Adsorption on Alternative Low-Cost Materials-Derived Adsorbents in Water Treatment PDF
Document Details
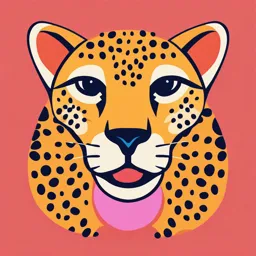
Uploaded by LushTonalism1394
Wojciech Stawiński and Katarzyna Wal
Tags
Summary
This article examines the use of low-cost adsorbents for water treatment, specifically focusing on materials derived from agricultural waste. The authors discuss the advantages and disadvantages of using these materials and explore their potential application in various water treatment methods. The paper covers different materials like bark, feathers, husks, and more.
Full Transcript
2 Adsorption on Alternative Low-Cost Materials-Derived Adsorbents in Water Treatment Wojciech S...
2 Adsorption on Alternative Low-Cost Materials-Derived Adsorbents in Water Treatment Wojciech Stawiński1* and Katarzyna Wal1,2 Łukasiewicz Research Network—Port Polish Center for Technology 1 Development, Wrocław, Poland 2 Department of Polymer and Carbonaceous Materials, Faculty of Chemistry, Wrocław University of Science and Technology, Wrocław, Poland Abstract The world has been facing a severe problem concerning water quality deteriora- tion due to anthropogenic activities. Among many water treatment techniques, adsorption is one of the most promising methods owing to advantages such as relatively low cost, versatility, effectiveness and simplicity. The most widely used adsorbents are based on activated carbon, however such are expensive and their production is burdened with big environmental footprint. Consequently, there is a need of finding new, more sustainable solutions for abatement of water pollut- ants. Low-cost, alternative materials-based adsorbents such as bark, feather, husks, leaves, peels, rinds, seeds, stones and spent coffee or tea, derived from waste gen- erated by various industries, may find application in the field. Nonetheless, there is still a substantial amount of issues connected with the materials’ stability and the logistics that impede their wide application and still require investigation. Keywords: Low-cost adsorbents, adsorption, water treatment, agricultural waste, alternative adsorbents, water pollution *Corresponding author: [email protected] Inamuddin, Mohd Imran Ahamed, Rajender Boddula, and Tauseef Ahmad Rangreez (eds.) Applied Water Science Volume 2: Remediation Technologies, (49–106) © 2021 Scrivener Publishing LLC 49 50 Applied Water Science Volume 2 2.1 Introduction It is an unquestionable axiom that the world, in the form we know, relies on water. It is a crucial and essential element for functioning of the Earth’s ecosystems and for survival of life on our planet. Nevertheless, the water resources have been suffering from severe pollution generated by anthro- pogenic activities. The quality of drinkable water, that constitutes only 1% of all the accessible water in the world, has been gradually deteriorating [1,2]. Access to uncontaminated water is already limited for many people and with the 30% population growth prognosis by the year 2050, the situ- ation is going to get worse if some preventive actions are not undertaken [2–5]. There are numerous contaminant types that find their way daily to water curses and water bodies. On their forefront are dyes [6,7], pharmaceuticals and personal care products [8,9], heavy metal cations [10,11] and pesti- cides. Some of them are cancerogenic or toxic even in low concentra- tion, other can bioacculumate in living organisms, change the organism’s metabolism or cause genetic mutations. Alarming is the fact that the adverse effects of some contaminants have not been yet fully determined, especially in the long-term aspect. Also there is almost no research on the synergic effect they can exhibit on organisms when occurring accompa- nied with other potentially harmful substances in water. 2.2 Water Treatment Water treatment is a process in which wastewater achieve the quality stan- dards demanded by regulations. The methods are classified as phys- ical, chemical, electrical, thermal or biological [11,14]. Although some are effective, they may have technical and economic challenges limiting their applications [3,4]. Biological treatment bears the problem of micro- organism stability that can be upset upon contact with toxic compounds. Reverse osmosis or nano and ultrafiltration are high energy consuming, the membranes are expensive and need to be regularly replaced [15–17]. Coagulation or flocculation removes the contaminants only partially and creates expensive to manage, toxic sludge [15–17]. Treatment based on phytoremediation treatment is time-consuming and often requires chelat- ing agents to increase the accumulation in plants. Ion exchange may be extremely costly as the exchange resins are expensive. Moreover, the methods need to be used in combination to remove all [3,4,6]. Low-Cost Alternative Adsorbents 51 Development of a cost-effective and efficient wastewater treatment method is still a challenge [6,11]. 2.3 Adsorption Among all the methods, there is one that stands out—the absorption. It is a process basing on interactions of the contaminants (adsorbates) with the sur- face of a solid material (adsorbent) via physical forces or by creating chem- ical bonds. This technique is simple, low-cost and universal, the removal efficiency is high and no advanced technological lines are required for the implementation [7,14,19]. Activated carbon in the most widely used adsor- bent, however it has one crucial disadvantage—its production is expensive and leaves a big environmental footprint. The yield from the carbonization process may be as low as 5–10% and it consumes a lot of energy as it requires high temperatures. Moreover, the regeneration is also pricy and significantly diminishes the material’s adsorption capacity [20–22]. Consequently, there is a constants search for other material that could be applied in the field. 2.4 Application of Low-Cost Waste-Based Adsorbents in Water Treatment This chapter is focused on application of natural materials, mostly waste generated by various industries, in water treatment. Although it is very common to subject that kind of raw materials to thermal treatment or to treatment with concentrated acids, both resulting in formation of activated carbons, such modifications are excluded from the chapter’s scope. The aim was to present the materials’ potential in their raw form or subjected to mild chemical or physical treatment not resulting in their carbonization. Many material groups have been broadly described with a special focus on the last decade. Although distinguishing between a leaf and a stem might me straightforward, a rind and a peel might cause a bit of concerns. Stone or the pit, more technically the endocarp, the inner most part of the fruit pericarp (without the endocarp layer, the pericarp is usually the edible part). Stone is the hard layer protecting the seed localized inside it. Stones are quite often confused with seeds or the other way around, or not dis- tinguished at all. Botany is obviously not the expertise filed of the Authors and not the topic to be discussed in the chapter, hence the classification is presented following the nomenclature used in the analyzed articles. 52 Applied Water Science Volume 2 2.4.1 Bark Bark is a by-product generated by the forest industry. Similarly to the other raw materials, bark is usually dried in temperature below 100 °C, ground and a determined fraction selected for experiments. Barks generally con- tain cellulose, hemicellulose and lignin, and several functional groups in their structure: carbonyl, carboxylic, amine, hydroxyl, sulfonic occurring in aromatic and aliphatic compounds including aldehydes, esters, ketones, carboxylic acids and phenols. Eucalyptus bark and pine bark are among the most widely investigated materials in this group. The adsorption capacities of the examples are given in Table 2.1. 2.4.1.1 Eucalyptus Eucalyptus bark contains very high amount of carboxylic and pheno- lic groups. These groups are known for their affinity for U6+. The bark’s adsorption properties for that metal cation were intensely studied in a col- umn system. The authors investigated the influence of many variables on the performance and proved it to be a promising material for that pur- pose. Similar good results were obtained in the case of Bisphenol A. The bark was additionally modified with Fe2O3 magnietic nanoparticles to form a hybrid of enhanced adsorption capacity and to facilitate the mate- rial separation from the solution. The adsorption process reached the equi- librium in approximately 60 min and the optimal condition were in pH 7. In the case of Hg2+ removal on eucalyptus bark, the optimum condi- tion were in pH 6, and the process was significantly faster than Bisphenol A adsorption, reaching the equilibrium in below 10 min. The solution salin- ity strongly influenced the adsorption which efficiency decreased when there was more NaCl in the tested systems. 