Gene Expression & Regulation - Part 2 PDF (August 19, 2024)
Document Details
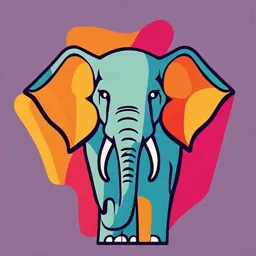
Uploaded by GratefulHyperbolic
University of Arizona
2024
Tags
Summary
These notes cover gene expression and regulation, focusing on mRNA splicing, translation regulation, nonsense-mediated decay, and microRNAs. The document includes learning objectives, instructions, and practice exam questions for a course at the University of Arizona.
Full Transcript
GENE EXPRESSION & REGULATION – PART 2 Block: Foundations Block Director: James Proffitt, PhD Session Date: Monday, August 19, 2024 Time: 2:00 - 3:00 PM Instructor: David Bear, PhD Department: Cellular & Molecular Medicine Email: Pl...
GENE EXPRESSION & REGULATION – PART 2 Block: Foundations Block Director: James Proffitt, PhD Session Date: Monday, August 19, 2024 Time: 2:00 - 3:00 PM Instructor: David Bear, PhD Department: Cellular & Molecular Medicine Email: Please contact Dr. Romanoski with questions – [email protected] INSTRUCTIONAL METHODS Primary Method: IM10: Independent Learning ☐ Flipped Session ☐ Clinical Correlation Resource Types: RE18: Written or Visual Media (or Digital Equivalent) INSTRUCTIONS/READINGS Please read the LEARNING OBJECTIVES and NOTES prior to initiating the ILM video. Review the cases and answer the PRACTICE EXAM QUESTIONS located at the end of NOTES. LEARNING OBJECTIVES 1. Describe how alternative mRNA splicing regulates gene expression and how aberrant mRNA splicing can lead to disease 2. Outline how the translation of ferritin mRNA is regulated by the iron response element binding protein 3. Discuss the role of nonsense-mediated decay in mRNA quality control 4. Explain how the mTOR pathway globally controls translation during cell growth and in cancer cells 5. Diagram the pathway for the synthesis of miRNAs and discuss how miRNAs regulate the translation and stability of specific mRNAs. 6. Explain how an mRNA COVID-19 vaccine works 7. Discuss the how the frameshift mutations and mutations in introns can cause Duchenne muscular dystrophy CURRICULAR CONNECTIONS Related Related Competency\EPO Disciplines Threads COs LOs CO-01 LO-01 MK-01: Core of basic Molecular H & I: Medical Genetics sciences Biology CO-01 LO-02 MK-01: Core of basic Molecular H & I: Medical Genetics sciences Biology CO-01 LO-03 MK-01: Core of basic Molecular H & I: Medical Genetics sciences Biology CO-01 LO-04 MK-01: Core of basic Molecular H & I: Medical Genetics sciences Biology Block: Foundations | BEAR [1 of 16] GENE EXPRESSION & REGULATION – PART 2 Related Related Competency\EPO Disciplines Threads COs LOs CO-01 LO-05 MK-01: Core of basic Oncology H & I: Medical Genetics sciences CO-01 LO-06 MK-06: The foundations of Genetics H & I: Medical Genetics therapeutic intervention, including concepts of outcomes, treatments, and prevention, and their relationships to specific disease processes CO-01 LO-07 MK-05: The altered Genetics H & I: Medical Genetics structure and function (pathology & pathophysiology) of the body/organs in disease USMLE 1 STUDY CONNECTIONS FIRST AID 2023: p. 39-44 AMBOSS: (1) Post-Transcriptional Modification (2) Translational Regulation https://next.amboss.com/us/article/po0L1S?q=rna%20processing#Z2089ca9ea64aa218 93be3cbe134a8a1b https://next.amboss.com/us/article/Jo0s1S?q=translation#hoccbW0 NOTES CONTENTS Clinical Cases - Introduction Mechanisms of mRNA Splicing Regulation of mRNA Splicing Translation Initiation in Humans Effects of Point Mutations and INDELs on Translation Regulation of Translation Initiation Regulation of Translation & mRNA Decay by MicroRNAs Regulation of mRNA Turnover Discussion of Clinical Cases Practice Exam Questions CLINICAL CONTEXT Many inherited disease-associated gene mutations cause disease not because of protein dysfunction but because of defective gene regulation. We previously considered those mutations that affect transcription initiation and elongation. In this topic, we will focus on how gene expression is regulated at the steps of mRNA processing, mRNA decay and translation Block: Foundations | BEAR [2 of 16] GENE EXPRESSION & REGULATION – PART 2 initiation. Dysregulation of these processes leads to several important diseases that will be discussed. CLINICAL CASES Case 1. Design of the COVID19 mRNA Vaccine One of your fellow students is skeptical about receiving the mRNA COVID-19 vaccine; he does not understand the rationale of the vaccine composition. You sit down with him and provide an explanation of the mRNA vaccine components and how they work. Case 2. A Child with a Muscle Disorder A four-year-old male child is brought to the pediatrics clinic by his mother. The pre-school teacher reports that the child is unable to keep up