Opioid and Nonopioid Analgesics PDF
Document Details
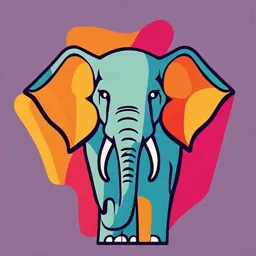
Uploaded by ThrivingSavannah9407
University of Puerto Rico Medical Sciences Campus
John J. Nagelhout
Tags
Summary
This document provides a comprehensive overview of opioid and nonopioid analgesics. It explores their historical context, various classifications, and mechanisms of action. The text includes detailed information regarding opiate receptors and their roles in pain modulation, along with specific examples of opioid drugs used in clinical practice.
Full Transcript
11 Opioid and Nonopioid Analgesics John J. Nagelhout In the past, opium was used as a topical, intravenous, and inhaled analgesic. One of the earliest uses of opium is found in Greek literature dating from 300 bc. Opium sponges, referred to as soporific sponges, were used for the control of pain as...
11 Opioid and Nonopioid Analgesics John J. Nagelhout In the past, opium was used as a topical, intravenous, and inhaled analgesic. One of the earliest uses of opium is found in Greek literature dating from 300 bc. Opium sponges, referred to as soporific sponges, were used for the control of pain as early as the 14th century. An attempt to administer opioids by the intravenous route was attributed to Elsholtz in 1665, approximately 200 years before the invention of the syringe and needle. The first attempt to administer an opium vapor by inhalation was documented in 1778. It was not until 1853, when the syringe and needle were introduced into clinical practice by Wood, that an accurate dose of opioid could be administered intravenously.1,2 In 1803, Sertürner reported the isolation of a pure substance from opium that he named morphine, after Morpheus, the Greek god of dreams. Other opium alkaloids were soon discovered: codeine by Robiquet in 1832 and papaverine by Merck in 1848. Abuse of opium and isolated alkaloids led to the synthetic production of potent analgesics. The goal of synthetic manufacture of analgesics was the creation of potent analgesics that would have high specificity for receptors, were not addictive, and were free of side effects. Synthetic production led to the development of opioid agonists, partial agonists, agonists- antagonists, and antagonists.1,2 OPIOIDS Opioid is a term used to refer to a group of drugs, both naturally occurring and synthetically produced, that possess opium-or morphine-like properties. Opioids exert their effects by mimicking naturally occurring endogenous opioid peptides or endorphins. The term narcotic is derived from the Greek word narkōtikos, meaning “benumbing,” and refers to potent morphine-like analgesics with the potential to produce stupor, insensibility, and dependence. Narcotic is not a useful term in a pharmacologic or clinical discussion because of its legal connotations. Several systems of classification are used to describe opioids. One common method divides the opioids into four categories: agonists, partial agonists, agonists-antagonists, and antagonists. Another system of categorization is based on the chemical derivation of the opioids and divides them into naturally occurring, semisynthetic, and synthetic compounds, with each group having subgroups (Box 11.1).3 Other classification systems describe the drugs as either weak or strong or hydrophilic and lipophilic. The term opioid is derived from the word opium (from opos, Greek for “sap”), an extract from the poppy plant Papaver somniferum. The properties of opium are attributable to the 20 different isolated alkaloids, and the alkaloids are divided chemically into two types: phenanthrene (from which morphine and codeine are derived) and benzylisoquinoline (from which papaverine, a nonanalgesic drug, is derived). Modification of the morphine molecule with retention of the five-ring structure results in the semisynthetic drugs heroin and hydromorphone. When the furan ring is removed from morphine, the resulting four-ring synthetic opioid levorphanol is formed. The 138 phenylpiperidines (e.g., fentanyl) and the diphenylheptane derivatives (e.g., methadone) all have only two of the original five rings of the basic morphine molecular structure. A close relationship exists between the stereochemical structure and potency of opioids, with the levo-isomers being the most potent. All opioids, despite the diverse molecular structures, share an N-methylpiperidine moiety, which seems to confer analgesic activity.1 Fig. 11.1 illustrates the structures of the commonly used opioids. Opioid drugs produce pharmacologic activity by binding to opiate receptors primarily located in the