2.4.1.2 Pine Pine bark has been also widely investigated as an adsorbent. Among the substances, of which removal was tested on the bark, were three common antibiotics: tetracycline, oxytetracycline and chlortetracycline. The tests were performed in a single and ternary system to investigate the materi- als affinity for each adsorbate. The bark exhibited the highest affinity for chlortetracycline and the lowest for tetracycline. Pine bark was also applied for removal of a dye Congo Red. The equilibrium was reach in 100 min and the material worked the best in pH 2. The bark modi- fied with H2O2, H2SO4 and NaOH was tested for adsorption towards Cd2+, Low-Cost Alternative Adsorbents 53 Table 2.1 Application examples of bark-derived adsorbents in removal of pollutants from water. Adsorbate and adsorption Adsorbent capacity (mg g−1) Reference Araucaria bark Crystal Violet 47.32 Argentine cedar Red-97 422.87 Brazilian pine bark Gentian Violet 305.3 Cedar bark Cu2+ 14.23 Cinnamon bark Indigo Carmine 1.61, Malachite Green 8.95 Coconut tree bark Methylene Blue 14.73 Cork bark Methylene Blue 30.52 Douglas fir-oxidized and Cs+ 200.68 impregnated Eucalyptus bark Hg2+ 73.64 Eucalyptus bark U6+ 3 Eucalyptus bark-Fe2O3 Bisphenol A 290.6 Old World sycamore bark Ni2+, Cr6+ Old World sycamore 126.58, 13.42 bark-HNO3 285.71, 19.92 Pine bark Tetracycline 3.27, oxytetracycline 3.57 chlortetracycline 3.66 Pine bark Congo Red 3.92 Pine bark Cd2+, Pb2+, Cr3+ Pine bark-H2O2 10.83, 12.42, 10.66 Pine bark-H2SO4 8.37, 13.27, 3.77 Pine bark-NaOH 17.59, 16.83, 10.06 26.08, 44.66, 18.35 Pine bark Cu2+, Cd2+ Pine bark-HCl 2.89, 2.84 Pine bark-NaOH 2.80, 2.64 3.44, 3.18 (Continued) 54 Applied Water Science Volume 2 Table 2.1 Application examples of bark-derived adsorbents in removal of pollutants from water. (Continued) Adsorbate and adsorption Adsorbent capacity (mg g−1) Reference Pine bark-quaternary V5+ 34.64 ammonium salt Pine bark-TNT-degrading 2,4,6-trinitrotoluen (TNT) bacteria the adsorption capacity not applicable Pineapple bark Methylene Blue, Brilliant Green, Congo Red, 60 Silver fir Cu2+ 7.80 Soap nut bark Cu2+ 37.07 True sago palm bark Cr6+ 61.73 Pb2+ and Cr3+. The treatments did not result in an increase of the bark rel- atively low specific surface area of 0.5 m2 g−1. However, some prominent changes in the surface morphology appeared and included pits, cracks and fissures. The acid and base modifications generally increased the material’s adsorption capacity, unlike the peroxide treatment. The authors indicated chemisorption as the Cr3+ removal mechanism and physisorp- tion as the removal driving force for Pb2+ and Cd2+. The assumption was based on the regeneration studies where, unlike Cr3+, Pb2+ and Cd2+ were easily desorbed with HCl form the saturated. Very interesting study was conducted on the adsorption of TNT (2,4,6-trinitrotoluen) in a hybrid system containing pine bark and TNT-degrading bacteria. The bark provided a growth support for the bacteria and aced as an adsorbent for the metabolites released during the TNT biodegradation. Pine bark modified with NaOH and a quaternary ammonium salt was used as an adsorbent for V5+. The equilibrium, in optimal pH 2, was reached in 24 h and the influence of temperature was neglectable. The regenera- tion studies showed the possibility of efficient (80%) V5+ desorption with NaOH that worked in 3 cycles. Pine bark, raw, treated with HCl, and with NaOH, was tested for Cu2+ and Cd2+ adsorption in a single and binary system. The alkali treated material exhibited the highest adsorp- tion capacity for both cations and the affinity for Cu2+ was higher than for Cd2+ in all cases. Low-Cost Alternative Adsorbents 55 2.4.1.3 Other The bark of araucaria was used as an adsorbent for Crystal Violet dye. The adsorption was strongly temperature-dependent and different dye aggre- gation systems were observed depending on the pH. The removal was the best in pH 8. Argentine cedar (Cedrella fissilis) bark was evaluated for adsorption of a dye Red 97. The process attained the equilibrium in 2 h in optimal pH equal 2. Moreover, the material was used for a model effluent containing additionally Methylene Blue, Malachite Green, Ponceau Red dyes, and NaCl and Na2CO3. The removal reached the level of 87%. Cedar bark was used for Cu2+ adsorption. The metal cation was removed most efficiently in pH ranging from 5 to 6, the equilibrium was reached in approximately 4 h. Cinnamon bark was applied for removal of two dyes: Indigo Carmine and Malachite Green. The optimal conditions for the first dye were established in pH 2, with equilibrium time of 90 min. The adsorption of the second one was optimal in pH 7 reaching the equi- librium within similar time. There is quite a few other examples where bark was applied in water remediation: Brazilian pine bark for Gentian Violet dye, with HNO3 regeneration ; pineapple bark for Methylene Blue, Brilliant Green, and Congo Red in a ternary mixture ; Douglas fir bark oxidized by the Fenton reaction or by 2,2,6,6-tetramethylpiperidine-1-oxy radical- mediated oxidation and impregnation of nickel (II) hexacyanoferrate (III) for Cs+ ; silver fir and soap nut for adsorption of Cu2+; Old World sycamore (Platanus orientalis) bark, raw and modified with HNO3, for abatement of Ni2+ and Cr6+ ; true sago palm bark for Cr6+ with regeneration with HNO3 ; coconut tree bark or cork tree bark for Methylene Blue. 2.4.2 Coffee Global production in 2017 reached 9.2 · 106 tons. Common method of coffee grounds pretreatment is washing with hot water until no more brown color is present in the solution. The grounds are then usually dried in temperature close to 105 °C and ground or smashed to obtain desired particles fraction. The adsorption capacities of the examples are given in Table 2.2. The removal of Ni2+ and Zn2+ was studied in coffee grounds coated with zero-valent iron nanoparticles. The adsorption on such material was around two times higher than on its raw counterpart. The coating increased the material’s specific surface area and provided new adsorption centra. 56 Applied Water Science Volume 2 Table 2.2 Application examples of spent coffee-derived adsorbents in removal of pollutants from water. Adsorbate and adsorption Adsorbent capacity (mg g−1) Reference Coffee-Fe0 Zn2+ Ni2+ Pb2+ 164.1 Cd2+ 112.5 As3+ 23.5 As5+ 9.3 Coffee-eggshells-sericite Pb2+ 155.67 Alginate–coffee Ni2+ 20.96, Cd2+ 91.18 Coffee Ni2+ 7.81, Cd2+ 12.75 Coffee–chitosan Pb2+ 163.51 Coffee Cd2+ 1.32 Washed coffee Ni2+ 1.56, Zn2+ 1.3, Cd2+ 2.68 Unwashed coffee Ni2+ 1.76, Zn2+ 1.43, Zn2+ 4.34 Coffee-HNO3, degreased Fe2+ 0.615 Coffee-attapulgite clay complex Pb2+ 6.98 Coffee-Fe3O4-polyvinyl alcohol Pb2+ 56.98 Coffee-HCl F- 9.05, Pb2+ 61.6 Coffee chitosan polyvinyl Acetylsalicylic acid alcohol composite 9.92, Caffeine 8.21, Acetaminophen 7.25, Metamizol 6.29 Coffee-polypyrrole Rhodamine B 50.59 Coffee powder Rhodamine B 2.50, Rhodamine 6G 8.08 Coffee modified with: Methyl orange cetyltrimethyl ammonium 58.82 bromide 62.50 cetylpyridinium chloride Coffee-H2SO4 Methylene Blue 812, Tetracycline 462, Cr6+ 302 Coffee 4-chloro-2-methyl phenoxy acetic acid pesticide 340 Low-Cost Alternative Adsorbents 57 Similar good results were obtained for Pb2+, Cd2+, As3+ and As5+ removal. The coffee grounds served as supporting medium for the nanoparticles preventing their agglomeration. The grounds had negative net surface charge whilst the iron nanoparticles positive charge. This resulted in strong interaction between the two and good dispersion of the iron spe- cies on the grounds surface. A hybrid material composed of coffee grounds, eggshells and sericite (natural mineral) was used for remediation of Pb2+. The adsorbent had many OH groups that took the major action in the removal. Another coffee grounds-based adsorbent containing calcium alginate was tested for removal of Ni2+ and Cd2+. The adsorption capacity was significantly increased for of both cations owing the pres- ence of phenolic groups in the grounds and their high affinity for metal cations. Additionally, such adsorbent was formed in beads to facilitate its separation from the solution. Another kind of beads based on spent coffee and chitosan were used for Pb2+ removal. The material was prepared by mixing the coffee with chitosan acetate solution, pouring the mixture dropwise to a coagulant solution and then crosslinking the polymer. Ultrasonic treatment of spent coffee reduced the grain size what resulted in increased adsorption capacity for Cd2+ providing a good alternative for the grinding process. Spent coffee adsorbent was also applied for remedi- ation of model mine wastewater laden with Zn2+, Ni2+ and Cd2+. The results demonstrated that washing, commonly applied to that kind of material, may decrease its adsorption capacity. Such adsorbent had different affinity for the tested cations with the greatest for Zn2+. The divalent ions pres- ent in the solution hindered the adsorption the monovalent ones. Coffee grounds activated in HNO3 and degreased in ether solvent were applied for adsorption of Fe2+ from water. The adsorbent removed more cations that the activated carbon used in the study. Coffee grounds were combined with a clay mineral attapulgite to form a complex on the hydrothermal reaction way. This material was successfully used for Pb2+ removal. The same metal cation was adsorbed on coffee grounds com- bined with magnetic Fe3O4 nanoparticles coated with and polyvinyl alco- hol. Spent coffee grounds activated with HCl were used for removal of F- and Pb2+. The adsorbent was subjected to regeneration