2024 Cell#8 RNA Processing Notes PDF
Document Details
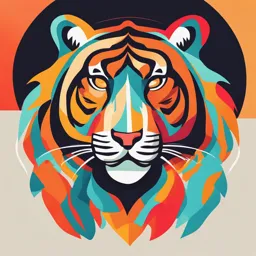
Uploaded by WellEducatedWichita
Burman University
Tags
Summary
This document provides notes on post-transcriptional gene control, specifically covering topics like RNA processing, splicing, and the regulation of mRNA molecules, with references to Lodish et al., 2021, Chapter 9.
Full Transcript
Burman University, BIOL 374, Post-Transcriptional Gene Control, Lodish et al. (2021), Chapter 9, Page 1 Post-Transcriptional Gene Control Learning Objectives: 1. Describe the posttranscriptional processing of eukaryotic pre-mRNA to form mRNA, specifically, 5’-capping, 3’ cleavage and po...
Burman University, BIOL 374, Post-Transcriptional Gene Control, Lodish et al. (2021), Chapter 9, Page 1 Post-Transcriptional Gene Control Learning Objectives: 1. Describe the posttranscriptional processing of eukaryotic pre-mRNA to form mRNA, specifically, 5’-capping, 3’ cleavage and polyadenylation and RNA splicing to remove introns. 2. Discuss the regulation of post-transcriptional processing to create alternately spliced mRNA, and the role of splicing factors. 3. Describe the mechanisms of molecule transport through the nuclear pore complex. 4. Discuss the role of cytoplasmic proteins/pathways to exert post-transcriptional control of mRNA. 5. Define the following terms: 5’ cap, alternate splicing, cleavage/polyadenylation complex, cross-exon recognition complex, FG-nucleoporins, nuclear pore complex, miRNA, mRNA surveillance, mRNP exporter, poly(A) tail, hnRNP, pre-mRNA, RNA editing, siRNA, RNA splicing, snRNA, spliceosome, SR proteins and others. Text: Lodish et al. (2021), Ch. 9 & S13.6 Verse: If the axe is dull and its edge unsharpened, more strength is needed, but skill will bring success. Ecc 10:10 (NIV) At times you may wonder, or feel, that all your hard work in this class is not paying off in better grades – that you’re investing so much in but not seeing a lot come out! Then it must be time to “sharpen your axe” and try a new way to study for this class. What got you through first and second years may not be sufficient, necessitating the need to add more effective study habits ("skills") to your current tool kit. Let’s have a chat if that is the case. Introduction ~60% of human genes have alternatively spliced mRNA. Chapter 9 Outline 9.1 Processing of Eukaryotic Pre-mRNA 9.2 Regulation of Pre-mRNA Processing 9.3 Transport (of mRNA) Across the Nuclear Envelope (Ch. 13.6) 9.4 Cytoplasmic Mechanisms of Post-transcriptional Control LW2024 Burman University, BIOL 374, Post-Transcriptional Gene Control, Lodish et al. (2021), Chapter 9, Page 2 9.1 Processing of Eukaryotic Pre- mRNA § Processing of eukaryotic pre-mRNA, or primary mRNA transcript, occurs in the nucleus [F9-2], involves: 1. 5’-capping 2. 3’ cleavage and polyadenylation 3. RNA splicing to remove introns § Processed RNA is transported into cytoplasm where mRNA is translated by ribosomes. A. 5’-cap – After initiation by RNA polymerase II, the 5¢-cap is added to nascent RNAs, after ~25 n’tides long. – Made up of a 7-methylGMP and methylated 2’-position of ribose in the 1st and 2nd nucleotides [F4-14, old text]. – 5’-cap marks RNA molecules as mRNA. – A capping enzyme associated with phosphorylated CTD of RNA pol II so that only mRNA is capped (mRNA is only ~10% of total RNA). Removes the g-phosphate from the 5’ end of nascent mRNA [F9-3]. Transfers a GMP moiety from GTP to the 5’ diphosphate of the mRNA. Unusual 5’-5’ triphosphate bond is formed. Separate enzymes transfer CH3 group from S- adenosylmethionine to N7 position of guanine and the 2’-OH of ribose. B. Pre-mRNAs are associated with hnRNP proteins. – hnRNPs, or heterogeneous ribonucleoprotein particles, are abundant RNA-binding proteins. – hnRNPs contain hnRNA, or heterogeneous nuclear RNA. – hnRNA is a collective term referring to pre-mRNA and other nuclear RNAs of various sizes. – hnRNP proteins assist in processing and transport of mRNAs. – Association of pre-mRNA with hnRNPs prevent the formation of short complementary intramolecular secondary structures. – Hybridization of splice factors-RNA molecules is accelerated by hnRNP. – While some hnRNPs are localized in the nucleus, others can cycle in and out of the cytoplasm to function as mRNA transporters. LW2024 Burman University, BIOL 374, Post-Transcriptional Gene Control, Lodish et al. (2021), Chapter 9, Page 3 – hnRNPs, like transcription factors, contain one or more