Biochemistry Lecture 1 PDF
Document Details
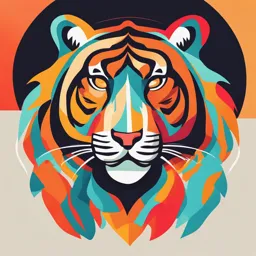
Uploaded by ProfoundBrown
Tags
Summary
This document provides a lecture on the fundamentals of biochemistry. It covers topics such as the structure of living matter, various macromolecules, non-covalent interactions, and the role of water in biological processes.
Full Transcript
1 Lecture 1 Biochemistry 2 MS Teams: Biochemistry 2023/2024 3 4 5 6 Attendance in laboratories and seminars is mandatory. Student can miss 1 laboratory and 1 seminar per semester without excuse Student has 7 days to deliver a doctors excuse for classes...
1 Lecture 1 Biochemistry 2 MS Teams: Biochemistry 2023/2024 3 4 5 6 Attendance in laboratories and seminars is mandatory. Student can miss 1 laboratory and 1 seminar per semester without excuse Student has 7 days to deliver a doctors excuse for classes on which he/she was absent, excuse should be sent by e-mail to course coordinator ([email protected]) Student cannot be late for classes, being late once no more than 15 minutes is permitted, being late more than 15 minutes is treated as absence every second and subsequent lateness will result in losing 5 points being late = arriving after attendance has been taken 7 Student has 7 days to deliver a doctors excuse for classes on which he/she was absent, excuse should be sent by e-mail to course coordinator MAIL: Attachment: doctors excuse photo Students Number: ….. Students Name: ….. Type of class (lab/sem): ….. Date of class: ….. [email protected] 8 Total: 200 points Exam (final): 100 Winter midterm: 25 Summer midterm: 25 Seminars: 10 winter + 10 summer Labs: 10 winter + 10 summer Lectures: 5 winter + 5 summer 9 Literature Harpers Illustrated Biochemistry (at least 29th Edition) -Robert Murray et al at least Fourth Edition of Lubert Stryer's Biochemistry Biochemistry concepts and connections – Dean Appling et al 10 Biochemistry The actual lecture… 11 What Is Biochemistry? Biochemistry is the branch of science that seeks to describe the structure, organization, and functions of living matter in molecular terms. Biochemistry can be divided into three principal areas: 1. The structural chemistry of the components of living matter and relationships of biological function to chemical structure. 2. Metabolism, the totality of chemical reactions that occur in living matter. 3. Genetic biochemistry, the chemistry of processes and substances that store and transmit biological information. This third area is also the province of molecular genetics, a field that seeks to understand heredity and the expression of genetic information in molecular terms. Biochemistry as a Chemical Science 12 13 Biochemistry as a Chemical Science Macromolecules Proteins Polysaccharides Nucleic acids Lipids Many of the macromolecules are polymers, which are formed by condensation reactions of the monomers. 14 Biochemistry as a Chemical Science Biochemistry as a Chemical Science 15 16 17 Biochemistry as a Chemical Science 18 Biochemistry as a Chemical Science Biological Functions of the macromolecules: Polysaccharides – structure, energy storage Nucleic acids – storage and transcription of genetic information Proteins – structure, enzymes, hormones, receptors Lipids – energy storage, membranes 19 Biochemistry as a Biological Science The cell is the basic unit of biological organization. 20 Biochemistry as a Biological Science The prokaryotes are always unicellular. Includes the true bacteria (eubacteria) and an ancient class called archaea. A plasma membrane and usually a rigid cell wall surrounds the cell. The cytoplasm, containing the cytosol is inside the cell. The DNA is in the form of one or more molecules that exist free in the cytosol. The ribosomes, where protein synthesis occurs is free in the cytosol. The cell surface may carry pili, which aid in attaching the organism to other cells or surfaces, and flagellae, which enable it to swim. 21 Biochemistry as a Biological Science The cell is the basic unit of biological organization. 22 Biochemistry as a Biological Science The cell is the basic unit of biological organization. 23 The Matrix of Life: Weak Interactions in an Aqueous Environment 24 25 The Nature of Noncovalent Interactions Some molecules interact because they possess dipole moments. 26 27 The Nature of Noncovalent Interactions The hydrogen bond. The figure shows an idealized H bond that might exist, for example, between an alcohol (the donor) and a keto compound (the acceptor). The H-bond is represented by a dotted line between the H and the acceptor atom. The Nature of Noncovalent Interactions 28 29 Hydrogen bonding in biological structure. This example is a portion of a protein in an -helical conformation. The -helix, a common structural element in proteins, is maintained by N-H……O=C hydrogen bonds between groups in the protein chain. 30 Forces are involved in biomolecular structures Sometimes relatively weak forces play an important role in biomolecular structures. Why? Because there are many of them! 31 The Role of Water in Biological Processes Hydrogen-bond donors and acceptors in water. The two nonbonded electron pairs on O act as H-bond acceptors and the two O-H bonds act as H-bond donors. The angle between the two O-H bonds is 104.5; thus, water has a net dipole moment. 32 The structure of liquid water. When ice melts, the regular tetrahedral lattice is broken, but substantial portions of it remain, especially at low temperatures. In liquid water, flickering clusters of molecules are held together by hydrogen bonds that continually break and re-form. In this schematic “motion picture,” successive frames represent changes occurring in picoseconds (10-12 s). 33 The Role of Water in Biological Processes 34 The Role of Water in Biological Processes Water serves as the universal intracellular and extracellular medium, thanks primarily to two properties: ability to form hydrogen bonds polar character Substances that can take advantage of these properties so as to readily dissolve in water are called hydrophilic, or “water loving.” 