with HNO3 in the case of Pb2+ and NaOH in the case of F-. A slight decrease upon two adsorption-regeneration cycles was observed (12 and 18 % for Pb2+ and F-, respectively), however the deterioration was less significant between the second and the fifth cycle. Spend coffee washed with hexane and eth- anol were combined with chitosan and polyvinyl alcohol to obtain a com- posite and applied for removal of pharmaceuticals. The composite with 5 wt-% coffee exhibited adsorption capacity increased up to 50% for selected 58 Applied Water Science Volume 2 pharmaceuticals. The removal was strongly influenced by the pH and was the greatest in slightly acidic conditions (pH 6). The adsorption was also selective with the greatest affinity for acetylsalicylic acid followed by caf- feine, acetaminophen and metamizol. Another composite material obtained by combining spent coffee with semi-conducting polymer poly- pyrrole. The coffee had been rinsed subsequently with NaOH and HCl to extract soluble elements and was applied for Rhodamine B removal. The adsorption was enhanced in alkaline pH and the material was more stable than the coffee grounds. Rhodamine B and 6G were also removed on an unmodified spent coffee grounds, however with significantly lower efficiency than on the composite. The removal of Rhodamine B was almost 20 times lower compared Rhodamine 6G. Two cationic surfactants, cetyltrimethyl ammonium bromide and cetylpyridinium chloride were used to enhance adsorption capacity of coffee waste towards an organic dye Methyl Orange. The removal was the greatest in acidic pH (3.5) and was slightly (6%) better when cetylpyridinium chloride was applied for the modification. Spent coffee sulfonated with H2SO4 acid was applied for removal of a dye Methylene Blue, an antibiotic tetracycline and Cr6+. Studies on removal of a pesticide 4-chloro-2-methyl phenoxy acetic acid on spent coffee grounds found that the pH did not significantly influenced the adsorption capacity (investigated in the range of 2–8) and the carboxyl and hydroxyl functional groups were the main contributor to the adsorp- tion process. 2.4.3 Feather Feathers have also found their application in water remediation. This mate- rial is mostly built of keratin (around 85%) regardless the bird species, beside that there is also water, lipids and some metals such as magnesium or calcium. The active adsorption sites present in the feather’s structure are mainly the functional groups of amides and the cysteic acid and in the peptide bonds (C=O, C–N, N–H). The feathers are usually pretreated with H2O2, ethanol or a detergent to remove any impurities and contami- nants from their structure. The adsorption capacities of the examples are given in Table 2.3. Ostrich feathers, raw and treated with H2O2 were used for adsorption of phenol. The adsorption was strongly pH-dependent, favored in lower range, and it had very long equilibrium time of 24 h. A dye Reactive Yellow 39 was removed on hen feather. The adsorbent was only 30% less effective than the granular activated carbon used for the comparison. Hen feathers were also applied in removal of another dye Bismark Brown R. Low-Cost Alternative Adsorbents 59 Table 2.3 Application examples of feathers and feathers-derived adsorbents in removal of pollutants from water. Adsorbate and adsorption Adsorbent capacity (mg g-1) Reference Duck feather-tannic acid Cu2+ 48.93 Zn2+ 62.11 Duck feather Pb2+ Duck feather-Na2S2O5-urea 6.11 10.98 Ostrich feather Phenol Ostrich feather H2O2 0.64 2.16 Hen feather Reactive Yellow-39 219 Bismark Brown R 360 Brilliant Yellow 93.6 Dark Blue Astrazon 2RN 47 ± 4 Amido Black 10B 12.95 Congo Red 73.85 Zn2+ 4.31 Ni2+ 1.74 Cd2+ 1.98 Pb2+ 8.49 Malachite Green 3.55 Hen feather–epichlorohydrin Cu2+ 3.06 Cr6+ 9.62 Hen feather–ethylenediamine Cu2+ 6.95 Hen feather–glyceryn film Cr6+ 75.45 Hen feather–chitosan Pb2+ 70.42 Here the adsorption was also more intense in lower pH (3.0) but the equi- librium was reached within 2 h. Brilliant Yellow dye was similarly adsorbed onto hen feathers as well as Dark Blue Astrazon 2RN with the adsorption optimum in pH 9 , Amido Black 10B Congo Red and finally Malachite Green. 60 Applied Water Science Volume 2 Heavy metal cations have also been adsorbed on feather-based materials. The adsorption of Pb2+ was studied in a dynamic system on melt-blows polypropylene filter cartridges prepared with duck feather pretreated with Na2O2O5 and urea. The adsorption capacity of the mod- ified material increased roughly by 80%. Additionally the material was regenerated with Na2S·9H2O and NaOH. Investigation on simulta- neous removal of Cd2+, Ni2+ and Pb2+ on hen feathers indicated that the adsorption was a complicated antagonistic process: the affinity for Pb2+ was the strongest, and the presence of these ions hindered adsorption of the other; the effect was the most visible in pH 5. The material was feasible to regenerate with diluted acid solution. Batch and columns studies on removal of Zn2+ on hen feather revealed that the process was fast, occurred on the external material’s surface and was the most effec- tive also in pH 5. Duck feather modified with tannic acid was proved to be an effective adsorbent for Cu2+ and Zn2+. Modification of hen feather with NaOH to exfoliate the keratin filaments form the surface resulted in slightly increased adsorption capacity towards Cr6+. Further crosslinking of the exfoliated filaments with epichlorohydrin resulted in increased odsorption of the cation in low concentration but the adsorp- tion of Cu2+ was rather low. Finally when the product was functionalized with ethylenediamine it was able to adsorb efficiently Cd2+ from higher concentration range. Hen feathers and glycerol were formed under pressure and increased temperature into a thermoplastic keratin film and applied for Cr6+ adsorption. The material worked the best in neutral or slightly acidic environment and reached good removal rate. The feathers were also combined with chitosan to form a biopolymer com- posite and used for removal of Pb2+. 2.4.4 Husks or Hulls Husk or hull is the protective outer shell of a seed. The materials have also found their place among low-cost adsorbents. Generally the pretreatment methods are similar than in the case of the other materials and include washing, drying in mild temperature and grinding. Hulls are composed mainly of cellulose, hemicellulose, lignin, polysaccharides, proteins, lipids, and silica. The most important adsorption centra are localized on the car- bonyl, carboxyl, amino and hydroxyl groups. The adsorption capacities of the examples are given in Table 2.4. Low-Cost Alternative Adsorbents 61 Table 2.4 Application examples of husks and husk-derived adsorbents in removal of pollutants from water. Adsorbate and adsorption Adsorbent capacity (mg g−1) Reference Almond green hull Co2+ 45.5 Cr6+ 10.58 Green longan hull Ni2+ 3.96, Cd2+ 4.19 Lentil husk Cd2+ 107.31 Mustard husk Pb2+ 30.48, Cd2+ 42.85 Peanut husk Methylene Blue 72.13 Peanut husk Indosol Yellow BG Peanut husk-acetic acid 73.05 Peanut husk-Na-alginate 79.7 50.9 Peanut husk Reactive Black-5 55.55 Pistachio hull Methylene Blue 389 Cyanide 156.2 Rice husk Malachite Green 17.98 U6+ 11.86 Direct Red-31 129.87 Direct Orange-26 66.67 Tl3+ 42.87 Rice husk Co2+, Methylene Blue [83,84] Rice husk–NaOH 17.6, 52.2 Rice husk–supercritical not analysed, 65.0 Rice husk–ultrasounds 20.8, 56.4 35.0, 58.7 Rice husk-epichlorohydrin and NO3− 55.55 dimethylamine 62 Applied Water Science Volume 2 2.4.4.1 Peanut Peanut husks were investigated as an adsorbent for several pollutants. In batch and column experiments with Methylene Blue the equilibrium was 250 min. Adsorption of another dye Reactive Black 5 reached it in longer time of 380 min and the conditions were optimal in very low pH of 1. The husks modified with acetic acid, and immobilization in Na-alginate was tested for Indosol Yellow BG removal. In this case the equilibrium was attained in 45 min in pH 2. The first treatment enhanced the maximum adsorption capacity by 10%, whereas the immobilization decreased it by 70%, in relation to the raw husk. 2.4.4.2 Rice Rice husk modified with NaOH, was used for removal of Malachite Green. The adsorption process was more effective in pH range higher than 4, reaching the optimum in pH 7. The treatment resulted in formation of pores and cavities in the material. It also removed the silica and lignin from the husks exposing more OH groups acting as the main adsorption centra.. Rice husk modified by sonication, and treatment with CO2 in super- critical conditions was applied in removal of Co2+. No changes in the par- ticles size was observed upon the modification occurred, however the ash content and the point of zero change decreased due to the leaching of the silica and exposition of the organic fraction as the consequence. Changes were observed also in the specific surface that in both cases increased. This phenomenon occurred due to the cavitation happening during the ultrasound treatment and due to high pressure action in the case of the supercritical CO2 treatment. The optimal adsorption conditions were established in pH 5 with the equilibrium time of 300 min. Similar treatment regimen, extended for a NaOH modification, was applied to the husk used in Methylene Blue adsorption. The alkaline treatment caused raptures in the material’s surface and exposed the internal structure what provided more adsorption centra. Another modification of the husks was their treatment with epichlorohydrin and dimethylamine. Such mate- rial was used for adsorption of nitrates from water. The optimal pH for he presses was 7 and the equilibrium was reach in 90 min. The tests on real wastewater reveal the NO3− removal was strongly influenced by the pres- ence of other anions in the descending order of CO32− > Cl > PO24− > SO24−. Rice husks were also tested for removal U6+ , Direct Red 31 and Direct Orange 26 with 200 min equilibrium time and optimal condition in pH 2 , Tl3+ with 20 min equilibrium time in pH 10 , Cd2+ in pH 5 Low-Cost Alternative Adsorbents 63 and 60 min equilibrium. In the last study it was shown that Pb2+ had higher affinity than Cd2+ for the material. 