conserved RNA-binding domains and at least one other domain that interacts with other proteins. – RNA-binding motifs contain amino acid sequences with positively-charged side chains that bind to the negatively-charged backbone of hnRNA. – e.g., RNA recognition motif (RRM), also called the RNP motif, is the most common RNA-binding domain in hnRNP proteins. This ~80 amino-acid motif is characterized by two a helices and four b strands, two of the later contain conserved sequences, RNP1 and RNP2, that lie side-by-side. The ssRNA lie across the surface of the b sheet containing RNP1 and RNP2 [F9- 6]. C. 3’ Cleavage and Polyadenylation – In eukaryotic cells, all mRNAs, except histone mRNAs, have a 3’ poly(A) tail. – 3’ Cleavage of pre-mRNAs, which occurs first, is followed by polyadenylation. – These processes are tightly coupled by poly(A) polymerase or PAP, which cleaves pre-mRNA at the poly (A) site and adds the poly (A) tail. – Poly(A) site is where cleavage and polyadenylation occur [F9-15]. Nearly all mRNA contains a conserved polyadenylation signal (AAUAAA), 10-35 n’tides upstream from a poly(A) site. A GU- or U-rich sequence, ~50 n’tides downstream from the poly(A) site, contributes to the efficiency of cleavage/polyadenylation. – At the PAP complex: Cleavage and polyadenylation specificity factor (CPSF) binds the AAUAAA polyadenylation signal to form an unstable complex. Cleavage stimulatory factor (CStF) binds the G/U- site and CPSF, forming a loop. Cleavage factors (CF), CFI and CFII, help stabilize the CPSF-CStF-pre-mRNA complex. Poly(A) polymerase (PAP) cleaves poly(A) site. PAP adds ~ 12 A residues slowly at first. The binding of nuclear poly(A) binding protein (PABPN1) accelerates the rate of A addition and signals termination when 200-250 As are added. Binding of PABPN1 is also essential for the export of the mRNA into the LW2024 Burman University, BIOL 374, Post-Transcriptional Gene Control, Lodish et al. (2021), Chapter 9, Page 4 cytoplasm. PABPN1 is exchanged for PABPC1 after mRNA is transported into the cytoplasm (PABPC1 resides in the cytoplasm and is the same PABPC involved in the polysomes). D. RNA Splicing – RNA splicing follows cleavage and polyadenylation for shorter transcriptional units. However, for longer transcripts, one with many exons, splicing of exons begins before transcription is complete. – RNA splicing is carried out by a large ribonucleoprotein complex called the spliceosome. The spliceosome is assembled by interactions of 5 different snRNP particles with each other and with pre-mRNA. – The spliceosome catalyzes two transesterification reactions that join the exons and remove the intron as a lariat structure, which is subsequently degraded. – Splicing occurs at short, conserved sequences [F9-7], called splice sites around intron-exon junctions in pre-mRNA. Only ~ 30-40 n’tides at each end of the intron are necessary. 5’-GU and 3’-AG (or GU-AG sequences; a pyrimidine-rich region is also important in higher animals) Branch point A – Splicing proceeds via two sequential transesterfication reactions [F9-8]: During transesterification, one phosphate-ester bond is exchanged for another. No energy is consumed because the number of phosphate-ester bonds are maintained. In the lariat structure (lasso) the 5’G of the intron is joined to the branch point A by an unusual 2’,5’- phosphodiester bond. – Small nuclear RNAs (snRNAs) Five snRNAs, designated as U1, U2, U4, U5 and U6, assist in pre-mRNA splicing. These ~100-200 n’tide snRNAs are associated with 6-10 proteins in small nuclear ribonucleoprotein particles (snRNPs). During splicing, snRNA base-pair with pre- mRNA and with one another. Rearrangements in the RNA-RNA interactions are critical in the splicing pathway [F9-9a]. § U1 snRNA base pairs to the 5’-splice site. LW2024 Burman University, BIOL 374, Post-Transcriptional Gene Control, Lodish et al. (2021), Chapter 9, Page 5 § U2 snRNA binds the branch point A. – The spliceosome is a ribonucleoprotein complex composed of multiple snRNPs and pre-mRNA. Spliceosome-mediated splicing [F9- 13]: Base-pairing of U1 snRNA (of U1 snRNP) to the 5’-GU splice site. SF1 (splicing factor 1) binds to branch point A. U2AF (U2 associated factor) associates with pyrimidine tract and 3’-AG splice site. U2 snRNP associates at branch point via base-pairing interactions, displacing SF1. U4/U5/U6 complex joins the preformed U1/U2/pre-mRNA complex to form the spliceosome. Extensive rearrangements in the base