Lecture 3: Amino Acids and Proteins PDF
Document Details
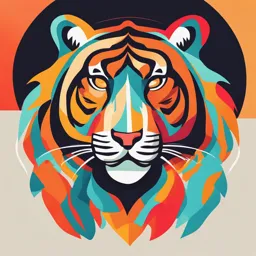
Uploaded by ProfoundBrown
Tags
Summary
This document is a lecture on amino acids and proteins, providing an overview of their structure, properties, and stereochemistry. The lecture also covers the role of these molecules in various biological processes and provides examples.
Full Transcript
1 Lecture 3 Amino acids and proteins 2 Proteins play an enormous variety of roles: transport and storage of small molecules structural framework of cells and tissues muscle contraction immune responses blood clotting e...
1 Lecture 3 Amino acids and proteins 2 Proteins play an enormous variety of roles: transport and storage of small molecules structural framework of cells and tissues muscle contraction immune responses blood clotting enzymes—the biological catalysts 3 Proteins play an enormous variety of roles: 4 Structures of the a-Amino Acids To the a-carbon of every amino acid are attached: an amino group a hydrogen atom a side chain (“R” group) Different a-amino acids are distinguished by their side chains. 5 Structures of the a-Amino Acids The pKa of the carboxylic acid is about 2 The pKa of the a-amino group is about 10 Therefore, at physiological pH both the carboxylic acid group and the a-amino group will be ionized, to yield the zwitterion form Amino acids are typically written in their zwitterionic form 6 Structures of the a-Amino Acids There are 20 different kinds of amino acids are commonly incorporated into proteins. The amino acids are classified by their side chains (R-groups). Two amino acids, selenocysteine and pyrrolysine, are encoded genetically and incorporated into proteins; however, they are found in a relatively small number of proteins. For the purposes of this introductory discussion we will focus our attention on the 20 common amino acids. 7 Stereochemistry of the a-Amino Acids When a carbon atom has four different substituents attached to it, it is said to be chiral, or a stereocenter. All a-amino acids have a stereocenter at the a-carbon. The sole exception is glycine, whose R-group is hydrogen, therefore is achiral. 8 Stereochemistry of the a-Amino Acids 9 Stereochemistry of the a-Amino Acids 10 Stereochemistry of the a-Amino Acids a-Amino acids’ stereochemistry is designated as D- or L-, which is best visualized from its Fischer projection There is a preference for L-amino acids in proteins Compare this to the preference for D-configured carbohydrates. 11 Stereochemistry of the a-Amino Acids The preference for L-amino acids in natural proteins has two important consequences: 1.The surface of any given protein, which is where the interesting biochemistry occurs, is asymmetric. This asymmetry is the basis for the highly specific molecular recognition of binding targets by proteins. 2. The stereochemistry of the amino acids plays an important role in the formation of so-called “secondary structure” (i.e., a-helices and b-strands) and thereby the overall structure of proteins. 12 Properties of the Amino Acid Side Chains The 20 common amino acids are classified by their side chains: Aliphatic Hydroxyl or sulfur-containing Aromatic Basic Acidic and their amides 13 Properties of the Amino Acid Side Chains The more hydrophobic amino acids such as isoleucine are usually found within the core of a protein molecule, where they are shielded from water. Proline, is the only amino acid in this group in which the side chain forms a covalent bond with the a–amino group. The proline side chain has a primarily aliphatic character; however, it is frequently found on the surfaces of proteins due to its unique structural constraints. The rigid ring of proline is well-suited to those sites in a protein structure where the protein must fold back on itself (so-called “turns”). 14 Properties of the Amino Acid Side Chains Methionine and tyrosine are fairly hydrophobic, but display more hydrophilic character than their aliphatic analogs. The –OH group of serine and the –SH group of cysteine are good nucleophiles and often play key roles in enzyme activity. 15 Properties of the Amino Acid Side Chains Cysteine is noteworthy in two additional respects: 1.The side chain has a pKa = 8.3, so it can ionize at moderately high pH: 2. The oxidation of two cysteine side chains yields a disulfide bond. The product of this oxidation is given the name cystine. 