vaccinology → see further) • Serotypes are added FYI Against pneumococci: • 7-valent PCV (PrevenarTM): 4, 6B, 9V, 14, 18C, 19F, 23F • 10-valent PCV (Synflorix TM ): 1, 4, 5, 6B, 7F, 9V, 14, 18C, 19F, 23 F • 13-valent PCV (Prevenar 13 TM): 1, 3, 4, 5, 6A, 6B, 7F, 9V, 14, 18C, 19A, 19F, 23F • 15-valent PCV (Vaxneuvance TM): 13-PCV + 22F, 33F • 20-valent PCV (Apexxnar TM): 13-PCV + 8, 10A, 11A, 12F, 15B, 22F, 33F (ST 8 is currently predominant in Belgium, causing 15-20% cases IPD = invasive pneumococcal disease) Viral vector vaccines Pathogen Viral vectors • Adenovirus • rMVA • Canarypox virus • Yellow fever virus Gene encoding pathogen antigen identified Antigen identified Approved for Ebola and SARS-CoV-2 In development for e.g. HIV, HCV, TB, and cancer candidate vaccines Viral antigen/host MHC-1 loading Stimulation of host immunity against the pathogen rMVA, recombinant modified vaccinia Ankara; HIV, human immunodeficiency virus; HCV, hepatitis C virus; TB, tuberculosis; MHC, major histocompatibility complex Stanberry et al. Chapter 6 in: Garçon et al. Understanding Modern Vaccines, Perspectives in vaccinology, Vol 1, Amsterdam. Elsevier 2011;p151–199 Recombination with viral genome Vector entry into cell Non-pathogenic viral vector Administration to vaccine recipient Expression and possible excretion of pathogen antigens in human cells Advantages and limitations of vectored vaccines Advantages Limitations Potent immunogenicity in animal and human infectious disease High level immunity to some vectors in humans Ability to induce humoral and cell-mediated immunity Need for qualified packaging cell lines Relative ease of production for many vectors Induction of anti-vector immunity following initial injection of viral vaccines Many potential prime-boost strategies Complexity with multiple vectors in primeboost strategy Overall favorable safety profile and lack of persistence in vivo Very rare, but severe side effect TTS induced by adenoviral vectored SARSCoV-2 vaccine TTS: thrombosis with thrombocytopenia syndrome FYI: Vaccine pipeline of Vaccitech with ChAdOx and MVA viral vector platforms Vaccitech is a spinoff of the University of Oxford Nucleic acid-based vaccines (DNA and mRNA) Advantages Challenges Synthetic, rapid and easily standardizable production method Establishing proof-of-principle → In the meanwhile established for mRNA (SARS-CoV-2) → DNA? Induction of humoral and cell-mediated immunity Immunogenic potency (DNA) Favourable safety profile Rare side effect myocarditis in adolescents and young adults (mainly men) DNA vaccines - Various techniques to deliver DNA to the cells, e.g. Naked DNA, DNA liposome complexes, DNAcoated polymer, DNA-coated metal (biolistics, and electroporation - No licenced DNA vaccine to date - Challenge: disappointing immunogenicity in humans Naked DNA vaccine mRNA vaccines Drew Weissman and Katalin Kariko Courtesy of Rein Verbeke and Ine Lentacker The future = mRNA vaccines? FYI: Bacterial vector vaccines Pathogen Gene encoding pathogen antigen identified Bacterial vectors • Salmonella typhi • Vibrio cholerae • Listeria monocytogenes Recombination with bacterial plasmid DNA Antigen identified Non-pathogenic bacterial vector Tested in HIV, cholera, ETEC, and H. pylori candidate vaccines Stimulation of host immunity against the pathogen Administration to vaccine recipient Translation HIV, human immunodeficiency virus; H. pylori, Helicobacter pylori ETEC, enterotoxigenic escherichia coli Secretion For some infectious diseases (e.g. influenza) many approaches have been used. Split Adjuvanted split Subunit Adjuvanted subunit Viral components Influenza virus Whole virus, inactivated Adjuvanted whole virus Seasonal influenza vaccines Pandemic influenza vaccines Liveattenuated Reassortant Coldadapted The antigen can be produced in a cell culture system, but by our own cells as well Pathogen Clone gene encoding antigen Insert gene into expression system Purification of recombinant protein encoding antigen Administration as Protein expression vaccine Recombinant protein antigen DNA plasmid DNA plasmid Protein expressed within host cell Live vaccine vector Live vaccine vector Protein expressed in host Which vaccine type/platform is missing on this slide? Sinovac Sinopharm Valneva SARS-CoV-2 vaccine development: 7 different strategies are being used Novavax Sanofi Pasteur GSK CanSino Biologicals Aiming at inducing binding/neutralizing antibodies (spike, RBD) Aiming at inducing Strong cell-mediated immunity CTL B cell Helper T cell RBD: receptor binding domain Lianpan Dai and George F. Gao. Nature Rev Immunol 2021 Pfizer/BioNTech Moderna Curevac (now + GSK) Oxford/AstraZeneca Johnson&Johnson Sputnik V CanSino In bold: EMA licensed WHO’s COVID-19 vaccine tracker: https://www.who.int/publications/m/item/draft-landscape-of-covid-19-candidate-vaccines Reverse vaccinology Genome sequence Expression and purification Purified recombinant proteins Complete epidemiology performed on protective antigens All potential antigens Selection of final candidates for human trials Bioinformatics refinement 2000–3000 proteins Vaccination in animal model 100–200 proteins Further animal validation of least variant proteins <100 proteins Classical vs. reverse vaccinology Classical vaccinology Study relationship between pathogen and host Mutate/delete genes encoding virulence determinants Establish importance of individual determinants/antigens Identify key virulence determinants Retest the mutant pathogen in an infection model Test identified candidates for immunogenicity and safety as vaccine antigens Reverse vaccinology Test immunogenicity/ safety in a validated testing model Clone and express candidate antigens Identify genes with signatures indicative of good antigen candidates Start with the genome of a pathogen Despite the unmet need, development of a MenB vaccine was challenging and a multicomponent approach was needed The polysaccharide capsule is poorly immunogenic1,2 Multiple components enable broad coverage across a number of strains5 A single subcapsular protein component (e.g. OMV) is susceptible to antigenic variability3,4 NadA NHBA fHbp PorA Neisseria meningitidis OMV, outer membrane vesicle Images of antigenic components of 4CMenB adapted by permission from Springer Nature: Drugs, A Multi-Component Meningococcal Serogroup B Vaccine (4CMenB): The Clinical Development Program, O'Ryan M et al, Copyright 2014 1. Finne J et al. J Immunol 1987;138:4402–4407; 2. Wyle FA et al. J Infect Dis 1972;126:514–521; 3. Sadarangani M et al. Lancet Infect Dis 2010;10:112–124; 4. Tan LK et al. N Engl J Med 2010;362:1511–1520; 5. Donnelly J et al. Proc Natl Acad Sci U S A 2010;107:19490–19495 BE/BEX/0046/18(1) – December 2018 41 BEXSERO was developed using reverse vaccinology and contains four antigenic components1 Antigens are associated with meningococcal survival, function or pathogenesis, are conserved across a wide range of strains, and induce a bactericidal response1‒8 NadA NHBA fHbp (Neisseria adhesin A) (Factor H binding protein) Mediates binding to, and entry, into human epithelial cells3–5 (Neisseria heparinbinding antigen) Binds factor H, thereby enabling bacterial survival in the blood1,2 Binds heparin, which may promote bacterial survival in the blood6,7 NZ PorA P1.4 (Porin A) Major OMV protein; induces strain-specific bactericidal response in MeNZB OMV vaccine*8 Multiple antigens may provide synergistic killing and improve strain coverage9,10 *Developed by Chiron Vaccines in association with the Norwegian Institute of Public Health MeNZB, meningococcal B vaccine developed in New Zealand; OMV, outer membrane vesicle Images of antigenic components of 4CMenB adapted by permission from Springer Nature: Drugs, A Multi-Component Meningococcal Serogroup B Vaccine (4CMenB): The Clinical Development Program, O'Ryan M et al, Copyright 2014 1. Madico G et al. J Immunol 2006;177:501–510; 2. Schneider MC et al. Nature 2009;458:890–893; 3. Comanducci M et al. J Exp Med 2002;195:1445–1454; 4. Capecchi B et al. Mol Microbiol 2005;55:687–698; 5. Mazzon C et al. J Immunol 2007;179:3904–3916; 6. Serruto D et al. Proc Natl Acad Sci U S A 2010;107:3770–3775; 7. Bambini S et al. Vaccine 2009;27:2794–2803; 8. Martin DR et al. Clin Vaccine Immunol 2006;13:486–491; 9. Vesikari T et al. Lancet 2013;381:825–835; 10. Vogel U et al. Lancet Infect Dis 2013;13:416–425 BE/BEX/0046/18(1) – December 2018 42 Structure-based vaccine design works – era of “precision vaccinology” - 2014: stabilized pre-fusion conformation of HIV-1 env protein - A new era of antigen design: Rapid identification and selection of human monoclonal antibodies → atomic-level structural information for viral surface proteins → precision engineering of protein immunogens - HIV-1 vaccine development has been a driving force behind these technologies and concepts, but as yet unsuccessful in this context. - More success in the context of RSV and SARS-CoV-2 vaccine development Anthony Fauci, 2021 Beatty Lecture, YouTube Prefusion RSV candidate vaccine: proof-of-concept of how structural biology can contribute to precision vaccine design RSV vaccine development - 1960’s: formol inactivated whole virus RSV vaccine → withdrawn - 2019: development of DS-Cav1, a pre-fusion RSV candidate vaccine eliciting high levels of high-quality neutralising antibodies - Recently RSV vaccines for use in older adults (2) and pregnant women (1) have been licensed Crank et al. Science 2019 SARS-CoV-2 vaccines are also based on the prefusion conformation of the spike protein Whatisbiotechnology.org