central nervous system (CNS), supraspinal and spinal, and in several peripheral sites. These include the gastrointestinal (GI) system, vasculature, lung, heart, and immune systems. Supraspinal analgesia occurs through activation of opioid receptors in the medulla, midbrain, and other areas, which causes inhibition of neurons involved in pain pathways. Spinal analgesia occurs by activation of presynaptic opioid receptors, which leads to decreased calcium influx and decreased release of neurotransmitters involved in nociception. Clinically, supraspinal and spinal opioid analgesic mechanisms are synergistic.3 This explains why opioids such as fentanyl and sufentanil produce more profound analgesia when delivered epidurally than when delivered systemically, despite the similar blood concentrations measured with both routes of administration.1,4 Opiate Receptors Opiate receptors have been deoxyribonucleic acid (DNA) and amino acid sequenced and cloned. The discovery of opioid receptors can be traced back to the 1950s, when pharmaceutic companies were involved in research in anticipation of the development of an effective nonaddictive analgesic. In 1973, the examination of vertebrate species led to the discovery of three opiate receptor classes that mediate analgesia.5 Questions emerged as to why the receptors existed, and further research led to the hypothesis that the receptors possess endogenous functions. After the discovery of opiate receptors in the early 1970s, the search began for endogenous substances that were their agonists. In 1975, three such agonists were identified: enkephalins, endorphins, and dynorphins.5 Each group is derived from a distinct precursor polypeptide and has a characteristic anatomic distribution. By the early 1980s, three precursor molecules to these agonists were identified: proenkephalin, pro-opiomelanocortin, and prodynorphin.1,5 Opioid peptides share the common amino-terminal sequence of Try-Gly-Gly-Phe-(Met or Leu), which has been labeled the opioid motif or message and is necessary for interaction at the receptor site. The peptide selectivity resides in the carboxy-terminal extension, providing the address.1 Since then, an additional precursor, pronociceptin, that results in nociception, has been identified. Endomorphins 1 and 2, which are agonists at mu (μ) receptors, have been identified, but the precursor’s molecule has not yet been defined.3 In 1975 Hughes and Kosterlitz6 identified the first endogenous substance with opioid activity. 139 CHAPTER 11 Opioid and Nonopioid Analgesics HO BOX 11.1 Classification of Opioids Based on Chemical Class Naturally Occurring Opium Alkaloids Phenanthrene Derivatives Morphine Codeine Thebaine Synthetic Opioids Morphinans Levorphanol Nalbuphine (Nubain) Butorphanol (Buprenex) Dextromethorphan Semisynthetic Phenanthrenes Oxymorphone (Opana) Hydromorphone (Dilaudid) Diacetylmorphine (Heroin) Hydrocodone (Vicodin) Oxycodone (Oxycontin) Diphenylheptanes (Methadones) Methadone Antagonist Phenanthrenes Naloxone Naltrexone Methylnaltrexone Piperidines Anilinopiperidine Loperamide Benzylisoquinoline Derivatives Papaverine Thebaine Derivatives Buprenorphine Oxycodone Morphine O H NCH3 HO CH3 N Meperidine C6H5 Benzomorphans Pentazocine (Talwin) Phenazocine Fentanyl COOC2H5 CH3CH2CON N CH2CH2 CH2 S Phenylpiperidines Meperidine (Demerol) Alfentanil Fentanyl Sufentanil Remifentanil CH2 Sufentanil CH2 C O CH3 CH2 O CH3 O N N Alfentanil Martin et al.7 were the first to provide evidence for opiate receptor subtypes (Table 11.1). Their findings provided evidence for the existence of three opiate receptors: mu, kappa (κ), and sigma (σ), named after their respective agonists—morphine, ketocyclazocine, and SK&F 10047. The sigma receptor was later determined not to be an opiate receptor. A delta (δ) receptor was subsequently identified. Each major opioid receptor has a unique anatomic distribution in the brain, spinal cord, and periphery.8 Their diversity is greatest in their extracellular loops.1 A fourth receptor has been cloned and named opiate receptor- like (ORL1) or the nociceptin orphanin FQ peptide receptor (NOP). Because this receptor family awaits further clarification as to its role in pain signaling and does not display opioid pharmacology, discussion of this category is not included here. Opioid receptors are expressed by central and peripheral neurons and by pituitary, adrenal, immune, and ectodermal cells.9 As noted previously, there are three main types of opioid receptors in the CNS: the mu, delta, and kappa receptors; the endogenous ligands for each receptor are noted in Table 