16 Properties of the Amino Acid Side Chains The oxidation of two cysteine side chains yields a disulfide bond. 17 Properties of the Amino Acid Side Chains Phenylalanine is one of the most hydrophobic amino acids. Tyrosine and tryptophan have some hydrophobic character as well, but it is tempered by the polar groups in their side chains. 18 Properties of the Amino Acid Side Chains The aromatic amino acids, like most highly conjugated compounds, absorb light in the near-ultraviolet region of the spectrum. This characteristic is frequently used for the detection and/or quantitation of proteins, by measuring absorption at 280 nm. Properties of the Amino Acid Side Chains 19 Lysine (pKa = 10.0) and arginine (pKa = 12.5) are the most basic amino acids. Their side chains are almost always positively charged under physiological conditions. The guanidino group of arginine makes it the most basic amino acid due to the resonance stabilization of the protonated side chain. Properties of the Amino Acid Side Chains 20 Aspartic acid (pKa = 3.9) and glutamic acid (pKa = 4.2) typically carry negative charges at pH 7. These amino acid residues are often referred to as aspartate and glutamate. (i.e., the conjugate bases rather than the acids). Unlike their acidic analogs, asparagine and glutamine have uncharged polar side chains. Like the basic and acidic amino acids, Asn and Gln are hydrophilic and tend to be on the surface of a protein molecule, in contact with the surrounding water. 21 Properties of the Amino Acid Side Chains Amino acids can undergo post-translational modification resulting in modified amino acids with unique properties 22 23 Peptides and the Peptide Bond Amino acids can be covalently linked together by formation of an amide bond between the a-carboxylic acid group on one amino acid and the a-amino group on another. This bond is often referred to as a peptide bond, and the products formed by such a linkage are called peptides. A peptide composed of 2 amino acids is called a dipeptide. A peptide composed of 4 amino acids is called a tetrapeptide. 24 Peptides and the Peptide Bond The amide bond formation leaves an amino group available on one end of the tetrapeptide and a carboxylate group on the other, called the N-terminus and C-terminus, respectively. The portion of each amino acid remaining in the chain is called an amino acid residue. When specifying an amino acid Glu-Gly-Ala-Lys residue in a peptide, the suffix –yl may be used (e.g., glycinyl, or alanyl). EGAK 25 Peptides and the Peptide Bond Chains containing only a few amino acid residues (like a tetrapeptide) are collectively referred to as oligopeptides. If the chain is longer (>15-20 residues), it is called a polypeptide. Polypeptides greater than ~50 residues are generally referred to as proteins. Note that most globular proteins contain 250–600 amino acid residues. Peptide and protein sequences are always written in order from the N-terminus to the C-terminus using the three-letter or one-letter abbreviations (e.g., Glu-Gly-Ala-Lys or EGAK). 26 Peptides and the Peptide Bond Blocking N-terminus and C-terminus in proteins 27 Peptides and the Peptide Bond The peptide bond can be considered a resonance hybrid of two forms. 28 Peptides and the Peptide Bond A peptide bond exist in two possible configurations, trans and cis, which are related by rotation around the CCO-N bond. The trans form is usually favored because in the cis configuration the R groups can sterically interfere. The major exception is the bond in the sequence X-Pro where X is any other amino acid. In this bond the cis configuration is sometimes allowed, although the trans configuration is still favored by about 4:1. 