with the other kids, tends to fall a lot and seems to have trouble negotiating the playground equipment. In retrospect, the patient’s mother has noticed that he won’t sleep in the top of his bunk bed anymore; and is not as physically active as his older sister. The patient had a normal gestational period and birth, is up-to date on all immunizations, has had no significant childhood illnesses, has met all the normal physical milestones (rolled over, sat up, walked), and is of normal height and weight. The mother is 30 years old and has two siblings (male and female); both are healthy. The father is 31 years old and has two siblings (male and female); both are healthy. Both sets of grandparents are alive and well. The mother’s uncle, who lived in Europe all his life, was confined to a wheelchair at an early age, and died in his teens before the family immigrated to this country. Physical exam reveals that the patient’s calf muscles are enlarged. He has difficulty rising from a lying or sitting position, has a waddling gait, and walks more on his toes than on the balls of his feet. MECHANISMS OF mRNA SPLICING All mammalian messenger RNAs (mRNA) are transcribed as precursors referred to as pre- mRNAs and processed to become mature mRNAs. Messenger RNA processing consists of three steps: (1) capping, the addition of the 7-methylguanosine cap onto the 5’ end of the mRNA; (2) splicing, removal of the introns and ligation of the exons; (3) polyadenylation, cleavage of the transcript 10-30 nt downstream of the polyadenylation signal and polymerization of a poly(A) tail containing 200-300 adenosine monophosphates. mRNA processing usually occurs concomitantly with transcription and is said to be transcriptionally coupled. The cleavage of the pre-mRNA occurs at specific sites called splice junctions. In the first step of RNA splicing, the 5-splice junction of the intron is cleaved at a site that usually has the sequence AG/GU. Next, the GU of the 5 end of the intron forms a covalent bond with an internal A residue at a site within the intron called the branch site, which has a loosely conserved sequence with a strategically located A in the center, which forms the covalent adduct to the 5’ end of the intron, generating a looped structure referred to as a lariat. In the last step of splicing, the 3-end of the intron, termed the 3’ splice junction, usually at an AG/G sequence, is cleaved between the two G residues, and the exons are used together. The mRNA splicing reaction is mediated by a set of ribonucleoprotein complexes called snRNPs Block: Foundations | BEAR [3 of 16] GENE EXPRESSION & REGULATION – PART 2 (pronounced “snurps.”). The snRNPs are made up of several small RNAs and associated proteins. In autoimmune diseases such as lupus, the body often makes large amounts of antibodies to the snRNPs. Figure 1. The steps of messenger RNA (mRNA) processing. Source: Wikimedia Commons. Figure 2. The mRNA splicing reaction occurs by a mechanism including two transesterification reactions: a nucleophilic attack of the branch point 2’ OH group on the branch point adenosine on the phosphate at the 5’ splice junction, and nucleophilic attack of the terminal OH group of base at the 3’ end of the exon on the phosphate at the 3’ splice junction. Source: D.G. Bear in Plopper et al. (2015) Lewin’s Cells 3rd Ed. REGULATION OF MESSENGER RNA SPLICING The cell utilizes several strategies to produce more than one transcript from a single gene. Eukaryotic genes can yield multiple protein products through a variety of processes including the following: (1) alternative use of RNA splice sites; (2) alternative use of polyadenylation sites; (3) alternative use of start and stop codons; (4) post-translational cleavage of a pro-peptide; and (5) frame shifting where ribosomes shift their reading frame in the middle of translating an mRNA. Block: Foundations | BEAR [4 of 16] GENE EXPRESSION & REGULATION – PART 2 Alternative splice site utilization is the most common mechanism for generating different protein isoforms. The figure below shows the variety of transcripts that can be generated by alternative splicing Figure 3. Examples of different alternative splice site utilization to generate different mRNAs. Source: Alberts et al. Molecular Biology of the Cell 6th Ed. 2015. Alternative RNA splicing is controlled by splice site activators and repressors. Splicing activators convert weak or cryptic splice sites into active splice sites, while splicing repressors occlude active splice sites, directing the splicing apparatus to the next active splice site. Figure 4. Regulation of mRNA splicing by activators and repressors Source: Alberts et al. Molecular Biology of the Cell 6th Ed. 2015. TRANSLATION