pairing of the snRNAs and the pre-mRNA lead to the release of U1 and U4. Catalytic core of U6 and U2 catalyze 1st transesterification that forms the 2’,5’- phosphodiester bond between the branch point A and the phosphate at the 5’splice site. Rearrangement followed by 2nd transesterification ligates the exons, and releases the lariat. Intron-snRNP complex rapidly dissociates, and the excised intron is rapidly degraded by a debranching enzyme and nuclear RNAses (called exosomes). – Exosomes degrade excised introns Nuclear exosomes are multiprotein complexes that contain eleven 3’à5’ exonucleases as well as RNA helicases. Exonucleases hydrolyze one base at a time from either the 5’ or 3’ end of an RNA molecule. RNA helicases disrupt base pairing and RNA-protein interactions that impede the exonucleases. Exosomes also degrade improperly processed pre-mRNAs. And also, antisense RNA. § RNA transcribed in the “wrong” direction contain polyadenylation signals (PAS) that lack U1 binding sites (left side of F10-17; Old text). § Antisense transcripts are cleaved to generate free RNA ends that are digested by nuclear exosomes and the 5’à3’-exonuclease. LW2024 Burman University, BIOL 374, Post-Transcriptional Gene Control, Lodish et al. (2021), Chapter 9, Page 6 § In contrast, pre-mRNA transcribed in the coding regions have few polyadenylation signals. Which when they occur usually is preceded by a binding site for UI snRNP, which inhibits cleavage at a nearby PAS [right side of F10-17; Old text]. § However, PAS at the 3’-end of a coding mRNA does not have a UI RNP binding site, hence can be cleaved there. – AU-AC introns A small % (~1% in humans) of pre-mRNA have introns that begin with AU and ending with AC (instead of the usual “GU-AG” rule). These introns are removed via a splicing cycle that involves four novel snRNPs in addition to U5 snRNP. – Cross-exon recognition complex Since the 5’/3’ splice sites are degenerate and multiple copies of the splice sites occur randomly in long introns, additional sequence information is required to define the exon-intron boundaries. In large pre-mRNAs of higher eukaryotes, SR proteins bind to a sequence in the exon, called the exonic splicing enhancer (ESE) sequence, critical for correct exon definition. A network of interactions between SR proteins, snRNPs and splicing factors forms a cross-exon recognition complex (i.e., spans an exon) that specifies correct splicing sites [F9-16]. SR proteins mediate the cooperative binding of U1 snRNP to a true 5’splice site and U2 snRNP to the branch point. ~15% of single-base-pair mutations that causes human genetic diseases interfere with proper exon definition. Other proteins associated with the exon boundaries. § Following splicing, some hnRNPs remain bound to the exon-exon junction, forming the exon-junction complex. One of such is an RNA export factor (REF) which functions in the export of fully processed hnRNPs from the nucleus to the cytoplasm. § Some exon junction proteins function to degrade improperly spliced mRNAs in the nonsense-mediated decay pathway. LW2024 Burman University, BIOL 374, Post-Transcriptional Gene Control, Lodish et al. (2021), Chapter 9, Page 7 E. CTD – The long CTD domain of RNA Pol II allows multiple proteins to associate simultaneously with a single RNA Pol II molecule [F9-4]. – e.g., 5’-capping enzyme is associated with phosphorylated CTD shortly after transcription initiation. – RNA splicing and polyadenylation factors are also associated with phosphorylated CTD. – These processing factors are at high local concentrations when splice sites and poly(A) signals are transcribed by RNA Pol II, thus enhancing the rate and specificity of RNA processing. – These interactions also stimulate transcription elongation, possibly to ensure that pre-mRNA synthesis only occurs unless the machinery for processing is properly in place. 