11.2.10 They are not generally selective to one receptor subtype.11 Additional receptor types were proposed (sigma, epsilon, orphanin) but are no longer considered opioid receptors. They have several cellular mechanisms. There are seven transmembrane G protein–coupled receptors (GPCR) that show 50% to 70% homology between their genes.12 They either act to inhibit adenylyl cyclases and cyclic adenosine monophosphate (cAMP) production or they directly interact with different ion channels in the membrane. Opioid receptors can modulate pre-and postsynaptic calcium channels, suppressing calcium influx. This causes attenuation of the excitability of neurons and/or reduces the release of pronociceptive neuropeptides. In addition, opioid receptor activation leads to opening of G protein–coupled inwardly rectifying K+ (GIRK) channels, thereby preventing neuronal excitation and/or propagation of action potentials.13 C6H5 C6H5 CH2 CH3 CH2 N N N N CH2 CH2 O CH3 O N N C CH2 CH3 HO Naloxone O OH N CH2 CH N CH2 CH2 O HO Nalbuphine O OH HO Fig. 11.1 Selected opioid agonists and antagonists used as anesthesia adjunct drugs. Stimulation of the mu receptor produces supraspinal analgesia, euphoria, a decrease in ventilation, and most of the classic clinical actions of the opioid agonists. Kappa receptor stimulation produces spinal analgesia, sedation, and miosis. Currently, κ-opioid drugs are being investigated for antiinflammatory actions that reduce disease severity of arthritis and other inflammatory diseases.14–18 The delta receptor is responsible for spinal analgesia, responds to enkephalins, and serves to modulate activity of the mu receptors.3 Various subtypes of each of the three opiate receptors have been proposed but have not been well defined.8 140 UNIT II Scientific Foundations TABLE 11.1 Actions Produced at Each Opioid Receptor Subtype Effects Mu (μ) Receptor Kappa (κ) Receptor Delta (δ) Receptor IUPHAR name MOP KOP DOP Analgesia Supraspinal, spinal Supraspinal, spinal Supraspinal, spinal; modulates mu receptor activity Cardiovascular Respiratory Central nervous system Bradycardia Depression Euphoria, sedation, prolactin release, mild hypothermia, catalepsy, indifference to environmental stimulus Miosis Inhibition of peristalsis, nausea, vomiting Urinary retention Yes Yes Possible depression Sedation, dysphoria, psychomimetic reactions (hallucinations, delirium) Depression Pupil Gastrointestinal Genitourinary Pruritus Physical dependence Antishivering Miosis Diuresis (inhibition of vasopressin release) Low abuse potential Urinary retention Yes Yes Yes DOP, Delta opiate peptide; IUPHAR, International Union of Basic and Clinical Pharmacology; KOP, kappa opiate peptide; MOP, mu opiate peptide. NC-IUPHAR-Approved Nomenclature for Opioid Peptide Receptors TABLE 11.2 Current NC-IUPHAR- Approved Nomenclature Presumed Endogenous Ligand(s) μ, mu or MOP β-endorphin enkephalins δ, delta or DOP κ, kappa or KOP NOP Endomorphin-1 Endomorphin-2 Enkephalins β-endorphin Dynorphin A Dynorphin B α-neoendorphin Nociceptin/orphanin FQ (N/OFQ) Receptors activated by opiate drugs respond physiologically to endogenous opioid peptides; they are therefore opioid peptide receptors, the receptor family being designated by the two-letter abbreviation OP. The well-established Greek terminology for opioid receptor types using the descriptors μ, δ, and κ is recommended, but where Greek symbols are not permitted or impractical, the use of mu, delta, or kappa, or MOP, DOP, or KOP is permissible. No mechanism for the endogenous synthesis of endormorphins has been identified; their status as endogenous ligands for the mu receptor is tentative. NC-IUPHAR, International Union of Basic and Clinical Pharmacology Committee on Receptor Nomenclature and Drug Classification. Adapted from Alexander SPH, Christopoulos A, Davenport AP, et al. The concise guide to pharmacology 2019/20: G protein-coupled receptors. Br J Pharmacol. 