INITIATION IN HUMANS The information in mRNA is encoded by base triplets called codons. Each codon specifies an individual amino acid. In most cases, there is more than one codon for each amino acid. Most (but not all) translation begins with an AUG codon, which encodes methionine, referred to as the start codon or initiator codon. The initiator codon resides within the context a short sequences on either side, together referred to as the translation initiation site. In eukaryotes, a set of initiation factors bind to the 5 and 3’ends of the mRNA linking the 7-methylguanosine cap with the poly(A) tail and guide the small ribosomal subunit (40S subunit) to attach to the 5’ end. Block: Foundations | BEAR [5 of 16] GENE EXPRESSION & REGULATION – PART 2 The 40S subunit then scans in the 5’ to 3’ direction along the mRNA looking for the first AUG sequence within a consensus sequence context. Most of the time in eukaryotes, the first AUG of the mRNA is the start codon. But in rare instances, the first AUG may be bypassed in favor of an AUG located in a more favorable sequence context. Figure 5. Initiation of translation in eukaryotes. Source: Wikimedia.commons. More recently, several mammalian translation initiation sites have been characterized where the ribosomes enter internally. These sites are called Internal Ribosome Entry Sites (IRES) and are found in several specific genes associated with cancer as well as in many of viral mRNAs. EFFECTS OF POINT MUTATIONS AND INSERTION-DELETION MUTATIONS (INDELS) ON TRANSLATION Point Mutations Point mutations (SNPs or SNVs) are single base substitutions that arise predominantly due to base modifying chemicals and ionizing radiation and DNA replication errors. If point mutations occur in the open-reading frame (ORF) of a protein-encoding gene, one of three results may occur. (1) If the mutation changes a codon, but due to the degeneracy of the genetic code, the amino acid corresponding to the codon is not changed, there is usually no effect. This is referred to as a synonymous mutation. (2) If the mutation results in a codon that codes for a different amino acid, it is referred to as a missense mutation. Missense mutations may or may not produce a phenotypic change in the protein. If the new amino acid is closely related in chemical properties to the amino acid normally encountered at that position in the protein (e.g., a glycine instead of an alanine, an arginine instead of a lysine, or glutamic acid instead of an aspartic acid), we call this a conservative substitution because the chemical properties (small aliphatic hydrocarbon, acid, base) are conserved. However, if the mutation results in a new amino acid with different chemical properties (non-conservative substitution), the function of the Block: Foundations | BEAR [6 of 16] GENE EXPRESSION & REGULATION – PART 2 resultant protein may be significantly affected. (3) If the mutation changes a codon into a stop codon (also referred to as a nonsense codon), the mutation is referred to as a nonsense mutation. There are three nonsense codons (UGA, UAA, and UAG), which can be easily remembered by a device: UGA (U Go Away), UAA (U Are Away), and UAG (U Are Gone). It is difficult to predict the effects of missense mutations because it depends on many factors including the chemical nature of the amino acid and its location within the protein sequence; however, a nonsense mutation is almost always going to lead to aberrant expression of the gene product. One would think that a truncated protein would be expressed due to the premature stop codon. However, such proteins can be extremely toxic to the cell, often competing with the wild-type protein generated from the heterozygous allele on a homologous chromosome. To minimize the generation of toxic truncated proteins due to nonsense mutations and out-of-reading frame changes that result in stop codons (see below), the cell has a mechanism for the recognition of nonsense codons in the exons of mRNAs that results in degradation of the mRNA before a toxic protein is translated; the process is called nonsense mediated decay (NMD). Thus, in most mutations that result in the generation of a nonsense codon, a truncated protein is not produced, although exceptions occur. Figure 9. Nonsense-mediated decay (NMD) is a quality control process, where transcripts containing a nonsense mutation or a premature stop codon caused by intron retention during splicing, are degraded. NMD prevents truncated proteins that would be generated by the nonsense (stop) codon. Truncated proteins are usually toxic to the cell. Source: Wikimedia Commons. Insertion/Deletion (INDEL) Mutations Various processes including replication and recombination errors, and