1st Lecture 2024 PDF
Document Details
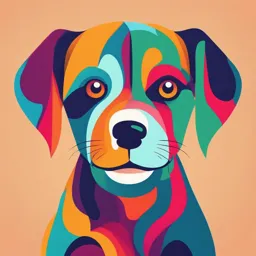
Uploaded by CureAllFuchsia2959
Reykjavík University
2024
Dr. Paolo Gargiulo
Tags
Related
- BME520 Biomedical Devices Design and Troubleshooting Chapter 2 Concepts and Requirements PDF
- Biomedical Devices Design and Troubleshooting (BME520) Chapter 2 PDF
- Medical Instrument Electrical Safety PDF
- Bio-Medical Instrumentation (UEI608) Lecture Notes PDF
- Lect_1_Biomed_instru_design_5th_BME_Thi_Qar_Uni_Ali_Basim_Mahdi PDF
- Med Term Exam 2024 PDF
Summary
This is a lecture on biomedical instrumentation for 2024. It covers topics such as course organization, instrumental amplifier design, and different types of measurements and sensors.
Full Transcript
Mælitækni og lífsmörk 2024 Introduction Dr. Paolo Gargiulo, Professor Course organization Theoretical lectures and calculation exercise (reference book: Medical Instrumentation Application and Design, John G. Webster 4th edition, Chapters 1-12 with so...
Mælitækni og lífsmörk 2024 Introduction Dr. Paolo Gargiulo, Professor Course organization Theoretical lectures and calculation exercise (reference book: Medical Instrumentation Application and Design, John G. Webster 4th edition, Chapters 1-12 with some exception). Quizzes (individual, 40% of the final grade). 1st Development of the instrumentation amplifier for EKG applications and comparison with a manufactured EKG amplifier (group projects, 40% of the final grade). Assessment of the two devices in terms of: quality of the signal, physiological measurement (such as: QRS, R-R, ST intervals) and common mode rejection ratio CMMR. Group Presentation of the results. 2nd LAB. Bio Signals and Medical Instrumentation measurements ( group project, 20% of the final grade): Acquisition and Classification of EMG, HRV and CoP associated to VIRTUAL REALITY environment. Group Presentation of the results. Instrumental amplifier design ECG signal Amplitude: 1-5 mV Bandwidth: 0.05-100 Hz Largest measurement error sources: – Motion artifacts – 50/60 Hz powerline interference Typical applications: – Diagnosis of ischemia – Arrhythmia – Conduction defects Instrumental amplifier design Postural control Lab https://biovrsea.is/ Course Organization The course is held in presence. Course organization may change if needed.. Lectures/Lab: Mondays (3hours) and Thursday (3 hour) TA: Alfonso Ponsiglione, Carlo Ricciardi and Federica Kiyomi Ciliberti Lab manager: Hannes Páll Þórðarson Medical device/ Biomedical Instrumentation, what is it? Medical Device/Instrumentation or Technology A medical device or technology is any article, including software, intended to be used by humans for the diagnosis, prevention, monitoring or treatment of a disease, injury or physiological process. This includes products such as complex capital equipment (including operating theatre equipment and diagnostic imaging equipment such as x-ray machines and magnetic resonance imaging (MRI) scanners), high-tech advanced devices (such as Cochlear ear implants, artificial hearts and other implantable devices), simple, low-risk devices (such as bandages and walking sticks), pathology tests and diagnostic devices such as in vitro diagnostic test kits. General block diagram for Medical Instrumentation Calibration signal Transducer Conditioning processing sensor Subject/ display Measurand Memor. feedback Comunicazione Radiation, current, and others… Measuring subject Measurand: The physical quantity, the property or the condition that the biomedical instrumentation will measure. – The Measurand of biomedical interest can be listed as follows: biopotentials, pressure, flow, dimensions (bioimaging), displacements (velocity, accelerations, forces), impedance, temperature, chemical concentrations, etc. The accessibility of the Measurand is very important since it can be internal (eg. blood pressure), available on the body surface (bioelectric potentials), can be irradiated by the body (infrared emission), can be obtained from a tissue sample (collection of blood, biopsy), etc. Significant factors in measurements WWW.HR.IS Accuracy vs. Precision Precision is often confused with accuracy. High precision does not imply anything about measurement accuracy. Precision represents degree of repeatability of several Accuracy represents degree independent measurements of correctness of the of desired input at the same measured value with reference conditions reference to true Precision of instruments value. depends on factors that Accuracy of instrument cause random or accidental depends on systematic errors. errors. Accuracy vs. Precision Measuring a fixed target position Low precision, low accuracy High precision, low accuracy High precision, high accuracy Sensitivity OUT OUT signal signal measured measured IN IN Quantity to be measured Quantity to be measured low Sensitivity high Sensitivity The sensitivity of measurement is a measure of the change in instrument output that occurs when the quantity being measured changes by a given amount. Thus, sensitivity is the ratio: Change of output signal /change of input signal Static sensitivity The static sensitivity of an Output instrument is the slope of Drift of sensitivity(offset) the input-output curve at a specified input value. ideal If the output inlet curve is a straight line, the sensitivity does not depend on the input value and coincides with the angular coefficient of the Input straight line Instrument Drift It is defined