2.4.4.3 Other Other plants which husks served as adsorbents were: almond green hulls treated with H2O2 and ammonia to extract soluble organic compounds for adsorption of Co2+. The modifications resulted in increased specific surface area what was reflected in very fast 1 min equilibrium time , unmod- ified almond green for Cr6+ in pH 2 and 60 min equilibrium time , green longan husks for removal of Ni2+ and Cd2+ in pH 5 and 20 min equi- librium time , mustard husk for Pb2+ in pH 4 and Cd2+ in pH 8 , pistachio hulls for Methylene Blue and cyanide in pH 8 and pH 10 8 and 70 and 60 min equilibrium time, respectively [94,95]. 2.4.5 Leaves Leaves of quite a lot of plant species were tested for removal of various substances form water. Leaves are usually pretreated as the rest of the material described in this chapter, third chemical composition is similar to the other material as well: cellulose, lignin and hemicellulose being the main components. The adsorption capacities of the examples are given in Table 2.5. Aloe vera leaves were used for adsorption of a dye Titan Yellow from wastewater. The material was characterized by specific surface area of 3.94 m2 g−1 and exhibited higher adsorption capacity than its counterpart trans- formed into activated carbon. Guava leaves were employed in phe- nol adsorption. The equilibrium was reached in 9 h and the material was subjected to regeneration with NaOH keeping 60% of its initial capacity after the 1 investigated cycle. Lesser bulrush leaves were tested for Methylene Blue removal. The optimal conditions were found in pH 10 and the equilibrium was reached in 60 min. Magnolia leaves were tested for Cr6+ removal. The material functioned the best in pH 2 and the equi- librium was achieved in 45 min. The regeneration studies showed that HNO3 was a better desorbing agent than HCl or EDTA. Pineapple leaves were modified with NaOH and iminodiacetic acid to be applied for Pb2+ removal. The adsorption kinetics on the NaOH-material had lower rate compared to the second adsorbent. The adsorption reached the equi- librium in 6 and 4 h, respectively. The latter material attained almost 2 times higher adsorption capacity than the first one. The capacity decreased around 13% after 4 regeneration cycles with HCl. Pomelo leaves were 64 Applied Water Science Volume 2 Table 2.5 Application examples of leaves and leave-derived adsorbents in removal of pollutants from water. Adsorbate and adsorption Adsorbent capacity (mg g−1) Reference Aloe vera leaves Titan Yellow 55.25 Ash tree leaves Cd2+, Cu2+, Pb2+ mix 51.2 Bay tree leaves Cd2+ 7.65, Pb2+ 31.25 Castor bean Engine oil 3.8 g/g Dead Neem Leaves Co2+ 476.12 Eucalyptus leaves As5+ 84.03, Hg2+ 129.87 Ficus leaves Cr6+ 56.82 Guava leaves Phenol 10.85 Lesser bulrush leaves Methylene Blue 106.76 Magnolia leaves Cr6+ 3.96 Malabar plum leaves F- 7.4 Olive leaves Cd2+ 36.6 Persimmon tree leaves Cu2+ 19.42, Pb2+ 22.59, Cd2+ 18.26 Pineapple leaves-NaOH Pb2+ Pineapple leaves– 15.81 iminodiacetic acid 32.55 Pomelo leaves Pb2+ 207.2 Prosopis cineraria leaves F- 11.52 Sugarcane leaves Pb2+ 14.06 Teak leaves Eosin Yellow 31.64 Terap leaves Rhodamine B 104.96 Water bamboo leaves Methylene Blue 54.17 Willow leaves Ni2+ 38.8, Cd2+ 29.9 Low-Cost Alternative Adsorbents 65 also used for adsorption of Pb2+. The adsorption process reached equilib- rium in 2.5 h and the removal was greater in higher pH range (4–6) com- pared to more acidic conditions. The material exhibited similar affinity for Cd2+, Co2+, Zn2+ and Ni2+, however it was stronger in the case of Cu2+, com- pared Pb2+. Terap leaves were used as an adsorbent for Rhodamine B. The adsorption was favored in low pH range (below 3) and the equilibrium was attained in approximately 3 h. Solution of HCl and NaOH were the most effective desorbing agents, however in the case of NaOH the adsorp- tion capacity decrease in each cycles was greater than for HCl. Other reports on application of leaves in water treatment include: ash tree leaves for remediation of Cd2+, Cu2+, Pb2+ from ternary mixture , bay tree leaves for removal of Cd2+ and Pb2+ , castor bean for engine oil spill , Dead Neem Leaves for Co2+ , eucalyptus leaves for As5+ and Hg2+ , ficus leaves for Cr6+ , olive leaves for Cd2+ removal , persimmon tree leaves for Cu2+, Cd2+ and Pb2+ , Prosopis cin- eraria and Malabar plum for F- , sugarcane leaves for Pb2+ , Teak leaves for Eosin Yellow removal or Water bamboo leaves from Methylene Blue or willow leaves for Ni2+ and Cd2+ adsorption. 2.4.6 Peels Peel are usually chopped or ground, washed in water and dried in tem- perature below 120 °C. There are various functional groups present within the their structure such as the carbonyl, carboxylic, carboxylate, hydroxyl, phenolic, amino or the sulfonic group. Compounds such as alcohols, poly- saccharides, aromatic amines, aliphatic compounds, ketones, carboxylic acids, lipids, vinyl compounds have been identified in various peels. The adsorption capacities of the examples are given in Table 2.6. 2.4.6.1 Banana Banana peel has been widely used for removal of various substances form contaminated water. In adsorption of Rhodamine B, banana peel modified with H3PO4 showed effective adsorption of the dye in pH 2. The modifica- tion oxidized the lignin present in the material forming more adsorption centra. Another two dyes, Reactive Black 5 and Congo Red, were adsorbed on the peel. The adsorption reaches the optimum also in low pH (3). Congo Red was easier to desorb from the material, the most effec- tive eluent for that purpose was NaOH. Reactive Black 5 dye was also adsorbed on banana peel but modified with HCHO and HCOOH. This treatment modified the amino groups on the material’s surface. 66 Applied Water Science Volume 2 Table 2.6 Application examples of banana peels and banana peel-derived adsorbents in removal of pollutants from water. Adsorbate and adsorption Adsorbent capacity (mg g−1) Reference Banana peel Reactive Black 5 49.2, Congo Red 164.6 Banana peel Methylene Blue 211.9, Orange G 20.9 Banana peel Salicilic acid 6.62, Benzoic acid 9.80 Banana peel Oil spill 5–7 (g/g) Banana peel U6+ 34.13, Th4+ 10.10 Banana peel 14 lanthnides, La2+ 38.8, Ga3+ 46.2 Banana peel Cd2+ 98.4 Banana peel Sr2+ 41.5 Banana peel-HCHO-HCOOH Reactive Black-5 211.8 Banana peel-H3PO4 Rhodamine B 9.52 The equilibrium was reached within 180 min and the optimal condition was in pH 3. The adsorbent was regenerated with NaOH and was able to keep high adsorption capacity in subsequent cycles. Methylene Blue and Orange G showed various affinity for banana peel. The adsorption of the first one was approximately 10 times greater than the latter and it was optimal in pH 6 whilst pH 2 was better for Orange G. The adsorption of salicylic and benzoic acids on banana peels was characterized with rel- atively long equilibrium times of 14 and 12 h, respectively. The peels were also tested on their application in cleaning gas and crude oil spills. The sorbent could be reused more than ten times until it attained 50% of the initial adsorption capacity. Metal cations have also been removed from wastewater on banana peels—radioactive U6+ and Th4+ from syn- thetic and real mine water. The adsorption of U6+ was around 25% higher in the real wastewater case; in contrast, Th4+ removal decreased approx- imately 4 times compared to the model wastewater. An extensive study on adsorption of lanthanides was conducted employing banana peel Low-Cost Alternative Adsorbents 67 as the adsorbent. Intense milling of the peels increased the cations removal level making the adsorbent good candidate for remediation of mine waters laden with lanthanides. Banana peel was also applied for removal of Cd2+ and Sr2+ where it was regenerated with HCl. 2.4.6.2 Citruses Orange peel has also found their place amount low-cost adsorbents. Such peel ground to powder and soaked in acetone (removal of the color). The adsorbent was characterized with porous honeycomb-like structure and applied for Cr6+ removal of. Orange peel modified with quaternary amine in a multistep procedure was used for removal of a day Reactive Red 120. The main functional groups involved in the removal were the hydroxyl and amine groups on the peel surface and the process reached maximum in pH 2. Successful desorption with 92% efficiency was attained with NaOH solution. Unripe