2019;176(1):S21–S141. The mechanism of action of opioid drugs is shown in Fig. 11.2. The endogenous ligands for opioid receptors are noted in Table 11.2. Pharmacokinetics Pharmacodynamic and pharmacokinetic considerations must be combined to reach an ideal analgesic clinical state. Surgical requirements for analgesic drugs are very different from nonoperative uses. These differences include a much higher analgesic requirement, the coadministration of potent anesthetic and sedative drugs, and the ability to support respiratory effort so that respiratory depression is not an issue until the patient emerges from anesthesia. In anesthesia, opioids are most commonly administered by the parenteral, intrathecal, and epidural routes. They may also be given by oral, nasal, intramuscular, and transdermal administration. Physiochemical properties of opioids influence their pharmacokinetics. To reach effector sites in the CNS, opioids must cross biologic membranes from the blood to receptors on neural cell membranes. The ability of opioids to cross the blood-brain and placental barriers depends on molecular size, ionization, lipid solubility, and protein binding.19 The physicochemical characteristics, pharmacokinetic variables, and partition coefficients (octanol and water as a measure of lipid solubility) for several of the commonly used opioid analgesics are summarized in Table 11.3. The wide variation in dosing of the opioids in anesthesia, depending on the patient and surgical situation, leads to vastly different durations even with the same drug. Fentanyl, for example, can last from 30 minutes to 18 to 24 hours, depending on how it is administered and how much is given. The pharmacokinetic parameters are important, but the context of how they are used in clinical practice is a major factor in the ultimate patient response. Opioids are modestly absorbed orally. Some opioids undergo extensive first-pass metabolism in the liver, greatly reducing their bioavailability and therapeutic efficacy after oral dosing. Orally administered morphine has limited absorption from the GI tract. Drugs with greater lipophilicity are better absorbed through nasal and buccal mucosa and the skin. When small doses of the opioids are used, the effects are usually terminated by redistribution rather than metabolism. Larger or multiple doses or continuous infusions are much more dependent on metabolism for offset.19 Like most drugs, opioids are usually metabolized in the liver to more polar and less active or inactive compounds by both phase 1 and phase 2 processes. Some opioids such as morphine have active metabolites such as morphine-6-glucuronide that can prolong the therapeutic effects of the parent compound. The meperidine metabolite, normeperidine, is neurotoxic and may accumulate in the elderly or in patients with decreased renal or hepatic function. This has led to a decline in its use. It is avoided in the elderly or patients with renal or hepatic dysfunction and where chronic use may be needed.1 141 CHAPTER 11 Opioid and Nonopioid Analgesics Opioid binding site Cell Opioid receptor membrane K+ α K+ channel (inward rectifier) (+) (–) ATP β G-protein γ (–) (+) Ca2+ (Extracellular) OTFC (Intracellular) Voltage-gated Ca2+ channel (N-type) MAPK cascade Adenylyl cyclase Gene expression Cyclic AMP Phospholipase A2 Production of prostaglandins and leukotrienes Fig. 11.2 Opioid mechanisms of action. The endogenous ligand or drug binds to the opioid receptor and activates the G protein (three distinct protein subunits), resulting in multiple effects that are primarily inhibitory. The activities of adenylate cyclase and the voltage-dependent Ca2+ channels are depressed. The inwardly rectifying K+ channels and mitogen-activated protein kinase (MAPK) cascade are activated. AMP, Adenosine monophosphate; ATP, adenosine triphosphate; OTFC, oral transmucosal fentanyl citrate. (From Hemmings Jr. HC, Egan TD. Pharmacology and Physiology for Anesthesia. 2nd ed. Philadelphia: Elsevier, 2019:334.) TABLE 11.3 Physicochemical Characteristics and Pharmacokinetics Percent Nonionized Protein Binding (%) Vc (L/kg) Vd (L/kg) Clearance Elimination (mL/min/kg) Half-Life (hr) Partition Coefficient (Octanol/Water) Opioids pKa Morphine 7.9 23 35 0.23 2.8 15.5 1.7–3.3 1 Meperidine Methadone Fentanyl Sufentanil Alfentanil Remifentanil Butorphanol 8.5 9.3 8.4 8 6.5 7.26 8.6 7 N/A 8.5 20 89 58 17 70 85 84 93 92 93 80 0.6 0.15 0.85 0.1 0.12 0.1–0.2 0.1 2.6 3.4 4 2 0.6 0.39 5 22.7 1.6 13 12 5 41 38.6 3–5 23 2–4 2–3 1–2 0.1–0.3 2.65 21 115 820 1750 130 N/A 140 Nalbuphine 8.71 N/A N/A 0.45 4.8 23.1 3.7 N/A N/A, Not applicable; Vc, volume of distribution central compartment; Vd, volume of distribution. The opioid drugs are metabolized by the usual cytochrome enzymes, including CYP3A4, CYP2D6, and CYP2B6.20–22 Remifentanil was designed with an ester group in its structure and is metabolized by hydrolysis in the plasma and tissues by nonspecific esterases. Remifentanil has a low volume of distribution and a large clearance, which results in a short half-life of approximately 10 minutes. Opioids and their metabolites are excreted primarily by the kidneys and secondarily by the biliary system and GI tract.23 Clinicians become very adept in the art of administering opioids