transposons can result in insertions and deletions. INDELs can range from one or two nucleotides up to thousands of nucleotides. Unless by chance the INDEL is a multiple of three, INDELS in the protein encoding exonic regions of genes will result in a frame shift. Evolution has preserved the integrity of open reading frames of protein encoding genes by not placing nonsense codons in alternative exons. However, if a change in reading frame occurs due to an INDEL, there is no mechanism to Block: Foundations | BEAR [7 of 16] GENE EXPRESSION & REGULATION – PART 2 prevent a stop codon from occurring in the new reading frame. Because there are three stop codons out of 64, without any evolutionary constraints, there is a probability that once every three out of 64 codons (approximately once every 20 base pairs) there will be a stop codon in the new reading frame; thus, generation of a premature stop codon is virtually guaranteed when an INDEL occurs within a protein-encoding portion of an exon. However, as in the case of stop codons generated by nonsense mutations, INDEL-generated premature stop codons rarely give rise to truncated proteins. Truncated proteins are very toxic to the cell because they can compete with the normal full-length proteins, causing dysfunctional protein assemblies. The cell guards against these toxic truncated protein by destroying mRNAs with premature stop codons using the nonsense-mediated decay (NMD) surveillance machinery before translation occurs. REGULATION OF TRANSLATION Translation can be regulated by proteins that block ribosome location of the translation initiation site. A number of translational repressor proteins regulate translation by binding to the mRNA either at the 7-MeG cap at the 5’ end where the ribosome gains access to the transcript, or at a site near the AUG start of translation. Figure 6. Regulation of the translation of ferritin mRNA by the iron response element binding protein. Source: Pearson Education. The iron response binding protein (IRE-BP) regulates the synthesis of the iron storage protein ferritin. Ferritin is a protein involved in the storage of iron inside cells but may be released to remove excess iron from cells at high iron concentration. When iron stores are low, ferritin levels need to be decreased. The IRE-BP binds to the iron response element (IRE) located on the ferritin mRNA, upstream of the start codon. The binding of the IRE-BP to the IRE blocks translation initiation of the ferritin mRNA. In the presence of high levels of iron where ferritin levels need to increase, iron binds to an allosteric site on the IRE-BP causing it to dissociate from the mRNA and activating translation of the ferritin mRNA to produce ferritin. An important example of a global translational regulatory system is the mTOR pathway. mTOR stands for mammalian target of rapamycin. mTOR is a protein kinase that increases translation when nutrient levels of the cell are high. Rapamycin is an antibiotic that inhibits mTOR function. When cells are deprived of nutrients, including amino acids, the cell shuts down translation by activating the eIF4E-binding protein called 4E-BP1, which binds to translation initiation factor eIF4E and blocks its assembly with the 7-MeG cap, thereby inhibiting Block: Foundations | BEAR [8 of 16] GENE EXPRESSION & REGULATION – PART 2 translation. When nutrients and growth factors are at high levels during cell growth and proliferation, the mTOR complex becomes activated, leading to phosphorylation of 4E-BP1, causing it to dissociate from the eIF4E, and thereby stimulating global translation initiation. Another target of mTOR kinase activity is the ribosomal protein S6 kinase, which phosphorylates ribosomal protein S6 on the small subunit of the 40S ribosome. Phosphorylation of S6 ribosomal protein activates increased ribosome synthesis and assembly in response to growth factors. In cancer cells, due to mutations in cell signaling pathways, phosphorylation of mTOR may become growth factor-independent. Rapamycin and its derivatives have been used in chemotherapy to inhibit mTOR and restrict the growth of cancer cells. Figure 7. The mTOR pathway for regulating translation initiation in response to increased growth factors. mTOR activates the ribosomal S6 kinase, which increases ribosome biogenesis, and dissociates the inhibitory eIF4E binding protein from initiation factor eIF4E thereby activating translation initiation at the 5’ 7-meG cap at mRNAs encoding factors that increase cell growth, survival, division, and motility. Source: Cen & Amato (2012) OncoTargets & Therapy REGULATION OF TRANSLATION AND mRNA DECAY BY MICRORNAS MicroRNAs (miRNAs) are non-coding bind to mRNAs and either block the