9.2 Regulation of Pre-mRNA Processing § Several forms of regulation include: A. Alternative splicing of pre-mRNA. – Alternative splicing of different exons (including those with alternate poly(A) sites). B. Regulation of RNA splicing by RNA-binding proteins. – Splicing repressors and activators control splicing at alternative splice sites. C. RNA editing. – The sequence of the pre-mRNA is altered so that the mature mRNA differs from the genomic DNA. A. Alternative Splicing – Alternative splicing of the same gene leading to different mRNAs expressed in different cell types. – Different mRNAs may also be expressed at different developmental stages. – Involve internal exons, 5’ or 3’-exons of complex transcriptional units [F7-3b]. – Alternative splicing of exons is especially common in the nervous system, generating multiple protein isoforms required for neuronal development and function in both vertebrates and invertebrates. – The Dscam Gene of Drosophila retinal neurons can produce 38,016 different mRNAs by alternative splicing of 95 exons [F9-20]. – e.g., alternative splicing of slo mRNA, which encodes a Ca2+-gated K+ channel, in LW2024 Burman University, BIOL 374, Post-Transcriptional Gene Control, Lodish et al. (2021), Chapter 9, Page 8 auditory hair cells [F9-17]. Individual “hair cells”, or ciliated neurons that respond strongly to a specific frequency of sound. Cells “tuned” to low 50 Hz frequency are found at one end of the tubular cochlea of chickens, those tuned to high frequency (5000 Hz) at the other end, and cells in between responding to a gradient of frequencies between the extremes. The tuning of these auditory neurons occurs via the opening of K+ ion channels in response to increased Ca2+ concentrations. The Ca2+ concentrations at which the channel opens determines the frequency to which the cell is tuned. Alternative splicing of slo mRNA, which encodes a Ca2+-gated K+ channel in auditory hair cells, contributes to the perception of sounds at different frequencies. The Slo protein contains 7 transmembrane a helices (S0-S6), which associate to form the K+ channel. The cytosolic domain, encoded by 4 hydrophobic regions (S7-S10), regulates opening of the channel. Isoforms of Slo open at different Ca2+ concentrations and thus to different frequencies. Eight regions (à) in the mRNA where alternative exons are utilized permit the expression of 576 possible Slo isoforms. B. Splicing Repressors & Activators – Regulation of alternate splicing by RNA-binding proteins that bind to specific sequences near regulated splice sites. – Splicing inhibitors block access of splicing factors to splice sites, causing exons to be skipped [F9-18a, b; Sxl]. – Splicing activators may enhance splicing by interacting with splicing factors, thus promoting their association with the regulated splice site [F9-18c; Tra-Tra2]. – A cascade of regulated RNA splicing controls Drosophila sexual differentiation [F9-18]. The sex-lethal (sxl) gene is only produced in females. Sxl is a splicing inhibitor that prevents splicing of exon #2 in the transformer (tra) gene of females. Tra is a splicing activator that promotes splicing of exons #3 and 4 of the double-sex (dsx) gene in females. Tra works in conjuction to SR (or splicing regulator) proteins Rbp1 and Tra2 [F9-19]. LW2024 Burman University, BIOL 374, Post-Transcriptional Gene Control, Lodish et al. (2021), Chapter 9, Page 9 Exons 2 and 4 of Sxl contain RNA binding domains. In the presence of Tra, the dsx mRNA includes exon 4 in females. In the absence of Tra, exon 4 is skipped and exons 3 and 5 are joined in male dsx mRNA. The male Dsx protein is a transcriptional repressor that inhibits the expression of genes required for female development. Conversely, the female Dsx protein represses transcription of “male” genes. C. RNA editing – In RNA editing the nucleotide sequence of a pre-mRNA is altered in the nucleus. – In vertebrates, this process is fairly rare and entails changes at one nucleotide (or codon). – Widespread in the mitochondria of protozoans and plants (also chloroplasts). – Involve insertions and deletions of U residues using guide RNAs (gRNAs). The 5’end of gDNA complementary base-pairs with a section of the unedited pre- mRNA, hence serving as an anchor, while the 3’-end serves as a template for editing. Editing in regions 1, 2, 3 and 5 above result in insertions in the edited pre-mRNA, while editing in region 4 results in a deletion [Fig above; old text]. – RNA editing alters the sequences of mammalian pre-mRNAs, e.g., cell-type-specific expression of the apo-B gene result in two forms of the serum apolipoprotein B (ApoB-48 and ApoB-100; F9-25). Both are components of the lipoprotein complexes that transport lipids in the serum. However, only ApoB-100 (in LDLs) delivers cholesterol to body tissues by binding to the LDL receptor present in all cells. RNA editing of the apo-B mRNA gene result in the two isoforms. RNA editing enzyme is a deaminase, converting the C6666 to a U (CAA codon for glutamine to a UAA stop codon). 