29 Peptides and the Peptide Bond While hydrolysis of peptide bonds is thermodynamically favored in aqueous solutions, the reaction is exceedingly slow at physiological pH and temperature Peptide bond hydrolysis can be achieved by: o Strong mineral acid (e.g., 6 M HCl) cleaves all peptide bonds (including the Asn and Gln amide bonds) o Chemicals that cleave at specific sites (e.g., CNBr cleaves at Met) o Proteolytic enzymes (proteases) that cleave at specific sites Peptides and the Peptide Bond 30 Proteins: Polypeptides of Defined Sequence 31 Every protein has a defined number and order of amino acid residues. As with the nucleic acids, this sequence is referred to as the primary structure of the protein. Sequence homology between myoglobin in humans vs. whales. From Gene to Protein 32 Remember the central dogma: o DNA is transcribed into RNA o RNA is translated into protein Therefore, DNA codes for protein. The primary structure (sequence) of a protein corresponds to the DNA (gene) sequence. The genetic code 33 Many proteins, such as insulin, undergo post-translational modification. Protein Structure: 34 Regular Ways to Fold the Polypeptide Chain Protein molecules have four levels of structural organization: Primary structure - the amino acid sequence Secondary structure - local folding into regularly repeating units Tertiary structure - overall folding of a monomeric protein or subunit Quaternary structure - subunit association Secondary Structure: 35 Regular Ways to Fold the Polypeptide Chain Three-dimensional folding of the protein myoglobin. This “cartoon” shows the polypeptide main chain as helices connected by thick lines. Side chains are shown as thin lines. Individual helical regions are color- coded, with the peptide N-terminus shown in blue and the C-terminus shown in red. This protein binds a heme group. Secondary Structure: 36 Regular Ways to Fold the Polypeptide Chain Of the several possible secondary structures for polypeptides, the most frequently observed are: a helix b sheet Secondary Structure: 37 Regular Ways to Fold the Polypeptide Chain Rotation around the bonds in a polypeptide backbone. Two adjacent amide planes are shown in light green. Rotation is allowed only about the Namide–Ca (f, phi) and Ca–Ccarbonyl (y, psi) bonds. Positive rotation is clockwise as seen from the a-carbon. The extended conformation of the chain shown here corresponds to f = +180 , y = +180. Secondary Structure: 38 Regular Ways to Fold the Polypeptide Chain (a) In the a-helix the hydrogen bonds are within a single polypeptide chain and are almost parallel to the helix axis. The alignment of amide bonds in the helix gives rise to a macrodipole moment. The N-terminal end of the helix has a d+ charge and the C-terminal end has a d- charge. (b) In the b-sheet, the hydrogen bonds are between adjacent chains and are nearly perpendicular to the chains. (c) The 310 helix is found in proteins; but, is less common than the a-helix. Secondary Structure: 39 Regular Ways to Fold the Polypeptide Chain Secondary structures that display a predominantly hydrophobic face opposite a predominantly hydrophilic face are said to be “amphiphilic ” (or “amphipathic”). o a-helix will have side chains of similar polarity every 3–4 residues o b-strand will have alternating polar and nonpolar side chains. Secondary Structure: 40 Regular Ways to Fold the Polypeptide Chain In the a-helix the side chains radiate away from the center of the helix. In the b-sheet the side chains are located on opposite faces of the sheet defined by the H-bonded main chain amides. Secondary Structure: 41 Regular Ways to Fold the Polypeptide Chain Idealized Helices: These hypothetical structures show the effect of varying the number (n) of polypeptide residues per turn of a helix. The n = 4 and n = 3 helices are right-handed, the n = -3 helix is left-handed, an n = 2 (a flat ribbon) has no handedness. The right-handed a-helix (not shown), with n = 3.6, is intermediate between the n = 3 and n = 4 structures. Secondary Structure: 42 Regular Ways to Fold the Polypeptide Chain Parameters of an a-helix: The carbonyl oxygen, on residue i, is hydrogen-bonded to the amido proton that is located four residues in the direction of the C-terminus (i.e., on residue i + 4). A loop of 13 atoms is formed. The 310 helix has exactly 3.0 residues per turn and a 10-member hydrogen-bonded loop. The helix could also be called a 3.613 helix. Hydrogen bonds tend to be linear, so the atoms in polypeptide helices should lie on a straight line. Secondary Structure: 43 Regular Ways to Fold the Polypeptide Chain Hydrogen bonding patterns for the a- and 310 helices. The structures are represented in a diagrammatic way to simplify counting the atoms in each H-bonded loop. For example, there are 13 atoms in the H-bonded loop corresponding to the (3.613) helix. Secondary Structure: 44 