35 The Role of Water in Biological Processes Water is an excellent solvent for ionic compounds. The interactions of the negative ends of the water dipoles with cations and the positive ends with anions in aqueous solution cause the ions to become hydrated, that is, surrounded by shells of water molecules called hydration shells. The dissolution of ionic compounds like NaCl in water can be accounted for largely by two factors. o The formation of hydration shells is energetically favorable. o The high dielectric constant of water screens and decreases the electrostatic force between oppositely charged ions that would otherwise pull them back together. 36 The Role of Water in Biological Processes Hydration of ions in solution. A salt crystal is shown dissolving in water. As sodium and chloride ions leave the crystal, the noncovalent interaction between these ions and the dipolar water molecules produces a hydration shell around each ion. The energy released in this interaction helps overcome the charge–charge interactions stabilizing the crystal. The Role of Water in Biological Processes 37 Nonpolar substances like hydrocarbons, are nonionic and cannot form hydrogen bonds, show only limited solubility in water. Such nonpolar molecules are called hydrophobic, or “water fearing.” We can also call them lipophilic, or “fat loving.” When hydrophobic molecules do dissolve, they are not surrounded by hydration shells, rather the regular water lattice forms ice-like clathrate structures, or “cages,” about the nonpolar molecules. This ordering of water molecules extends well beyond the cage, corresponds to a decrease in the entropy, or randomness, of the mixture. This hydrophobic effect plays a role in protein folding. 38 Ionic Equilibria The Brønsted-Lowry definition of acids and bases is useful for the aqueous systems encountered in biochemistry. o acids are proton donors o bases are proton acceptors A strong acid dissociates almost completely into hydronium and a weak conjugate base. A strong base also ionizes entirely, releasing OH- ion, which is a powerful proton acceptor. 39 Ionic Equilibria 40 41 Ionic Equilibria Buffered solutions are able to minimize the change in pH following addition of acid or base because the conjugate acid (HA) and conjugate base (A-) of the buffering compound are present in sufficient concentration to combine with the added H+ or OH- and neutralize them. Buffer solutions function because the pH of a weak acid–base solution is least sensitive to added acid or base near the pKa, where the conjugate acid and conjugate base of the buffer are both present in nearly equimolar concentrations. 42 Ionic Equilibria Organisms must maintain the pH inside cells and in most bodily fluids within the narrow pH range of about 6.5 to 8.0. o The normal pH of human is 7.4. The dihydrogen phosphate–hydrogen phosphate system, with a pKa of 6.86, plays a major role in controlling intracellular pH because phosphate is abundant in cells. Blood contains dissolved CO2 as a waste product of metabolism, therefore the carbonic acid–bicarbonate system provides considerable buffering capacity. 43 The Energetics of Life 44 Energy, Heat, and Work Bioenergetics describes how organisms capture, transform, store, and utilize energy. A system is any part of the universe that we choose for study. o a single bacterial cell o a Petri dish containing nutrient and millions of cells o the whole laboratory in which this dish rests o the earth o the entire universe A system may be isolated, open, or closed The surroundings is anything not defined as part of the system. 45 Energy, Heat, and Work A system may be isolated, open, or closed Should we consider human as a isolated, open, or closed system? 46 Energy, Heat, and Work A system may be isolated, open, or closed Should we consider organism an isolated, open, or closed system? An open system can exchange matter and energy through its boundary. A closed system can exchange energy but NOT matter through its boundary. An isolated system can exchange neither energy nor matter through its boundary. 47 Energy, Heat, and Work The internal energy (U) of a system includes all forms of energy that can be exchanged via simple (nonnuclear) physical processes or chemical reactions. The thermodynamic state is defined by describing the amounts of all substances present and any two of the following three variables: o the temperature (T) o the pressure on the system (P) o the volume of the system (V) 48 Energy, Heat, and Work Unless a system is isolated, it can exchange energy with its surroundings and thereby change its internal energy; we define this change as U. For a closed system, this exchange can happen in only two ways. 1. Heat (q) may be transferred to or from the system. 1. The system may do work (w) on its surroundings or have work done on it. 49 Energy, Heat, and Work Because the internal energy of a closed system can change only by heat or work exchanges with the surroundings, the change in internal energy must be given by U = q – w This equation expresses the first law of thermodynamics. Energy is conserved. According to the first law of thermodynamics, in a closed system, the internal energy (U) can change only by the exchange of heat or work with the surroundings; however, energy can be converted from one form to another. 50 Energy, Heat, and Work To express the heat change in a constant-pressure reaction, we need another function of state. We define a new quantity, the enthalpy (H) as H = U + PV or H = U + P V When the heat of a reaction is measured at constant pressure, it is H that is determined. 