9.3 Transport across the Nuclear Envelope § Fully processed mRNAs in the nucleus remain bound by hnRNP proteins in complexes now referred to as nuclear mRNPs. § Before mRNA is translated into protein, it must be exported out of the nucleus into the cytoplasm. LW2024 Burman University, BIOL 374, Post-Transcriptional Gene Control, Lodish et al. (2021), Chapter 9, Page 10 A. NPCs (see also section 13.6) – The nuclear envelope contains numerous nuclear pore complexes (NPCs), which are large (~60-80 million da), complicated structures composed of multiple copies of ~30 proteins called nucleoporins. – Water, ions, metabolites and small proteins (up to 40 kDa) diffuse freely through nuclear pores. – Nuclear pore complex structure [F13-32ab]. From the cytoplasmic side (top), it is a roughly octagonal, membrane embedded structure with cytoplasmic filaments extending into the cytosol. The nuclear side has eight ~100-nm long filaments extending into the nucleoplasm, the distal ends joined by the terminal ring, forming a structure called the nuclear basket (like a basketball hoop). Three types of nucleoporins make up the NPC – structural nucleoporins, membrane nucleoporins and FG-nucleoporins. 1. Membrane nucleoporins form annular structures that are located where the inner and outer leaflets of the nuclear membrane connect at the NPC. 2. Structural nucleoporins form the scaffold of the NPC, together with the membrane nucleoporin, form the eight-fold symmetric ring-like opening of the NPC. § Several structural nucleoporins form a large Y-shaped structure called the Y-complex. § 16 copies of the Y-complex form the basic structure of the pore (bilateral symmetry in the nuclear envelope and 8-fold symmetry in the plane of the envelope; F13-32c). 3. Lining the channel of the NPC are FG-nucleoporins, which contain multiple repeats of a short hydrophobic Phe-Gly sequence (FG-repeats) interspersed with hydrophilic regions. § The FG-repeats extend into the central channel, filling the pore of the NPC. § FG-repeats in the FG-nucleoporins associate with each other to form a mesh-work much like a gel-like molecular sieve (F13-33d). § These FG-nucleoporins play a role in the transport of all macromolecules LW2024 Burman University, BIOL 374, Post-Transcriptional Gene Control, Lodish et al. (2021), Chapter 9, Page 11 through nuclear pores. § Small molecules can diffuse through the “holes” of the sieve while larger ones are too large and consequently cannot diffuse through the nuclear pore. § Transport of macromolecules larger than ~40 kDa through nuclear pores requires the assistance of chaperone proteins that interact with both the transported molecule (“cargo”) and with the FG-repeats of FG-porins. The membrane-embedded portion of the NPC is directly attached to the nuclear lamina, a network of intermediate filaments extending over the inner surface of the nuclear envelope. B. Formation of mRNPs [F8-28a, b] – Nascent RNA transcripts associate with various proteins forming hnRNPs. – hnRNPs containing fully processed mRNAs are referred to as messenger RNPs (mRNPs). – e.g., coiled mRNPs of Chironomous tentans containing Balbiani ring mRNA. – Passage of mRNPs through nuclear pore complexes [F9-28c] After the coiled mRNP moves through the terminal ring, it uncoils as it passes through the NPC, with the 5’-end of the mRNA leading the way. Ribosomes rapidly associates with mRNA as it enters the cytoplasm. – mRNPs are exported from the nucleus with the aid of an mRNP exporter. The heterodimeric mRNP exporter consists of a large subunit, called nuclear export factor 1 (NXF1) and a smaller subunit, nuclear exporter transporter 1 (NXT1). NXF1 associates with nuclear mRNP via binding directly to the RNA or other proteins in the mRNP complex like REF (RNA export factor; component of the exon-junction complexes). NXF1/NXT1 mRNP exporter also bind to SR proteins in the cross-exon recognition complex (recall SR proteins are involved in mRNA splicing, and now also involved in NPC transport). NXF1/NXT1 mRNP exporter interact with the FG-domains of the FG- nucleoporin to facilitate export of the mRNPs through the NPC central channel. – mRNP remodeling Other proteins like Dbp5, an RNA helicase that utilizes ATP hydrolysis for dissociating RNA-protein complexes, are also bound to the transport complex. In a process called mRNP remodeling, the proteins associated an mRNA in the LW2024 Burman University, BIOL 374, Post-Transcriptional Gene Control, Lodish et al. (2021), Chapter 9, Page 12 nuclear