Regular Ways to Fold the Polypeptide Chain Parameters for b-sheets: A sheet is composed of two or more strands. Each residue in the strand is rotated by 180o with respect to the preceding one, which makes each strand an n = 2 “helix.” If the chains are also folded in the pleated fashion, linear hydrogen bonds can occur between adjacent strands. The two strands can be arranged such that the N-terminus to C- terminus orientations are in opposite directions called “antiparallel.” o The hydrogen bonds between antiparallel strands are linear. The two strands can be arranged such that the N-terminus to C- terminus orientations are in the same direction called “parallel.” o The hydrogen bonds between parallel strands are not linear. Secondary Structure: 45 Regular Ways to Fold the Polypeptide Chain An antiparallel arrangement of strands forming b-sheets. Secondary Structure: 46 Regular Ways to Fold the Polypeptide Chain A parallel arrangement of strands b-sheets. Secondary Structure: 47 Regular Ways to Fold the Polypeptide Chain The p helix: Also known as an “a-bulge” or “p-bulge.” It is found in ~15% of protein sequences in the Protein Data Bank. It occurs only once in any given sequence. 85% appears to be the result of a mutation event that results in the insertion of an amino acid into an a-helix. This creates a bulge in the helical structure. Secondary Structure: 48 Regular Ways to Fold the Polypeptide Chain The p-helix conformation: On the left, a main-chain rendering of the C-terminal a-helix from S. aureus is shown On the right, the analogous p-helix from a Gly insertion mutant is shown. Note that the inserted Gly carbonyl does not form an intrahelical H- bond. 49 Proteins of human organism Fibrous Proteins Globular Proteins 50 Fibrous Proteins: Structural Materials of Cells Fibrous proteins: Have a filamentous, or elongated, form. Most of them play structural roles in animal cells and tissues. Include the major proteins of skin and connective tissue and of animal fibers like hair and silk. The amino acid sequence of each of these proteins favors a particular kind of secondary structure o Confers on each protein a particular set of appropriate mechanical properties. 51 Fibrous Proteins: Structural Materials of Cells 52 Fibrous Proteins: Structural Materials of Cells The a-keratins are the major proteins of hair and fingernails and comprise a major fraction of animal skin. The a-keratins are members of a broad group of intermediate filament proteins, which play important structural roles in the nuclei, cytoplasm, and surfaces of many cell types. Predominantly a-helical in structure. Built on a coiled-coil a–helical structure. 53 Fibrous Proteins: Structural Materials of Cells Proposed structure for keratin-type intermediate filaments. Two monomers (a) pair via a parallel coiledcoil to form the 50-nm-long dimer (b). These then associate to form first a 4- strand protofilament (c) and then an 8-strand protofibril (d). The regular spacing of 25 nm along the fibers is accounted for by the overlap. 54 Fibrous Proteins: Structural Materials of Cells Fibroin is a sheet protein. Almost half of its residues are glycine. Silkworm fibroin contains long regions of antiparallel b-sheet, with the polypeptide chains running parallel to the fiber axis. The b-sheet regions comprise almost exclusively multiple repetitions of the sequence: [Gly-Ala-Gly-Ala-Gly-Ser-Gly-Ala-Ala-Gly-(Ser-Gly-Ala-Gly-Ala-Gly)8] In silkworm fibroin almost every other residue is Gly and that between them lie either Ala or Ser residues. 55 Fibrous Proteins: Structural Materials of Cells The structure of silk fibroin. (a) A three-dimensional view of the stacked sheets of fibroin. The region shown contains only alanine and glycine residues. (b) Interdigitation of alanine or serine side chains and glycine side chains in fibroin. The plane of the section is perpendicular to the folded sheets. Fibrous Proteins: Structural Materials of Cells 56 Collagen: Collagen fibers are built from triple helices of polypeptides rich in glycine and proline. Because it performs such a wide variety of functions, collagen is the most abundant single protein in most vertebrates. In large animals, it may make up a third of the total protein mass. oForms the matrix material in bone oConstitutes the major portion of tendons oAn