translation of the transcript or promote the nucleolytic destruction of the mRNA. A very important mechanism for the regulation or translation of mRNA levels in mammalian cells are short (21-22 nucleotide) non- coding RNAs referred to as microRNAs or miRNAs. Within the human genome, more than 600 miRNA genes have been discovered and verified but this number is expected to climb to well over a 1,000. miRNAs are critical in the regulation of human differentiation and development and play Block: Foundations | BEAR [9 of 16] GENE EXPRESSION & REGULATION – PART 2 important roles in many human diseases including cancer, neurodegenerative, cardiovascular, and metabolic diseases. MicroRNAs (miRNAs) bind to mRNAs and either block the translation of the transcript or promote the nucleolytic destruction of the mRNA. miRNAs are transcribed as larger primary transcript precursors (pri-miRNAs) and processed in the nucleus into pre-miRNAs. Pre- miRNAs can also be derived from introns. The pre-miRNAs are exported to the cytoplasm by the transporter Exportin 5 and cleaved into 21-22 nt double-stranded miRNAs by the enzyme dicer. The mature miRNA binds to a set of proteins including the Argonaute protein (Ago) to form the RNA-induced silencing complex or RISC. RISC recognizes specific mRNAs guided by the complementarity of the miRNA “seed sequences” to short regions of the 5’ and 3’ UTRs. Binding of the RISC to the mRNA can induce either translational repression or cleavage of the mRNA target – depending on several factors including the number of base pairs between the miRNA and the mRNA target. Figure 8, The Synthesis of microRNAs. Source: Alberts et al. (2015) Molecular Biology of the Cell 6th Edition REGULATION OF mRNA DECAY There are both specific and general degradation pathways for RNAs. These processes, although not usually thought of as genetic events, are very important in controlling the level of gene expression. Nonsense mediated decay (NMD) is an example of a specific degradation pathway that is used to check mRNAs for the existence of premature stop (nonsense) codons that may have been created by mutations, low fidelity transcription, or faulty RNA processing. If a premature stop codon is detected, the mRNA is degraded by enzymes associated with the NMD complex. Premature stop codons may also be generated naturally by Block: Foundations | BEAR [10 of 16] GENE EXPRESSION & REGULATION – PART 2 consequence of alternative splicing where NMD serves to regulate which alternatively spliced transcripts are present within a cell. Figure 9. Nonsense mediated decay (NMD) functions in both mRNA quality control of damaged mRNA and in the selective degradation of alternatively spliced transcripts. Source: Nickless et al. (2017) Cell & Bioscience. A general pathway for mRNA degradation also exists. All mRNAs throughout their lifetime undergo progressive shortening of their poly(A) tails by poly(A) nucleases. The shortening process intensifies when an mRNA reaches the cytoplasm after export from the nucleus. Control sequences in the 3 UTR of messenger RNAs) are binding sites for proteins that can function to either stabilize or destabilize mRNAs. The poly(A) tail is partially protected from degradation by a cytoplasmic poly(A) binding protein (PABPC). However, over the lifetime of the mRNA, PABPC is displaced and the progressive degradation of the tail occurs. Once the tail is degraded to about 10 adenine residues, the mRNA becomes highly susceptible to cellular nucleases and is quickly and completely degraded. Figure 10. mRNA decay may occur from either the 5’ or 3’ end. In both pathways decapping of the 7-MeG cap at the 5’ end is a pre-requisite step. Source: Alberts et al. (2015) Molecular Biology of the Cell 6th Edition An example of the regulation of general mRNA degradation is the control of transferrin receptor mRNA degradation by the iron response element binding protein (IRP). We previously discussed how the IRP could block translation of ferritin mRNA when iron levels were low, where less ferritin protein is needed for iron storage. The IRP can also bind to the IRE Block: Foundations | BEAR [11 of 16] GENE EXPRESSION & REGULATION – PART 2 located in the 3’ UTR of the transferrin receptor protein mRNA. Transferrin is a protein that transports iron in the blood delivering it to red blood cells that use iron to make hemoglobin. The transferrin receptor functions to internalize iron-bound transferrin to the inside of the cell. When iron levels are low, transferrin receptor protein is up-regulated by the binding and stabilization of the transferrin mRNA, allowing translation to proceed and to increase iron uptake. When iron levels are high, the IRP binds iron and is released