orange peels were tested for elimination of naphthalene from water. The equilibrium was reached within 3 h and the optimal adsorption conditions were in pH 6. The characteriza- tion of a peel used for Reactive Gray BF-2R removal showed that the mate- rial was microporous with relatively low specific surface area of 2.14 m2 g−1. The adsorption was the most intense in pH 2 in which the material’s sur- face was positively charged. Peels of that fruit were also employed in water remediation from oil spills. The peel was ground and washed in iso- propanol, then magnetic Fe2O3 nanoparticles were deposited on its surface and finally the material was modified with polydimethylsiloxane (PDMS). It exhibited highly hydrophobic and superoleophilic properties and could adsorb diesel oil and engine oil up to 5 and 6 times its original mass. The material was easily regeneratable by ultrasonic washing and kept its initial capacity up to 8 cycles. Orange peels activated in HCl were used for removal of Cu2+, Mn2+ and Fe2+. The adsorption was the highest in pH 6 and increased upon the activation. Peel of another species from the Citrus group—the pomelo—was used for Methylene Blue and Cr3+ removal. The adsorption occurred in three stages: bulk diffusion, gradual adsorption and the equilibrium. The dye adsorption mechanism was electrostatic interactions and hydrogen bond- ing, whereas the metal cation adsorbed via electrostatic and n-d interac- tions (action between the electron donor atoms—the oxygen or nitrogen, and the acceptors—the free d orbitals of the metal ion). Methylene blue was also removed on pomelo peel, raw and modified with citrate. The modification increased the adsorption capacity roughly 2.5 times by intro- duction of carboxylic groups into the structure. Pomelo also served 68 Applied Water Science Volume 2 for wastewater remediation discharged from the nuclear industry. The raw peel and the peel modified in a long, multistep process involving soak- ing in methanol and acetic acid, stirring in isopropyl azodicarboxylic acid, boiling in oxaloacetate and freeze-drying. The modification increased the adsorption capacity for uranyl ions by 30%. Table 2.7 Application examples of citruses peels and citruses peel-derived adsorbents in removal of pollutants from water. Adsorbate and adsorption Adsorbent capacity (mg g−1) Reference Orange peel–acetone Cr 4.69 6+ Orange peel Cu2+ 4.80 Mn2+ 15.95 Fe 8.35 Orange peel–quaternary amine Reactive Red 344.8 Unripe orange peel Naphtalene ~1.5 Orange peel Reactive Gray BF-2R Orange peel–Fe2O3-PDMS Diesel and engine oil Pomelo peel Methylene Blue 218.5, Cr3+ 11.3 Pomelo peel Methylene Blue Pomelo peel-citrate 81.7 199.2 Pomelo peel UO2−2 Modified pomelo peel 31.276 42.733 Grapefruit peel Pb2+ 12.73 Grapefruit-NaOH Ciprofloxacin 566 Lemon peel Cd2+ Lemon peel-NaOH 20.83 35.71 Tangerine peel-HNO3 Cd2+ 0.58 Co2+ 2.68 Cr6+ 2.85 Cu2+ 2.57 Mn2+ 2.79 Ni2+ 2.66 Pb2+ 0.75 Zn2+ 0.98 Mix of citruses peels Zr2+ 68.48 Shaddock peels Methylene Blue 305.81 Low-Cost Alternative Adsorbents 69 Following the Citrus path; grapefruit peel was tested as a Pb2+ adsorbent. The process attained the optimum in pH range between 5.3 and 6.5 and was completed within 1.5h. Grapefruit peel modified with NaOH to cleavage of the esters present in the peel and generate –OH groups was applied for removal of ciprofloxacin. Several adsorption driving forces were involved in the process including electrostatic and hydrophobic inter- actions, hydrogen bonding, and π–π interactions. Alkali-treatment of a lemon peel increased its Cd2+ adsorption capacity approximately by 70%. The equilibrium on the raw peel was reached in 150 min, 60 min faster than on the modified material. Regeneration with HCl allowed to reuse the adsorbent in three cycles. Tangerine peel modified with HNO3 was used for removal of various heavy metal cations. The modifica- tion increased the material’s adsorption capacity most significantly in the case of Mn2+ (around 3 times); the least significant effect was on adsorption of Cu2+ (around 10% increase). A mix of three citruses (two orange species and a tangerine) was applied for Zr2+ removal. Shaddock peels were applied for abatement of Methylene Blue. The adsorption was favored in pH higher than 6, the equilibrium was reached in 300 min and the particle size did not have a significant effect on the adsorption (fraction from 1.4 mm to below 0.3 mm). The adsorption capacities of the examples are given in Table 2.7. 2.4.6.3 Garlic Garlic peels and their application in water treatment have also been the sub- ject of an intense study. In phenol removal, the optimal conditions were in pH 2 and the equilibrium time equal to 7h. The peels had porous and rough surface that became smooth upon phenol adsorption. Zirconium- loaded peels exhibited good adsorption properties for F-. The presence of nitrate and sulfate anions did not influence the adsorption. However, phos- phate anion hindered it slightly. Adsorption of Methylene Blue on gar- lic peels was also studied. In this case the adsorbent surface seemed rougher after adsorption; the specific surface area was around 0.5 m2 g1 and the mate- rial contained small mesoperes (8 nm). In adsorption of Direct Red 12B dye, the peels worked the best in pH 2 reaching the equilibrium in 25 min; ion exchange was indicated as the mechanism. Adsorption of Pb2+ on mercerized (treated with 5 M NaOH) garlic peels revealed that alkaline treatment may result in an increase in the material’s adsorption capacity like in the investigated case with two-fold increase. The pH influence was signif- icant up to pH 3, in higher values no further capacity increase was observed. The adsorption capacities of the examples are given in Table 2.8. 70 Applied Water Science Volume 2 Table 2.8 Application examples of garlic peels and garlic peel-derived adsorbents in removal of pollutants from water. Adsorbate and adsorption capacity Adsorbent (mg g−1) Reference Garlic peel Phenol 14.49 Methylene Blue 142.86 Pb2+ 209, Cu2+ 37, Ni2+ 32 Direct Red 12B 37.96 Garlic peel Pb2+ Garlic peel-NaOH 51.73 109.05 Garlic peel-Zr F- 20.9 2.4.6.4 Litchi Litchi peels were investigated on their application in Cr6+ removal in a batch and column system. The material had small mesopores (3 mm) and specific surface area of 1.2 m2 g−1, the highest removal was obtained in pH 3 reach- ing the equilibrium in 1 h. The adsorption was hindered by the presence of other metal cations in the descending order of Fe2+ > Ca2+ > Mg2+> K+ > Na+ and anions PO34− > SO24− > CO32− > NO32− > Cl −. The material exhibited good adsorption capacity up to the third regeneration cycle with HNO3. Adsorption of Cr6+ on litchi peels modified with mercaptoacetic acid was increased by 35% compared to the starting peels, however the capacity Table 2.9 Application examples of litchi peels and litchi peel-derived adsorbents in removal of pollutants from water. Adsorbate and adsorption Adsorbent capacity (mg g−1) Reference Litchi peels Cr6+ 23.56 Litchi peels Cr6+ Litchi peels–mercaptoacetic acid 7.05 9.55 Litchi peels–Fe2O3 Pb2+ 78.74 Litchi peels–Fe2O3 Amaranth dye 44.87 Low-Cost Alternative Adsorbents 71 of the unmodified material was around 3.5 times lower than of the peel in the study described above. In the first study the litchi was obtained from India, in the second from China. This shows how different the mate- rial can be depending on the origin place. Two magnetic adsorbents based on litchi peels and Fe2O3 were applied for adsorption of Pb2+ and Amaranth dye. In the first case the equilibrium was reached in 2 h in pH 6, in the second case in 3 h. The adsorbents were feasible to regenerate with acetone (80% efficiency) and ethanol (75 % efficiency), respectively. The adsorption capacities of the examples are given in Table 2.9. 2.4.6.5 Other There are many other application examples of various fruits and vege- tables peels in water treatment from a diverse spectrum of pollutants: breadnut peel for Malachite Green ; broad bean peels for Methylene Blue calabash peel for Reactive Red 195-A and Reactive Blue 222 ; cantaloupe peel for Cr6+ and Pb2+ ; Ceylon breadfruit fruit peels for Ni2+ ; cucumber peel for Cd2+ [157,158], Cu2+ and Pb2+ and Methylene Blue and Orange G ; dragon fruit peels for Methylene Blue ; durian peel for Methylene Blue and Crystal Violet ; eggplant peels for oil spills and Pb2+ ; Egyptian cucum- ber peel for Malachite Green ; green pea peels for Methylene Blue and Congo Red ; Grewia venusta peel for Indigo Carmine and Methyl Orange ; jackfruit peels for Cr3+ and Ni2+ and Cr6+ langsat peels for Ni2+ ; Malabar chestnut peel for Hg2+ ; mango peels for Cr3+ and Cr6+ ; melon peel for Methylene Blue ; pas- sion fruit peel for Eriochrome Black ; pineapple peel for Methylene Blue ; pokan peel for Pb2+ ; pomegranate peel for Cr6+ , Methylene Blue amd ciprofloxacin ; potato peels for Congo Red , Methylene Blue and Orange G and Emerald Green ; tarap peel for Brilliant Green or water chestnut (Trapa natans) peels for removal of Rhodamine B , Brilliant Green , Reactive Orange 122 and Pb2+. The adsorption capacities of the examples are given in Table 2.10. 