important constituent of skin Fibrous Proteins: Structural Materials of Cells 57 Collagen Structure: The basic unit of the collagen fiber is the tropocollagen molecule. A triple helix of three polypeptide chains, ~1000 residues in length. Left-handed helices, with about 3.3 residues/turn. The chains wrap around one another in a right-handed sense. Hydrogen bonds are between the chains. Every third residue can be only glycine Hydroxyproline and hydroxylysine are present. A repetitive motif in the sequence is of the form Gly–X–Y, where X is often proline and Y is proline or hydroxyproline. Fibrous Proteins: Structural Materials of Cells 58 Scurvy is a connective tissue disease from a deficiency in Vitamin C (ascorbic acid). Collagen fibers are weakened. It is caused by failure to hydroxylate prolines and lysines in collagen. There is less H-bonding between the chains of tropocollagen. Fibrous Proteins: Structural Materials of Cells 59 Lysine side chains can oxidize to aldehyde derivatives. These can react with either a lysine residue or with one another via an aldol condensation and dehydration to produce a cross-link: This process continues through life, and the accumulating cross-links make the collagen steadily less elastic and more brittle. Fibrous Proteins: Structural Materials of Cells 60 This process continues through life, and the accumulating cross-links make the collagen steadily less elastic and more brittle. Fibrous Proteins: Structural Materials of Cells 61 The structure of collagen fibers: 62 Biosynthesis and assembly of collagen: Steps 1–4 occur in the endoplasmic reticulum and cytosol of collagen-synthesizing cells. Steps 6 and 7 occur in the extracellular region. Fibrous Proteins: Structural Materials of Cells 63 The protein elastin forms elastic fibers found in ligaments and blood vessels. Rich in glycine, alanine, and valine. Very flexible and easily extended. Has little secondary structure at all in a conformation that approximates a random coil. Contains lysine side chains, which can cross-link. Four lysine side chains can be combined to yield a desmosine cross-link. Globular Proteins: 64 Teritary Structure and Functional Diversity Globular proteins: Carry out most of the chemical work of the cell o Synthesis o Transport o Metabolism Possess secondary structures Folded into compact tertiary structures Many carry prosthetic groups o small molecules that may be noncovalently or covalently bonded to the protein (e.g., heme in myoglobin). Globular Proteins: 65 Teritary Structure and Functional Diversity General rules governing tertiary folding: All globular proteins have a defined inside and outside. o There is no particular distribution pattern of hydrophobic or hydrophilic residues in the primary structure. o The tertiary structure folding causes hydrophobic residues to be packed mostly on the inside and the hydrophilic residues are on the surface, in contact with water. Sheets are usually twisted, or wrapped into barrel structures. Globular Proteins: 66 Teritary Structure and Functional Diversity a)The distribution of hydrophilic and hydrophobic residues in globular proteins. The three-dimensional structure of myoglobin. The hydrophobic amino acids (green) cluster about the hydrophobic heme cofactor (orange) and on the inside of the molecule. The hydrophilic residues (red) are on the solvent- exposed surface of the protein. Globular Proteins: 67 Teritary Structure and Functional Diversity General rules governing tertiary folding: The polypeptide chain can turn corners in a number of ways, to go from one b-segment or a-helix to the next. § One kind of compact turn is called a b-turn that causes a reversal of direction in 4 residues. § The carbonyl of residue i hydrogen-bonds to the amide hydrogen of residue i + 3. o The g-turn is an even tighter turn, H-bonding is to residue i + 2. o Proline often plays a role in turns and is also a breaker of helices. o Bends and turns most often occur at the surface of proteins. Not all parts of globular proteins can be conveniently classified as a-helix, b-sheet, or turns. Factors Determining Secondary 68 and Tertiary Structure Most of the information for determining the 3-D structure of a protein is carried in the amino acid sequence of that protein. Under harsh conditions, a protein loses its functional 