as the variation of output for a given input caused due to change in sensitivity of the instrument due to certain interfering inputs like temperature changes, component instabilities, etc. Prime sources occur as chemical structural changes and changing mechanical stresses. Drift is a complex phenomenon for which the observed effects are that the sensitivity and offset values vary. It also can alter the accuracy of the instrument differently at the various amplitudes of the signal present. Hysteresis Careful observation of the output/input relationship of a block will sometimes reveal different results as the signals vary in direction of the movement. Mechanical systems will often show a small difference in length as the direction of the applied force is reversed. The same effect arises as a magnetic field is reversed in a magnetic material. This characteristic is called hysteresis. Hysteresis is defined as the magnitude of error caused in the output for a given value of input, when this value is approached from opposite directions ; i.e. from ascending order & then descending order. Causes are backlash, elastic deformations, magnetic characteristics, frictional effects (mainly). Hysteresis can be eliminated by taking readings in both direction and then taking its arithmetic mean. DYNAMIC performance of measuring instruments Only a few biomedical measures (such as body temperature) are constant or variable amounts very slowly. Most of the medical equipment must process signals that are a function of time. It is therefore interesting to study the dynamic response of a measuring instrument. Linear differential equations with constant coefficients can very often be used to describe the relationship between input and output of an instrument. Dynamic performance of measuring instruments Input output y instrument z If we consider an instrument with input y and output z, the relation between input and output can be expressed by the formula d n z (t ) d n-1 z (t ) dz (t ) d n y(t ) d n-1 y(t ) dy (t ) an n + a n -1 n -1 +... + a1 + a 0 z (t ) = bn n + bn -1 n -1 +... + b1 + b0 y(t ) dt dt dt dt dt dt k d Repalcing with the differntial operator: D k = k dt (a D n n ) ( + a n -1 D n -1 +... + a1 D n + a 0 z (t ) = bn D n + bn -1 D n -1 +... + b1 D n + b0 y (t ) ) Dynamic characteristics In the case of linear or linearizable systems, the dynamic analysis of the response of the transducer system to the input signal can be expressed in a fairly simple form. The treatment is due to the evaluation of: TRANSFER FUNCTION, input impedance output impedance The transfer function can be formulated with a linear mathematical model of the system by means of partial differential equations that describe the relationships between input and output to the transducer on the basis of the physical laws that regulate its operation. Dynamic response The relationship that is obtained between input and output, in general, is a linear differential equation with constant coefficients (in the only time variable) The order of the differential equation distinguishes the order of the sensor (eg sensitive elements of the first order, second order, etc.) The solution of the differential equation allows to obtain the temporal response of the sensor to an input signal The solution of linear differential equations with constant coefficients can be performed with normal integration techniques, or you can resort to techniques such as the Laplace transform (replaces the differential equations => algebraic equations) Input output y instrument z Zero order systems If we consider a zero order system (no element that stores energy) with input y and output z, the relation between input and output can be expressed as: a0 z (t ) = b0 y(t ) b0 z(t ) = Ky(t ) K= a0 It is characterized by a transfer function that can be put in the form: z (D ) =K z jw =K ( ) y (D ) y( jw ) Input output y instrument z First order systems If we consider a system of the first order (one element that stores energy, the system identified by a first-degree differential equation) with input y and output z. The relationship between input and output can be expressed as: dz (t ) b0 a a1 dt + a0 z (t ) = b0 y (t ) (tD +1)z(t ) = Ky(t ) K= a0 ; t= 1 a0 It is characterized by a transfer function that can be placed in the form : z ( jw ) K = y( jw ) (1 + jwt ) z (D ) K = y (D ) (1 + tD ) Input output y instrument z Second order systems If we consider a second-order system (two elements storing energy, system identified by a second-degree diff equation) with input y and output z, the relationship between input and output can be expressed as: d 2 z (t ) dz (t ) éD 2 2zD ù a2 + a + a0 z (t ) = b0 y(t ) ê 2 + + 1ú z (t ) = Kx(t ) dt 2 1 dt ë wn wn û b a a1 K = 0 ; wn = 0 ; ζ = a0 a2 2 a0 × a2 It is characterized by a transfer function that can be put in z ( jw ) the form: K = y ( jw ) æ æ jw ö 2 2zjw ö çç ÷ + + 1÷ ç çè wn ÷ø wn ÷ è ø Frequency response 1.0 amplitude 0.1 0.05 Hz 150 Hz frequency Frequency Response of an electric or electronics circuit allows us to see exactly how the output gain (magnitude response) and the phase (phase response) changes at a particular single frequency, or over a whole range of different frequencies from 0Hz, (d.c.) to many thousands of mega-hertz, (MHz) depending upon the design characteristics of the circuit. Noise Ampiezza Ampiezza tempo tempo Original signal Noise signal Amplifier saturation Ampilitude 5 mV Input time dinamic