2.4.7 Rinds Water melon rind exhausts the application of rinds almost entirely. The rind contains a substantial amount of pectin, carotenoids, proteins citrul- line and cellulose. These are rich with functional groups such as hydroxyl, amine, carboxylic, methyl, methoxy that may act as the adsorption centra. 72 Applied Water Science Volume 2 Table 2.10 Application examples of various peels and adsorbents derived from them in removal of pollutants from water. Adsorbate and adsorption Adsorbent capacity (mg g−1) Reference Breadnut peel Malachite Green 353 Broad bean peels Methylene Blue 192.7 Calabash peel Reactive Red-195A 245.5 Reactive Blue-222 242.4 Cantaloupe peel Cr6+ 166.25 Pb2+ 22.27 Ceylon breadfruit fruit peels Ni2+ 2.26 Cucumber peels Cd2+ 112; 107 [157,158] Cu2+ 88.50 Pb2+ 147.06 Methylene Blue 157.5, Orange G 37.8 Dragon fruit peels Methylene Blue 192.31 Durian peel Methylene Blue 235.80 Crystal Violet 527.64 Eggplant peels Oil, 90% the adsorbent weight Pb2+ 88.33 Egyption cucumber Malachite Green 70.21 Green pea peels Methylene Blue 163.94 Congo Red 16.4 Grewia venusta peel Indigo Carmine 188.68 Methyl Orange 5.74 Jackfruit peel Cr3+ 13.50 Ni2+ 12.03 Cr6+ 64.47 Langsat peels Ni2+ 10.1 Malabar chestnut Hg2+ 0.71 (Continued) Low-Cost Alternative Adsorbents 73 Table 2.10 Application examples of various peels and adsorbents derived from them in removal of pollutants from water. (Continued) Adsorbate and adsorption Adsorbent capacity (mg g−1) Reference Mango peels Cr3+ 98.04, Cr6+ 66.67 Melon peel Methylene Blue 333.33 Passion fruit peel Eriochrome Black 303 Pineapple peel Methylene Blue 17.12 Pokan peel Pb2+ 112.1 Pomegranate peel Cr6+ 400 Methylene Blue 200 Ciprofloxacin 1135 Potato peels Congo Red 6.9 Methylene Blue 107.2, Orange G 23.6 Emerald Green 2.61 Tarap peel Brilliant Green 174; 275 Water chestnut Rhodamine B 2.45 Brilliant Green 50.51 Reactive Orange-122 46.43 Pb2+ 77.09; 105.40; 123.82 The rinds are usually washed with water and dried in temperature below 100 °C and powdered. The adsorption capacities of the examples are given in Table 2.11. Watermelon rind was applied to removal of a Rhodamine B, Methylene Blue and Cristal Violet. The rind was previously activated in HCl to remove any metal cations naturally present in the material. The equilibrium was reached within 30 min; acetic acid was found to be the most effective desorbing agent for the regeneration. The rind activated in H3PO4 was used for removal of Bbrilliant Green. The modification increased the material’s adsorption capacity about two times. Also in this case acetic acid was the most efficient adsorbent regenerating agent. Similar rind 74 Applied Water Science Volume 2 Table 2.11 Application examples of rinds and rind-derived adsorbents in removal of pollutants from water. Adsorbate and adsorption Adsorbent capacity (mg g−1) Reference Durian rind La3+ 71, Y3+ 35 Mangosteen rind Zn2+ 4.55 Watermelon rind Methylene Blue 188.68 Methylene Blue 243 Ni2+ 35.3, Cd2+ 23.3 Pb2+ 55.0 Ni2+ 38.98 Watermelon rind-H3PO4 Methylene Blue 416.4 Watermelon rind-HCl Pb2+ 116.2 Cu2+ 39.2 Watermelon rind-HCl Methylene Blue 489.8, Cristal Violet 104.76, Rhodamine B 86.6 Watermelon rind Brilliant Green Watermelon rind-H3PO4 92.6 188.6 Watermelon rind-HNO3-clove Zn2+, Fe2+, Pb2+ modification was used in the case of Methylene Blue adsorption. Unmodified rind was also employed for Methylene Blue adsorption in batch and in column system with regeneration with acetic acid. Adsorption of heavy metal cations has also been studied on watermelon rind-based adsorbents. It was applied for adsorption of Ni2+ and Cd2+ in a single, binary and complex solution with Zn2+, Co2+ and Cu2+. The affin- ity for Ni2+ was greater than for Cd2+, the adsorption of both cations was hindered in the presence of the other suggesting the lack of specificity of the process. Hydrochloric acid-treated watermelon rind was used for Pb2+ and Cu2+ adsorption, the adsorbent was regenerated with the same acid. In this case, the presence of Cu2+ did not have significant influence on Pb2+ adsorption, however adsorption of Cu2+ decreased almost two times in the presence of Pb2+. Adsorption of Pb2+ in a column system was Low-Cost Alternative Adsorbents 75 also investigated on watermelon rind. The column was regenerated within 15 min using HCl solution. Similar results were obtained in a column system and Ni2+ adsorption. Very interesting case of combination of two natural materials was obtained by impregnating HNO3-activated watermelon rind with clove extract. Such material possessed antibacterial properties and was a good Zn2+, Fe2+ and Pb2+ adsorbent. Clearly watermelon rinds are the most investigated low-cost adsor- bent, however there were also attempts to test the properties of mango- steen and durian rinds. The first, activated in nitric acid was applied to Zn2+adsorption , the second to adsorption of La3+ and Y3+ from a binary solution. 2.4.8 Seeds Unlike many stones or pits, seeds contain proteins, polysaccharides lip- ids, tannins and flavonoids compounds providing various types of adsorp- tion active sites (amino, carboxyl, carbonyl, sulphotante, phosphate and hydroxyl groups). Moreover, some of them contain active antibacterial agents. The adsorption capacities of the examples are given in Table 2.12. Moringa oleifera (drumstick or horseradish tree) is a rapidly growing tree in arid tropics. It is cultivated for its medical values and the seeds find their application is nutrition and for oil production. In adsorption of an industrial dye Reactive Red 120, some interaction between the seed and shell resulted in increased adsorption capacity compared to each of the elements alone. The material was also effective in adsorption from low-concentration solutions. This feature is very important in some particular cases such as removal of pharmaceuticals or endocrine disrupt- ing compounds. The seeds obtained from oil extraction factory were also applied in removal of Congo Red and Dispersed red 60 dyes. Also in this case the materials were effective in low pollutant concentrations where the adsorption rate was higher compared to the higher concentrations. Removal of microcystin-LR, the most abundant and toxic cyanobacterial toxin, was studied on pristine seeds and the seeds activated in HCl. The treatment increased the material’s specific surface area and porosity what was reflected in 10% enhanced adsorption capacity of the modified mate- rial compared to its raw counterpart. Seeds of that tree were also used for remediation of Pb2+. Coriander (Coriandrum sativum) is a common spice. After it has been processed for extraction of its oleoresins, the spent material, called nutra- ceutical industrial coriander seed spent, may be applied as an adsorbent in water remediation. Industrial, chromium-containing dye Acid Black 52 76 Applied Water Science Volume 2 Table 2.12 Application examples of seeds-derived adsorbents in removal of pollutants from water. Adsorbate and adsorption Adsorbent capacity (mg g−1) Reference Black sesame seeds Cr6+ Black sesame seeds–chitosan 18.32 31.44 Cassia surattensis seeds Oil content Chia seeds Reactive Yellow B2R 70.95 Clary sage seed Basic Violet-16 19.80 Coriander seeds Acid Black-52 748.94 Guava seeds As5+ 4 F- 0.345 Jujube seeds Cd2+ 182.4 Cu2+ 259.0 Ni2+ 140.9 Mango seeds Cr6+ 18 ± 2 Moringa oleifera seeds Reactive Red-120 344.86 Moringa oleifera seeds Congo Red 170.0, Dispersed Red-60 196.8 Moringa oleifera seeds Microcystin-LR Moringa oleifera seeds-HCl 85.5 91.49 Moringa oleifera seeds Pb2+ Nerium oleander seeds Methylene Blue 280.2 Nigella sativa seeds -Fe2O3-Sn2O Methylene Blue 58.82 Nigella sativa seeds -Fe2O3-ZrO2 Methylene Blue 38.10, As5+ 1.01 Nigella sativa seeds -MnFe2O4 Methylene Blue 10.07 Nigella sativa seeds -MnO2 Methylene Blue 84.03 Nigiella stavia seeds -HCl Methylene Blue 73.53 Paeonia ostii seeds -NaOH Methylene Blue 368.2 (Continued) Low-Cost Alternative Adsorbents 77 Table 2.12 Application examples of seeds-derived adsorbents in removal of pollutants from water. (Continued) Adsorbate and adsorption Adsorbent capacity (mg g−1) Reference Papaya seeds Methylene Blue Papaya seeds Methylene Blue Papaya seeds, defatted 769.23 1250 Papaya seeds Methylene Blue 555.56 Pergularia tomentosa seeds Methylene Blue 145.3 Populus tremula seeds Methylene Blue 168.0 Psyllium seeds Reactive Orange-16 206.16 Tamarind seeds Cr6+ 29.7 Wormwood seeds Cr6+ 45 was adsorbed on this material. The adsorption was the most intensive in pH 2 and significantly decreased in higher pH range. Chia seeds (Salvia hispanica) are widely used for direct consumption and oil extraction over the world. The residue after the extraction was applied for removal of a dye Reactive Yellow B2R. Guava (Psidium guajava) is a common tropi- cal fruit. Its seeds were modified with aluminum and tested for As5+ and F- adsorption. The material was first treated with HCl and then with AlCl3. The Al adsorption centra served to form complexes with the adsorbates. The removal of As5+ had roughly 1.5 times higher rate than of F−. Black caraway (Nigella sativa) seeds find their use as a spice in cuisine. In water remediation, serving as a part of a composite material with MnO2, they were used for Methylene Blue removal. The material also had antibac- terial and photacatalitical properties. It was regenerated in HCl with no significant