51 Entropy and the Second Law of Thermodynamics Reversible processes occur always near a state of equilibrium; irreversible processes drive toward equilibrium. The second law of thermodynamics tells us which processes are thermodynamically favorable (or spontaneous). To present the second law, we must consider a new concept— entropy. 52 Entropy and the Second Law of Thermodynamics The degree of randomness or disorder of a system is measured by a function of state called the entropy (S). The second law of thermodynamics states that the entropy of an isolated system will tend to increase to a maximum value. Diffusion as an entropy-driven process. 53 Entropy and the Second Law of Thermodynamics 54 Free Energy: The Second Law in Open Systems The form of the second law as stated in the previous section is not very useful to biologists or biochemists because we never deal with isolated systems. Because living systems can exchange energy with their surroundings, both energy and entropy changes occur. Both energy and entropy must be of importance in determining the direction of thermodynamically favorable processes. The Gibbs free energy (G) or simply free energy is such a function of state that includes both energy and entropy. 55 Free Energy: The Second Law in Open Systems The Gibbs free energy is defined as G = H -TS where T is the absolute temperature measured in kelvin For a free energy change in a system at constant temperature and pressure, we can write G = H -TS Why is the quantity called free energy? The reason is that represents the portion of an energy change that is available, or free, to do useful work. 56 Free Energy: The Second Law in Open Systems 57 Free Energy: The Second Law in Open Systems 58 Free Energy: The Second Law in Open Systems 59 Free Energy: The Second Law in Open Systems 60 Free Energy: The Second Law in Open Systems A thermodynamically favored process tends in the direction that minimizes free energy (results in a negative G); this is one way of stating the second law of thermodynamics. o Processes with a negative free energy changes (-G) are exergonic o Processes with a positive free energy changes (+G) are endergonic 61 Free Energy: The Second Law in Open Systems Two considerations about the Gibbs Free Energy: 1.Favorability of a process has nothing to do with its rate. o Favorable processes are not necessarily rapid. o A catalyst may increase the rate for some reactions, but the favored direction is always dictated by G and is independent of whether or not the reaction is catalyzed. o Protein catalysts called enzymes selectively increase the rates for specific thermodynamically favorable reactions. The entropy of an open system can decrease. 62 Free Energy: The Second Law in Open Systems Contribution of enthalpy and entropy to several favorable processes. 63 Free Energy: The Second Law in Open Systems Life involves a temporary decrease in entropy, paid for by the expenditure of energy. The folding of proteins is well-described by G = H -TS, where H is a measure of the change in bonding interactions and S is a measure of the change in order of the system going from the unfolded state to the folded state. The free energy change, G, is a measure of the maximum useful work obtainable from any reaction. 64 Free Energy: The Second Law in Open Systems Free Energy and Chemical reactions: 65 Chemical Equilibrium Characteristics of the living state include the capture, transformation, storage, and utilization of energy Such processes can only occur when G < 0. A living organism is not at equilibrium. Since life occurs within relatively narrow ranges of temperature, pH, and concentrations for ions and metabolites, this set of conditions is referred to as homeostasis or the homeostatic condition. Because the concentrations of certain solutes inside cells remain relatively constant, homeostasis is frequently confused with true thermodynamic equilibrium. Homeostasis must not be confused with equilibrium! (organism – homeostasis yes, equilibrium no) High Energy Phosphate Compounds: 66 Free Energy Sources in Biological Systems Coupling of endergonic reactions or processes to exergonic reactions High Energy Phosphate Compounds: 67 Free Energy Sources in Biological Systems Coupling of endergonic reactions or processes to exergonic reactions is used not only to drive countless reactions but also to transport materials across membranes transmit nerve impulses contract muscles carry out other physical changes High Energy Phosphate Compounds: 68 Free Energy Sources in Biological Systems Driving an unfavorable process by coupling it to a favorable one requires the availability in cells of compounds that can undergo reactions with large negative free energy changes. Such substances can be thought of as energy transducers in the cell. The most important of these high energy compounds are certain phosphates, which can undergo hydrolytic release of their phosphate groups in aqueous solution. “High-energy” phosphate compounds have very large negative free energies of hydrolysis. High Energy Phosphate Compounds: 69 Free Energy Sources in Biological Systems Hydrolysis reactions for some biochemically important phosphate compounds. High Energy Phosphate Compounds: 70 Free Energy Sources in Biological Systems Hydrolysis reactions for some biochemically important phosphate compounds. High Energy Phosphate Compounds: 71 Free Energy Sources in Biological Systems High Energy Phosphate Compounds: 72 Free Energy Sources in Biological Systems The ATP molecule and its hydrolysis reactions. High Energy Phosphate Compounds: 73 Free Energy Sources in Biological Systems The orthophosphate ion (HPO4-2) which is often abbreviated as Pi (inorganic phosphate), is capable of a wide variety of resonance forms. Consequently, release of the orthophosphate results in an entropy increase in the system and is therefore favored. Resonance stabilization applies in all of the phosphate hydrolysis reactions shown previously.