mRNP complex are exchanged for a different set of proteins as the mRNP is transported through the NPC [F9-26; F13- 36b]. – mRNP export Some of the proteins associated with the mRNP are dissociated from the mRNP before export through the NPC, others are transported back into the nucleus (e.g., PABPN1 and CBC). In the cytoplasm, translation initiation factor eIF4E replaces CBC (cap-binding complex) and PABPC1 (cytoplasmic poly(A)-binding protein) replaces PABPN1 (nuclear poly(A)-binding protein). Pre-mRNAs bound by a spliceosome normally are not exported from the nucleus, assuring that only fully processed, functional mRNAs reach the cytoplasm for translation. – Phosphorylation/dePhosphorylation Phosphorylation and dephosphorylation of SR proteins imposes directionality on mRNP export across the NPC. Phosphorylated SR protein Npl3 binds new pre-mRNAs, but when successfully polyadenylation occurs, the Glc7 nuclear phosphatase dephosphorylates Npl3. Dephosphorylated Npl3 binds to NXF1/NXT1, the mRNP exporter. mRNP complex passes through the NPC. In the cytoplasm, protein kinase Sky1 phosphorylates Npl3, causing the dissociation of the mRNP complex. Npl3 and mRNA transporter (NXF1/NXT1) are transported back into the nucleus (F9-27). C. Nuclear Export and Import [S13.6] – In both nuclear export and import, the protein to be transported (“cargo” protein) contains a specific amino acid sequence that functions as a nuclear-export signal (NES), or a nuclear-localization signal (NLS). – Nucleus-restricted proteins contain an NLS but not a NES, whereas proteins that shuttle between the nucleus and cytoplasm contain both signals. LW2024 Burman University, BIOL 374, Post-Transcriptional Gene Control, Lodish et al. (2021), Chapter 9, Page 13 – Proteins with a nuclear-localization signal (NLS) are recognized by receptors and transported into the nucleus [F13-33]. – NES/NLS Several different types of NES and NLS have been identified. Each class of nuclear-transport signal is thought to interact with a specific receptor protein (exportin or importin) belonging to a family of homologous proteins termed karyopharins. A “cargo” protein bearing NES or NLS translocates through nuclear pores bound to its cognate receptor (karyopharins), which also interacts with FG- nucleoporins. – FG-repeats and Ran Importins and exportins are thought to diffuse through the channel by binding transiently to different FG-repeats that act like “stepping stones” through the pore. Both nuclear export and import involve Ran, a monomeric G protein that can exist in different conformations when bound to GTP or GDP. – Cargo Complex Upon reaching its destination (the cytoplasm during export and the nucleus during import), the cargo complex dissociates, freeing the cargo protein and other transport components. The later are recycled, by being transported back through the nuclear pores in the reverse direction, to participate in transporting additional molecules of cargo protein (see F13-35 and F13-36a). – GEF and GAP The unidirectional nature of protein export and import through nuclear pores result from the localization of Ran guanine-nucleotide exchange factor (GEF) in the nucleus and of Ran GTPase-accelerating protein (GAP) in the cytoplasm. The interaction of import cargo complex with Ran-GEF in the nucleus causes the dissociation of the complex, releasing the cargo into the nucleoplasm. GEF exchanges the Ran-bound GDP for a GTP. Export cargo complexes dissociate in the cytoplasm when they interact with Ran GAP localized to the NPC cytoplasmic filaments. Ran GAP activates the Ran GTPase activity to convert Ran-bound GTP to GDP. – Nuclear import of cargo proteins [F13-35] and nuclear export of cargo proteins [F13-36a] LW2024 Burman University, BIOL 374, Post-Transcriptional Gene Control, Lodish et al. (2021), Chapter 9, Page 14 – Most mRNAs are exported from the nucleus by a Ran-independent mechanism (see previous S8.3b). 9.4 Cytoplasmic mechanisms of post-transcriptional control A. Regulation of translation by repressing translation. 