loss in the capacity up to the third cycle. Methylene Blue was also removed on HCl-modified Nigella seeds and on a series of biocomposites containing metal oxides and the seeds: Fe2O3-SnO2 MnFe2O4 , additionally Fe2O3-ZrO2-modified Nigella seeds were used for As5+ adsorption. Paeonia ostii is an ornamental plant also used for oil extraction. Its seed coats, pretreated with NaOH, were employed to adsorption of Methylene Blue. Such treatment improved the adsorbent’s performance due to removal of lignin and hemicellulose from its structure 78 Applied Water Science Volume 2. Papaya (Carica papaya) seeds and the seeds defatted with hexanol using the soxhlet extraction were also used for adsorption of Methylene Blue [210–212]. Aspen (Populus tremula), Nerium oleander (a small tree or shrub) and Pergularia tomentosa (an herb) seeds were as well tested for Methylene Blue adsorption. The seeds had cellulose content in the range between 40 and 60% and lignin in the rage between 8 and 20%. The func- tional groups (hydroxyl and ester) present in their structure interacted with the sulfur and nitrogen atoms of the dye. The aspen tree seeds showed the lowest whilst the oleander seed the highest adsorption capacity. Psyllium is a small plant which its seeds are used in medicine for mucilage production. The seeds were used for remediation of water contaminated with Reactive Orange 16. Mango seeds exhibited good adsorption capacity in removal of Cr6+. The adsorbnet was effective, however required very low pH for optional functioning (pH 1). Black sesame (Sesamum indicum) seeds were used for removal of the same pollutant. In this case the seeds were coated with chitosan what increased the adsorption capacity approximately about two times. Chromium cation was also adsorbed on tamarind (Tamarindus indica) and wormwood (Artemisia absin- thium) seeds while adsorption of Cd3+ Cu3+ and Ni2+ was investigated on jujube (Ziziphus jujube) seeds. Clary sage (Salvia sclarea) were used for removal of Basic Violet 16 and Cassia surattensis seeds for oil pro- duction effluent treatment. 2.4.9 Stones or Pits Stones are mostly composed of cellulose, hemicellulose and lignin. Adsorbents of such type are usually pretreated by washing them with water, drying in temperature below 120 °C and cursing or grinding to obtain the deserved fraction. The adsorption capacities of the examples are given in Table 2.13. 2.4.9.1 Date Date pits were used as an adsorbent for Methylene Blue. The adsorption was the most intense in pH 3 and 7. In the low range, the mechanism was attributed to ion exchange of the dye with H+ adsorbed on the surface of the pits, whereas in the high range, the surface was negatively charged, hence adsorption of the cationic dye was increased. Another adsor- bent made from date pits was employed for removal of two industrial dyes, Basic Red 2 and Methyl Violet. The material was characterized with rough surface with macrospores. It was amorphous with low content of crystalline Low-Cost Alternative Adsorbents 79 Table 2.13 Application examples of plant stones-derived adsorbents in removal of pollutants from water. Adsorbate and adsorption Adsorbent capacity (mg g−1) Reference Apricot stones-NaOH Methylene Blue 95.24 Date pits Paracetamol Olive seeds 29.74 37.12 Date pits Methylene Blue 58.38 Date pits Basic Red-2 92 Methyl Violet 136 Date pits Hg2+ 38.5, Zn2+ 52.6 Date seeds Pb2+ Date seeds-HNO3 72 Date seeds-KOH 91 50 Date stones Phenol 132.37, p-nitro phenol 161.44 Date stones Br- 185.2 Date stones Pb2+, Cd2+ Date stones-DTPAD 15.63, 8.55 59.17, 89.30 Date stones Congo Red 148.50 Date stones Pb2+ Date stones-NaOH 35.71 47.61 Mango stone biocomposite Crystal Violet 352.79 Olive stones-melanic anhydride Cd2+ 240.96, Pb2+ 127.38 Olive seeds Methylene Blue 140.55 Olive stones Cu2+ 0.98 (Continued) 80 Applied Water Science Volume 2 Table 2.13 Application examples of plant stones-derived adsorbents in removal of pollutants from water. (Continued) Adsorbate and adsorption Adsorbent capacity (mg g−1) Reference Olive stones Cr6+ Fe0-olive stones 1.48 Magnetite-olive stones 2.45 4.11 Peach stones-ethyldiamine Cr6+ 24.68 Peach stone-citric acid Methylene Blue 178.25 Pb2+ 118.76 Cd2+ 37.48 Cu2+ 32.22 Peach stones Cr6+ 23.26 Roasted date pits (RDP) Hg2+ Sulpate-modified RDP 282 Silane-modifies RDP 280 90 Sour cherry stones Cr6+ 0.53 phases and low specific surface area (below 0.5 m2 g−1). In its structure many carbonyl and hydroxyl groups were detected. Adsorption of Methyl Blue was approximately 1.5 times more efficient than of the other dye. Date pits were also used as a precursor for a Hg2+ adsorbent. The pits were roasted in elevated temperature (130 °C) and then one portion mod- ified with carbon sulfide and the other with 3-mercaptopropyltriethoxy- silane. The Roasted pits and sulfide-modified exhibited higher (2.3 times) adsorption capacity than the activated carbon tested in the study. Paracetamol was adsorbent on date pits and olive seeds. The study showed that the adsorption rate on the olive seeds was faster (4 times) than on the date pits and the adsorption capacity on that material also reached higher level. Dates stones were also used for adsorption of Pb2+. The starting material was boiled either with KOH or HNO3 of different concentrations and during varying time. Such proceedings aimed to enrich the material in various functional groups formed as a result of the biomass oxidation. The modification with acid inserted the material capacity roughly by 30% while alkaline treatment reduced it 30% relating to the untreated stones. Date stones were also used for removal of phenol and p-nitro phenol , Low-Cost Alternative Adsorbents 81 bromide ions with efficiency 20% greater than on activated carbon , Pb2+ and Cd2+ on raw and stones modified with diethylenetriaminepenta- acetic dianhydride , Congo Red (regeneration with acetone, signifi- cant decrease in the maximum adsorption capacity in subsequent cycles) , Pb2+ on raw and NaOH treated seeds , or Hg2+ and Zn2+. 2.4.9.2 Olive Olive seeds, powdered and dried, were applied for Methylene Blue adsorp- tion in continuous fixed bed column and in adsorption of Cu2+ in similar system. Olive stones used as a support for zero-valent iron and magnetite nanoparticles were tested for Cr6+ adsorption. The coated adsorbents showed 1.5 and 3 times increased adsorption capacity for the cation, respectively. A mixture of water, NaOH and oxalic acid was effi- cient for recovery of the adsorbed Cr6+ but deactivated the adsorbent, whereas sonification in water, although slightly less efficient, allowed to use the material again. Olive seeds modified with melanic anhy- dride (esterification upon pretreatment with NaOH) were used for Cd2+ and Pb2+ adsorption. The removal was characterized with high rate (15 min for 95% completion, the rate of Cd2+ higher than of Pb2+) due to the presence of abundant negatively charged maleate functional groups and higher selectivity towards Cd2+. The adsorbent was also regenerated with a solution of NaCl and used in 3 cycles without a significant loss of its capacity. 2.4.9.3 Other Powderized mango stone was used for fabrication of a biocomposite with ferric chloride and sodium borhydrate. The material was used for adsorp- tion of Crystal Violet. The best results were achieved in slightly alkaline pH (8). The material was regenerated by washing with CH3COOH that acted better than HCl, HNO3 or H2SO4. Chemically modified with NaOH apricot stones were used for removal of Methylene Blue. Limited amount of NaOH used for the treatment had beneficial effect on the adsorption capacity. The base was able to penetrate into to adsorbent surface and decrease the number of acid adsorption sites. Sour cherry stones were used for water remediation from Cr6+. Citric acid-modified peach stones were applied for remediation of water polluted with heavy metal cations and Methylene Blue. The modification aimed to increase the number of carboxyl groups (acting as active adsorption sites) in the mate- rials structure on the way of esterification reaction between the cellulose 82 Applied Water Science Volume 2 in the stone and the citric acid. Peach stones unmodified and modified with ethylenediamine were employed in adsorption of Cr6+. In the first modification step, the hydroxyl groups were introduced to the material structure by washing in NaOH, then epichlorohydrin was grafted to the groups serving as a bridge to graft ethylenediamine. The adsorbent was also regenerated and used in 4 cycles without significant loss of its capacity (regeneration method not disclosed). 