3-D structure. This process is called denaturation. Denaturing conditions include: o Increased temperature o pH becomes extremely acidic or alkaline o Organic solvents or urea Factors Determining Secondary 69 and Tertiary Structure The denaturation and refolding of ribonuclease A: This schematic drawing depicts the classic RNase A refolding experiment of Anfinsen. Factors Determining Secondary 70 and Tertiary Structure The Thermodynamics of Folding The folding of a globular protein is clearly a thermodynamically favorable process under physiological conditions. The overall free energy change for folding must be negative. This negative free energy change is achieved by a balance of several thermodynamic factors: o Conformational Entropy o Charge–Charge Interactions o Internal Hydrogen Bonds o van der Waals Interactions Factors Determining Secondary 71 and Tertiary Structure The stability of the folded structure of a globular protein depends on the interplay of three factors: o The unfavorable conformational entropy change, which favors the unfolded state. o The favorable enthalpy contribution arising from intramolecular interactions. o The favorable entropy change arising from the burying of hydrophobic groups within the molecule. Factors Determining Secondary 72 and Tertiary Structure The Role of Disulfide Bonds Some folded proteins are stabilized by internal disulfide bonds, in addition to noncovalent forces. Disulfide bonds bonds are relatively rare and are found primarily in proteins that are exported from cells, such as ribonuclease, BPTI, and insulin. One explanation is that the environment inside most cells is reducing and tends to keep sulfhydryl groups in the reduced state. External environments, for the most part, are oxidizing and stabilize bridges. 73 Dynamics of Globular Protein Structure Kinetics of Protein Folding The folding of globular proteins from their denatured conformations is a remarkably rapid process, often complete in less than a second. Protein folding is not a completely random search through a vast conformational space. Rapid kinetic studies, using a variety of physical techniques to monitor different aspects of protein structure, show that folding takes place through a series of intermediate states. Dynamics of Globular Protein Structure 74 A simplified representation of the folding pathway for a protein: “U” is the unfolded or denatured state. “F” is the folded or native state. “I” “on-pathway” are intermediate states. Off-pathway states include aggregates and other non-native states that may be kinetic or thermodynamic “dead-ends” Thus, the paths to these states are generally shown as irreversible. In fact, not all pathways leading to such states are irreversible. Copyright © 2013 Pearson Canada Inc. 6 - 74 75 Dynamics of Globular Protein Structure In the “energy landscape” model, the trajectory of protein folding is “downhill”— it proceeds with a decrease in free energy. Protein folding can be rapid but seems to involve well-defined intermediate states. Folding can be delayed by trapping of molecules in “off-pathway” states. 76 Dynamics of Globular Protein Structure Chaperones: Some proteins require the action of specialized proteins called molecular chaperones to achieve proper folding. Functions to keep the newly formed protein out of trouble. o improper folding o aggregation Dynamics of Globular Protein Structure 77 The GroEL-GroES chaperonin: 78 Dynamics of Globular Protein Structure Protein misfolding is the basis for several diseases, including Alzheimer’s disease and Parkinson’s disease. 79 Dynamics of Globular Protein Structure Protein misfolding Quaternary Structure of Proteins 80 The four levels of protein structure: Quaternary Structure of Proteins 81 Quaternary Structure of Proteins 82 Multisubunit Proteins: Homotypic Protein–Protein Interactions The interactions between the folded polypeptide chains in multisubunit proteins are of the same kinds that stabilize tertiary structure o salt bridges o hydrogen bonding o van der Waals forces o the hydrophobic effect o disulfide bonding These interactions provide the energy to stabilize the multisubunit structure. Association of polypeptide chains to form specific multisubunit structures is the quaternary level of protein organization. Quaternary Structure of Proteins 83