1. Micro RNAs repress translation of specific mRNAs. – LIN-14 transcription factor is important in the development of early larval organs in Caenorhabditis elegans. – lin-14 mRNA is regulated by lin-4 RNA, a small 21- nucleotide antisense RNAs called “micro-RNAs” (or miRNA), that is complementary to an imperfect repeat in the lin-14 3’UTR (fig old text). – Binding of lin-4 RNAs to lin-14 3’UTR prevents lin-14 mRNA translation. – miRNAs are made from precursor RNAs that are processed by the enzyme Dicer (which cleaves dsRNA as in the RNAi technique). § RNA interference – The related phenomenon of RNA interference (RNAi), which probably is a defense system against viruses and transposons, degrades mRNAs that form LW2024 Burman University, BIOL 374, Post-Transcriptional Gene Control, Lodish et al. (2021), Chapter 9, Page 15 perfect hybrids with short interfering RNAs (siRNAs). – Both miRNAs and siRNAs contain 21-23 n’tides, are generated from longer precursor molecules (DICER), and are assembled into multiprotein RNA- induced silencing complex (RISC) that either repress translation of target mRNAs or cleaves them. – Base pairing with target RNA distinguishes miRNA and siRNA. Perfect hybrids lead to RNA destruction [F9-31b]. § Repression by miRNA – Translation can be repressed by miRNAs which form imperfect hybrids with sequences in the 3’-UTR of specific targets [F9-31a]. – miRNA regulation is widespread in all multicellular plants and animals. – miRNAs are processed from genes encoding pre-miRNAs [F9-32], or from gene sequences derived from excised introns and from 3’-UTRs of some pre- mRNAs. 2. Translation-control complexes at 3’-UTRs § Sequence-specific translation-control proteins may bind cooperatively to neighboring sites in 3’UTRs and function in a combinatorial manner (much like transcription factors on promoter elements in transcription initiation). § In most cases, translation of the mRNA is repressed by these structures, e.g., selective inhibition of mRNA translation in the Xenopus Oocyte – In the immature oocytes, some mRNAs are selectively inhibited (or “stored”) from translation until after fertilization by the sperm. – These mRNAs have short poly(A) tails with a U-rich region (CPE), which form the binding site for the binding protein CPEB (F9-34). – Maskin tether the 5’ and 3’ ends of mRNA together by binding to CPEB (at the 3’ end) and eIF4E (at the 5’ cap) of the mRNA, forming the translation dormant complex, hence, blocking translation initiation. – Translation initiation requires the binding of eIF4E to eIF4G, a translation initiation factor that recruits the small ribosomal subunit to the mRNA. – Upon stimulation by progesterone during ovulation, CPEB is phosphorylated LW2024 Burman University, BIOL 374, Post-Transcriptional Gene Control, Lodish et al. (2021), Chapter 9, Page 16 and can bind CPSF, which recruits poly(A) polymerase to the 3’ end. This results in the growth of the poly(A) tail. – PABPC1 can bind to the elongated poly(A) tail and eIF4G, forming a circular polysome (where multiple ribosomes can translate the message). – Polysomes are translation-competent complexes (F5-39b). B. Regulating mRNA stability by modulating degradation rate. § mRNAs are degraded by several mechanisms in the cytoplasm. § Most mRNAs are degraded as the result of the gradual shortening of their poly(A) tail. § In the deadenylation-dependent pathway, the poly(A) tail is progressively shortened by a deadenylase until it reaches a length of 20 or fewer residues. – At this point, the interaction with PABPC1 is destabilized, leading to weakened interactions between the 5’cap and translation initiation factors (hence polysome disintegrates). – The deadenylated mRNA then may either (1) be decapped (decaping enzyme DCP1/DCP2) and degraded by a 5’à3’ exoribonuclease (XRN1) or (2) be degraded by a 3’à5’ exonuclease in cytoplasmic exosomes (F9-30a). § In the deadenylation independent pathway, some mRNAs are decapped before they are deadenylated, and then degraded by a 5’à3’ exonuclease [F9-30b, bottom left]. § Other mRNAs are cleaved internally by an endonuclease, and the fragments degraded by an exosome (endonucleolytic pathway, F9-30c, bottom right). § Short-lived mRNAs – Eukaryotic mRNAs encoding proteins that are expressed in short bursts generally have repeated copies of an AU-rich sequence in their 3’UTR that bind specific proteins. – These proteins also interact with the deadenylation enzyme and cytoplasmic LW2024 Burman University, BIOL 374, Post-Transcriptional Gene Control, Lodish et al. (2021), Chapter 9, Page 17 exosomes, promoting rapid RNA degradation. – By removing/degrading mRNAs, one could shut down the translation of these mRNAs. – These RNA-binding proteins bind to regulatory elements in the 3’ or 5’ UTRs of many mRNAs to regulate their translation and degradation in the cytoplasm. § Translation of ferritin mRNA and degradation