2.4.10 Tea Global production of tea in 2017 was estimated to reach 6.1 · 106 tons. This produces a lot of waste that may be valorized as an adsorbent. Being a natural material, the morphology of spent tea varies. It can be microp- orous or mezoporous amorphous material smooth or rough regular. Its surface area is generally below 15 m2 g−1. The material contains functional groups such as aliphatic carbons, carbonyl groups, polysaccharides, car- boxyl groups, polyphenols, amine groups, hydroxyl groups. The adsorp- tion capacities of the examples are given in Table 2.14. Tea waste-polyvinyl alcohol composite was applied for Cr6+ removal. The material reached higher removal rates compared to the raw tea. The adsorption took place mainly on the sulfonate gropus, carboxylate anions and sulfonyl chlorides present in the material. Spent tea-based adsorbent was also applied for of Cr6+ removal from tannery wastewater, the adsorbent reached good removal efficiency and was reused in sev- eral cycles with only a slight decrease in the capacity. Another two metal cations, As3+ and Ni2+, were removed on tea derived adsorbent. The adsorption capacity was higher for Ni2+ and there was an inhibitory action on the adsorption in the binary systems. Tea waste modified with Fe3O4 nanoparticles and sodium dodecyl sulfate was used for removal of Basic Blue Dye. In this case ion exchange on the sulfonate groups and film diffusion were the two main adsorption mechanisms. An adsor- bent obtained from extruded and loaded with Zr spent tea was applied for removal of F−. The extrusion process increased the material’s pore size exposing more active sites for the adsorption, hence the adsorption level was higher compared to the not-extruded and the raw counterparts. The adsorbent also worked well in broad pH range. Fluoride was also removed on a tea waste loaded with Al, Fe and Al/Fe oxides. The material containing both Al and Fe was the most efficient one. An adsorbent prepared from spent tea by amine crosslinking was applied for removal of nitrates and phosphates. The material reached high removal levels and was effective in wide pH range. The adsorption mechanism was not only Low-Cost Alternative Adsorbents 83 Table 2.14 Application examples of spent tea-derived adsorbents in removal of pollutants from water. Adsrobate and adsorption Adsorbent capacity (mg/g) Reference Spent tea Ni2+ 0.31, As3+ 0.42 Cr6+ 90.90 U6+ 24.41 U6+ 142.21 17 β-estradiol 4.01 17 α–ethinylestradiol 2.97 Bisphenol A 16.26 Progesterone 1.8 Hydralazine hydrochloride 131.45 Phenol 47.51 Penicillin 46.36 Nitrobenzene 14.86 Tea-polyvinyl alcohol Cr6+ 4.71 Tea U6+ Graphene oxide-tea 91.72 Reduced graphene oxide/Fe3O4/Tea 111.61 104.94 Amine-cross-linked tea Phosphates 97.72 Nitrate 136.43 Tea-Zr F- Tea-Fe 20.56 Tea-Al 3.83 Tea-Al-Fe 10.47 18.52 (Continued) 84 Applied Water Science Volume 2 Table 2.14 Application examples of spent tea-derived adsorbents in removal of pollutants from water. (Continued) Adsrobate and adsorption Adsorbent capacity (mg/g) Reference Peppermint Malachite Green Green tea 69.78 Decaf green tea 27.44 Chamomile 35.03 24.46 Tea-Fe3O4-SDS Basic Blue dye Tea- H2SO4 Tetracycline 381 Methylene Blue 1007 Cr6+ 483 physisorption but also ion exchange of the adsorbates and chlorine. The removal of U6+ was studied on several tea-based materials: a hybrid adsorbent comprising grapheme oxide, reduced graphene oxide and Fe3O4 , and on simple tea waste [252,253]. Tea-based material were also applied for removal of endocrine disrupting compounds: 17 β-estradiol, 17 α-ethinylestradiol and bisphenol A , and progesterone. The adsorption efficiencies were comparable to the one obtained by granu- lated activated carbon. Adsorption on various teas including green tea, peppermint, chamomile and decaffeinated green tea was studied for a dye Malachite Green. Significant difference in adsorption capacities was dis- covered with pepper mint having the highest and chamomile the lowest one. Tea modified with H2SO4 was used for removal of tetracycline, Cr6+ and Methylene Blue. The acid treatment increased significantly the material’s adsorption capacity. Spent tea waste was applied also for removal of hydralazine hydrochloride , phenol , penicillin and nitrobenzene. 2.5 Disadvantages Natural, low-cost adsorbents, mostly agricultural waste could seem like a good solution for water treatment: first waste finds its application and is valorized, second, harmful substances are removed from water in an eco- nomically-friendly fashion. This solution should be very interesting for the industry however, it has not been widely implemented. The question Low-Cost Alternative Adsorbents 85 behind the reason could be answered by focusing on few aspects com- monly omitted by the authors. The first aspect is the materials stability. None of the research described in this chapter relates to this issue. The focus is put only on adsorption capacity changes upon regeneration cycles (if regeneration studies are even included in the research’s scope). The true question here is how long will it take before the adsorbent begins to decompose. Most of the materials con- tain high content of organic matter and it is obvious that after prolonged contact with water in ambient temperature they will eventually start to decay. This in consequence should trigger gradual release of organic com- pounds from the adsorbent to the effluent. Depending on the scale, it could cause contamination of the water greater than before the treatment. Assuming that this process would take some time to start, the adsorbent would have already removed a certain quantity of pollutants that would be later released to the water. The concentration could be much higher than in the influent as this system would function as kind of a solid phase extraction system. The second aspect is related to the sanitary issues connected with micro- organisms’ growth. The adsorbents could work as growth medium for bac- teria and fungi. Such could produce toxic substances contaminating the water or clog the filters due to formation of films on the adsorbent surface rendering the process impossible to operate. This, together with the ques- tionable materials’ stability, aside from the contamination, may addition- ally change the water’s taste, smell and most probably color. The third aspect concerns the materials’ mechanical properties. First of all, they should swell in the column upon contact with water, as they had been dried losing considerable amount of their volume. Second, due to decomposition it could be expected that the adsorbents will fragmentate into small particles that will be pushed by the flow along the column. Both the processes could tamp the bed conducing clogging of the column. The forth aspect—the logistics. The martials, in their vast majority, exhibit adsorption capacity that is much lower compared to conventional adsorbents. Hence, to provide an ample amount required for attaining required water quality, there is a need of having sufficient quantity of the raw product. Having in mid that the mass loss upon drying may be signifi- cant, obtaining such amount may be problematic. Especially in the light of the fact that most of the sources are rather dispersed (aside from big food processing factories) and the materials would be most probably mixed with other organic waste and needed to be sorted. Consequently there would be a need of creating a whole infrastructure to collect sufficient amount of the waste, that would have to be transported to the facility. 86 Applied Water Science Volume 2 The fifth aspect rising attention is the spent adsorbent. It will be com- posed of putrid organic matter contain high amount to toxic compounds. Such should be classified as hazardous waste and handled and disposed of in special manner that will generate additional costs. 2.6 Conclusions Low-cost materials, waste generated by various industries, exhibit adsorp- tion properties for various pollutants commonly found in wastewater. They can be used in their raw form or after subjection to modifications resulting in enhancement of the adsorption properties. The adsorptive properties originate from diverse functional groups present in the materials’ struc- ture: the carboxylic, carbonyl, carboxyl anime, and the hydroxyl groups are the most common adsorption centra. The materials first have to washed in order to remove impurities, dried and ground to the desired fraction. Although the raw waste and the adsorbents obtained from them seem as a very attractive alternative for the conventional materials used in water treatment, there is a series of issues impeding their wide application. First of all problematic may be the materials’ mechanical properties, stability and their susceptibility to decomposition and microorganisms growth. The second issue is the availability, the third the logistics and the need of developing proper transporting infrastructure, and last but not least the problem related to the generation of substantial amounts of hazardous waste. References 1. Sharma, S.K., Sanghi, R et al, Green practices to save our precious “Water Resource”, in: Advances in Water Treatment and Pollution Prevention, S. Sharma, R. Sanghi (Eds.), pp. 1–36, Springer Netherlands, Dordrecht, 2012. 2. Mishra, A., Clark, J.H. Preface, in: Green Materials for Sustainable Water Remediation and Treatment, A. Mishra, J.H. Clark (Eds.), pp. 1–5, Royal Society of Chemistry, 2013. 3. Srinivasan, R. Natural polysaccharides as treatment agents for wastewater, in: Green materials for sustainable water remediation and treatment, A. Mishra, J.H. Clark (Eds.), pp. 51–81, Royal Society of Chemistry, 2013. 4. Sharma, R.K., Adholeya, A., Das, M., Puri, A. Green materials for sustain- able remediation of metals in water, in: Green materials for sustainable water remediation and treatment, A. Mishra, J.H. Clark (Eds.), pp. 11–26, Royal Society of Chemistry, 2013.