from the 3’ UTR, increasing the degradation of the transferrin receptor mRNA, thereby decreasing the level of transferrin receptor protein. Figure 11. Regulation of the translation of transferrin mRNA by the iron response element binding protein. Source: Pearson Education, Unfolded Proteins are degraded by lysosomes or through the ubiquitin pathway by large proteolytic complexes called proteasomes. Cells continuously synthesize proteins and degrade them into amino acids. Proteins have lifetimes that range from a few minutes to weeks or more. The functions of protein degradation are (1) to utilize amino acids as an energy source in times of metabolic need, (2) to eliminate abnormal misfolded proteins whose accumulation would be harmful to the cell, and (3) to facilitate metabolic regulation by controlling the level of enzymes and regulatory proteins. Lysosomes, which contain several proteases, degrade proteins that have been enclosed in vacuoles through vacuole-lysosome fusion. The 26S proteasome is a large multi-subunit protein complex that binds and degrades abnormal proteins that have been covalently tagged with the small protein called ubiquitin. Ubiquitin can form short polymers when attached to proteins. Abnormal proteins may include products of mutated genes or mistranslated messenger RNAs. Mutant proteins derived from genes carrying trinucleotide repeat expansions are often misfolded and are also degraded by the ubiquitin proteasome pathway. CASE DISCUSSION Case 1 For Case 1, the following points explain how the mRNA COVID19 vaccine works: In the normal course of the COVID-19 infection, the virus enters the cell by attaching to the ACE2 receptors of the cells in respiratory tract via the spike protein on the viral capsid and is then endocytosed into the cell. Once inside the cell, the virus disassembles to release its single-stranded RNA genome, which is translated by the cell’s own translation machinery. The viral encoded replicase replicates many copies of the viral genome, which are then packaged by newly translated viral assembly proteins. The new viral particles are released from the infected cells by exocytosis. Block: Foundations | BEAR [12 of 16] GENE EXPRESSION & REGULATION – PART 2 The strategy of the COVID-19 vaccine is to create a synthetic mRNA that encodes the spike protein. The mRNA contains all the essential sequences we have previously described that are required for expression: the 5’ 7-MeG cap, the 5’ UTR, the protein coding region, the 3’ UTR and the poly(A) tail. An additional feature of the synthetic viral spike protein mRNA is that the uridine residues in the mRNA are substituted with methylpseudouridines, which make the mRNA resistant to cellular nucleases and reduce the antigenicity of the mRNA so that it won’t be recognized as a foreign mRNA to be destroyed. The synthetic spike protein mRNA is delivered to cells in a lipid encased hydrophobic nanoparticle that can penetrate the cell membrane. Once inside the cell, the mRNA is translated to make spike protein, which is then displayed on the outside surface of the cell to illicit an immune response from B-cells and T-cells. Case 2 For Case 2, here are the key discussion points: The patient in Case 2 is showing the classic signs and symptoms of Duchenne muscular dystrophy (DMD): difficulty walking at a young age; enlarged calf muscles due to substitution of fat cells for dying myocytes; and characterizes the difficulty in rising from a lying or sitting position to assume an erect position. He also has a maternal uncle who most likely died of the same or similar disease many years ago, indicating that the disease was passed down by maternal inheritance, and suggesting an X-chromosome linked disorder. DMD and its more milder form Becker muscular dystrophy (BMD) are caused by mutations in the DMD gene, which encodes the muscle cell protein dystrophin. DMD is located on the p-arm of the X chromosome. The disease affects one in 3500 males in the U.S. Diagnosis of DMD usually occurs between the ages of two and six years, while BMD shows symptoms later, usually between the ages of ten and twenty years. Both diseases cause the progressive damage of skeletal and cardiac muscle. In the case of DMD, patients seem normal at birth but begin to show signs as soon as they begin to walk. The first muscles to show weakness are in the pelvis, causing the child to show Gower’s sign. As the disease progresses, the calf muscles become significantly enlarged due fat replacing the necrotic muscle. Most DMD patients die between the ages of 20 and 40 due to cardiomyopathy or diaphragmatic failure. In the case of BMD, symptoms are delayed and much milder, and patients have been known to live into