of transferrin receptor (TfR) mRNA are both regulated by the same iron-sensitive RNA-binding protein (F9-35). – Ferritin is an intracellular iron- binding protein that prevents the accumulation of toxic levels of free Fe. Ferritin mRNA translation is controlled by iron-response element-binding proteins (or IRE-BP) which bind to the iron response elements (or IRE) in the 5’UTR of ferritin mRNA. At low [iron], this protein is in a conformation that binds to the IREs, inhibiting ferritin translation [F9-35a]. – Transferrin-receptor (TfR) is a plasma receptor is responsible for the import of Fe (transported as transferrin) into the cell (by receptor- mediated endocytosis). Low [iron] causes IRE-BP to bind to iron response elements (IREs) at the 3’UTRs of TfR mRNA, preventing degradation. At high [iron], the exposed IRE, which contain AU-rich degradation signals, promote the degradation of TfR mRNA by the same mechanism that leads to rapid degradation of short-lived mRNA [F9-35b]. – The dual control by IRE-BP precisely regulates the level of free Fe ions within cells. § mRNA surveillance mechanisms – Several mechanisms, collectively termed mRNA surveillance, help cells avoid the translation of improperly processed mRNA molecules: 1. One of such is the prevention of nuclear export of incompletely spliced pre- mRNAs (that remain associated with a spliceosome). These sequences are eventually degraded by exosomes (3’à 5’ hydrolysis by nuclear exonucleases). 2. Nonsense-mediated decay causes degradation of mRNAs in which one or more exons are skipped during splicing. LW2024 Burman University, BIOL 374, Post-Transcriptional Gene Control, Lodish et al. (2021), Chapter 9, Page 18 Exon skipping could result in out-of-frame missense mutations and an incorrect stop codon (premature stop codons are often seen in other reading frames). The presence of a stop codon prior to the last splice junction results in rapid decay via nonsense-mediated decay. Exon-junctional complexes play a role in this mechanism. 3. Non-stop decay (F9-37b) degrades mRNA that do not possess a stop codon and prematurely poly adenylated. Ribosome continues translation to the end of the poly(A) tail where it is arrested and still tightly bound to the mRNA. (Typically, ribosomes are released at stop codons by release factors; F5-38). These still tightly bound ribosomal-mRNA structures are recognized by Ski7 protein (that binds to the empty A site of the ribosome), which then recruit the exosome to deadenylate and rapidly decay the transcript in the 3’à 5’ direction. In the absence of Ski7, the loss of PABPC1 (which associates with the poly(A) tail normally) leads to the decapping and 5’à 3’ degradation by XRN1. 4. No-go decay (F9-37c) degrades damages to the mRNA template or the presence of an unusually large and stable secondary structure in the mRNA upstream of the stop codon. This leads to the blocking of ribosome translocation along the mRNA causing ribosomes to stack-up. Leading to endonucleolytic cleavage of the mRNA and participation of exosomes (3’à5’ cleavage) and XRN1 (5’à 3’ cleavage) at the resulting cleavage site. C. Regulating mRNA localization by modulating protein localization. § Some mRNAs are directed to specific subcellular locations by sequences usually LW2024 Burman University, BIOL 374, Post-Transcriptional Gene Control, Lodish et al. (2021), Chapter 9, Page 19 found in the 3’UTR. § Localization of mRNAs permit production of proteins at specific regions within the cytoplasm. § e.g., localization Ash1 mRNA to daughter cell of budding yeast result in no HO gene transcription and hence, no switching of mating type. – Whether a haploid yeast cell exhibit the a or α mating type is determined by whether a or α genes are present at the MAT locus on chromosome III [F8-31]. – The transfer of a or α genes from the silent mating locus (closed to the repressed telomeres) is initiated by an endonuclease called HO. – Only the mother cell (M) can switch mating type to produce two cells of the opposite type [F9-38]. – Ash1 mRNA, which produces a transcriptional repressor (Ash1) of the HO gene, is accumulated only in the daughter cells by a localization process that include the transport of Ash1 mRNA from the mother cell to the daughter cell. – RNA-binding proteins bind to localization signals found in the 3’-UTR of Ash1 mRNA. – These proteins associate with motor proteins to carry mRNAs on actin or microtubule fibers from M to D cells [F9-39]. LW2024