input range -5 mV Amplitude 1V Time output -1 V OFFSET Amplitude time input Amplitude DC offset output time Analog and digital signals Amplitude Amplitude time time Analog signal Digital signal Signal sampling Amplitude Amplitude time time Continue signal Sampled signal Transducers Typically, the term transducer is defined as a device that converts one form of energy into another. Normally a transducer converts the measurand to a usable electrical signal A Transducer is a device capable of converting the physical quantity into a proportional electrical quantity such as voltage or current. The trasducer should: a. Respond only to the form of energy present in the measuring subject b. Interfacing to the biological system in a minimally invasive way, minimizing the extracted energy BLOCK DIAGRAM OF TRANSDUCERS Transducer contains two parts that are closely related to each other i.e. the sensing element and transduction element. The sensing element is called as the sensor. It is device producing measurable response to change in physical conditions. The transduction element converts the sensor output to suitable electrical form. TRANSDUCERS SELECTION FACTORS Operating Principle: The transducer are many times selected on the basis of operating principle used by them. The operating principle used may be resistive, inductive, capacitive , optoelectronic, piezo electric etc. Sensitivity: The transducer must be sensitive enough to produce detectable output. Operating Range: The transducer should maintain the range requirement and have a good resolution over the entire range. Accuracy: High accuracy is assured. Cross sensitivity: It has to be taken into account when measuring mechanical quantities. There are situation where the actual quantity is being measured is in one plane and the transducer is subjected to variation in another plan. Errors: The transducer should maintain the expected input- output relationship as described by the transfer function so as to avoid errors. Transient and frequency response : The transducer should meet the desired time domain specification like peak overshoot, rise time, setting time and small dynamic error. Loading Effects: The transducer should have a high input impedance and low output impedance to avoid loading effects. Environmental Compatibility: It should be assured that the transducer selected to work under specified environmental conditions maintains its input- output relationship and does not break down. Insensitivity to unwanted signals: The transducer should be minimally sensitive to unwanted signals and highly sensitive to desired signals. Signal Conditioning Signal conditioning is the manipulation of an analogue signal in such a way that it meets the requirements of the next stage for further processing. Generally, a sensor can not be connected directly to the display unit: – For example, the signal output to the sensor must be simply amplified, filtered (to eliminate unwanted signal components) or adapted (to adapt the impedance towards the later stages, or converted into digital signals for the interface with microProcessor). Signal visualization The result of the measurement process must be appropriately visualized in a form such that a human operator can perceive it. In general, the display forms can be numeric or graphic, discrete or continuous, permanent or temporary, etc. Consider also to exploit other senses of the person such as hearing, (eg phonoendoscope, velocity Doppler signals, etc.) Remember also the alarm signals (often optical-acoustic) widely used in various biomedical instruments. Measurements, functional modality: Direct and indirect mode Data acquisition, functional modality: Sample mode and continuous recording Sample mode: Measurements at certain times: Some measures (eg. temperature, ion concentration, etc.) vary very slowly and can be measured once in a while Continuous measurements: Other measures (eg. ECG, respiratory flow, etc.) require continuous (or very frequent) measurement. Signals, Analog / Digital and Real-time / store and forward A distinction may be between intrinsically continuous (analog) and discrete (digital) signals that can only take a finite number of values A sensor that immediately provides the estimate of the measurand is said to act in real-time. Alternatively, the measurement may not be provided at the time of measurement but with a certain delay, e.g. due to signal processing (deferred time). (eg cell cultures) Some physiological signals Measures Amplitude Freq. Hz Method Blood flows 1 - 300 ml/s 0 – 20 Elettromagnetic, ultrasound Blood pressure 0 - 400 mmHg 0 – 50 strain gage Cardiac output 4 - 25 l/min 0 – 20 Fick, diluzione color. o term. Electrocardiography(ECG) 0.5 - 4 mV 0.05 – 150 Cutaneous electrodes Electroencephalography(EEG) 5 - 300 µ V 0.5 – 150 Cutaneous electrodes Electromyography (EMG) 0.1 - 5 mV 0 – 10000 Cutaneous electrodes (needles) Electroretinography (ERG) 0 - 900 µ V 0 – 50 Lente Elettrodo Electrooculography (EOG) 0 – 50 Cutaneous electrodes 50 - 3500 µ V pH 3 - 13 pH units 0–1 Elettrodes for pH pCO2 40 - 100 mmHg 0–2 Elettrodes for pCO2 pO2 30 - 100 mmHg 0–2 Elettrodes for pO2 Pneumotachography 0 - 600 L/min 0 – 40 Pneumotacometer Impedenziometria,sens. Respiratory Freq 2 - 50 atti/min 0.1 – 10 dilataz. toracica, termistore nasale Temperature 32 to 40 °C 0 - 0.1 Termistori, termocoppie, etc. Characteristics amplitude/frequency of some bio-potentials Amplitude Frequency