their fifties and early sixties. The diagnosis of DMD and BMD involves several tests. Measurement of creatine kinase enzyme levels provides nonspecific evidence of muscle damage, which can be confirmed by a procedure called electromyography, which records the electrical activity of the muscle tissue using needle electrodes inserted into the muscle. Examination of the muscle fibers in a cross-section biopsy of calf muscle usually shows muscle fiber necrosis and invasion by macrophages. Immunohistochemistry of the sections will detect the absence of dystrophin at the rims of the fibers. Special microarrays have been Block: Foundations | BEAR [13 of 16] GENE EXPRESSION & REGULATION – PART 2 designed to detect the most common DMD and BMD mutations, but sometimes whole genome sequencing is required to identify less-common mutations. It is important to determine the exact type of mutation to provide useful genetic counseling. Dystrophin is the largest gene in the genome spanning over 2.5 million base pairs, and is expressed primarily in muscle, but low levels of normal dystrophin can be detected in other tissues. The dystrophin gene contains 79 exons and encodes a single polypeptide chain with a molecular weight of 427,000 Da. In general, DMD patients have the complete elimination of dystrophin expression due to INDEL mutations and splicing mutations that all lead to nonsense mediated decay of the DMD transcript, causing the absence of translation of the dystrophin mRNA. BMD patients show point mutations and in-frame INDELs, which allow some dystrophin to be made. Because of the fatal nature of DMD, multiple therapeutic approaches are being investigated. The standard therapy is to use steroids to reduce inflammation and modified exercises to try maintaining muscle strength, although there is debate as to whether exercise is helpful or harmful. The two experimental approaches are gene therapy and cell therapy. In cell therapy, the goal is to use embryonic stem cell replacement to attempt to replace cardiac and skeletal muscle with newly differentiated myocytes. This approach is still in technical development and years from being in the clinic. Block: Foundations | BEAR [14 of 16] GENE EXPRESSION & REGULATION – PART 2 SUMMARY OF KEY CONCEPTS Regulation of alternative RNA splicing is the primary mechanism for generating different protein isoforms. Regulation is controlled by splicing activators and repressors. Nonsense mediated decay (NMD) is an important mechanism for the prevention of the expression of abnormal proteins due to splice site mutations that result in aberrant splicing and intron retention leading to a translational reading frameshift. Regulation of translation initiation occurs by proteins that interfere with access of the ribosomes to the 7-MeG cap or bind to the 5’ untranslated region and block the scanning ribosome from reaching its start codon. MicroRNAs regulate gene expression by interfering with translation or increasing mRNA degradation RNA and protein turnover ensure that gene products are maintained at optimal levels throughout the life of the cell. PRACTICE EXAM QUESTIONS 1. mTOR regulates which one of the following processes? A. mRNA splicing B. transcription initiation C. translation initiation D. mRNA decay E. microRNA synthesis 2. How does the RNA silencing complex (RISC) regulate gene expression? A. Enhances transcription of mRNAs B. Increases pre-mRNA splicing C. Enhances translational initiation D. Represses pre-mRNA splicing E. Enhances mRNA decay 3. What is the most common effect of an mRNA splice site mutation on gene regulation? A. Causes changes in the spliceosome B. Inhibits regulatory promoter function C. Causes decay of the transcript D. Inhibits core promoter function E. Causes changes in chromatin structure 4. Which one of the following statements about alternative mRNA splicing is not true? A. It is regulated by splicing activators and repressors B. An intron can become an exon and an exon can become an intron C. It generates multiple proteins from the same protein-encoding gene D. It generates multiple mRNAs from the same protein-encoding gene E. It is important in the expression of a few genes, but most mRNAs are constitutively spliced 5. What would be the most expected result of a mutation that deleted the iron response element (IRE) in the 5’ UTR of the ferritin mRNA transcript? A. Ferritin protein would not be synthesized Block: Foundations | BEAR [15 of 16] GENE EXPRESSION & REGULATION – PART 2 B. Iron would not be transported C. Ferritin levels would increase significantly D. Iron would rise to toxic levels E. Hemoglobin synthesis would be inhibited Key: 1-C; 2-E; 3-C; 4-E; 5-C Block: Foundations | BEAR [16 of 16]