by bolus, incremental injection, or infusion to maximize analgesia during surgery as needed, yet allowing for safe and rapid recovery and residual postoperative analgesia. Pharmacogenetics The wide variations in dosing and patient responses have both pharmacodynamic and pharmacokinetic causes. Pharmacogenetics appears to be an important factor as well. Opioids have a narrow therapeutic index, calling for a fine balance between optimizing pain control and sedative effects (without causing respiratory depression), while at the same time recognizing great variability from patient to patient in response and dose requirements. Genetic factors regulating their pharmacokinetics (metabolizing enzymes, transporters) and pharmacodynamics (receptors and signal transduction) contribute to this variability and to the possibility of adverse drug effects, toxicity, or therapeutic failure of pharmacotherapy. Significant variation in conversion of the prodrug codeine into the active metabolite morphine has been noted.24 Polymorphisms in CYP2D6 are responsible for the wide variations among different populations.25 The clinical use of opioids involves knowledge regarding patient characteristics, their perception, severity, and likely duration of pain, lifestyle variables such as smoking habits and alcohol intake, and opioid drug and dosing regimen selection.25 Other factors affecting pharmacokinetics and pharmacodynamics of opioids include age, body weight, renal failure, hepatic failure, cardiopulmonary bypass, acid- base changes, and hemorrhagic shock. In adults, advancing age requires lower opioid doses for the treatment of postsurgical pain. Pediatric doses can vary widely as well. In addition, in relatively similar patient groups, dosage requirements vary. Aubrun et al.26 reported that in more than 3000 patients morphine dosage requirements for postoperative hip replacement therapy varied almost 40-fold. Large variabilities have been reported in cancer patients receiving morphine via various routes.25 Variability is contributed to by inherent pain sensitivity, tolerance, and other factors, 142 UNIT II Scientific Foundations BOX 11.2 Pharmacogenetics and Opiates BOX 11.3 Common Clinical Effects of Cytochrome Enzyme Subtype Acute Chronic Analgesia Respiratory depression Sedation Euphoria Dysphoria Vasodilation Bradycardia Cough suppression Miosis Nausea and vomiting Skeletal muscle rigidity Smooth muscle spasm Constipation Urinary retention Biliary spasm Pruritus, rash Antishivering (meperidine only) Histamine release Hormonal effects Tolerance Physical dependence Constipation Clinical Outcome CYP2D6 Codeine is a prodrug that is metabolized to its active form, morphine. Extensive metabolizers may produce dangerously high levels of morphine. See Chapter 6. Tramadol is metabolized to its M1 metabolite, which is six times more potent than the parent compound. Hydrocodone effectiveness can vary widely depending on the genetic status of the patient, which influences mu receptor binding. Oxycodone has significant variation and effectiveness depending on the metabolic genotype. CYP3A4 Fentanyl, buprenorphine, and methadone metabolism vary according to the activity of this enzyme group. CYP3B6 Women generally have a higher activity of these enzymes, which may alter the metabolism of methadone and propofol. Mu and kappa receptor Influence the dosing and efficacy of several clinical variants opioids. Adapted from Trescot AM. Genetics and implications in perioperative analgesia. Best Pract Res Clin Anaesthesiol. 2014;28(2):153–166; Gray K, Adhikary SD, Janicki P. Pharmacogenomics of analgesics in anesthesia practice: a current update of literature. J Anaesthesiol Clin Pharmacol. 2018;34(2):155–160. including pharmacogenetics influencing the clinical pharmacology of opioids.27,28 Some pharmacogenetic influences on the clinical performance of opioids are noted in Box 11.2. Clinical Effects of Opioids The common clinical effects of opioid agonists are listed in Box 11.3. Central Nervous System Effects: Analgesia, Sedation, and Euphoria Opiate analgesia results from actions in the CNS, spinal cord, and peripheral sites. They are most effective for visceral continuous dull pain; however, at high doses they will relieve any pain. They are less effective against neuropathic pain that requires chronic multimodal therapy.29–31 The sedative and euphoric actions contribute to the feeling of well-being in awake patients. The analgesic effects of opioids come from their ability to (1) directly inhibit the ascending transmission of nociception information from the spinal cord dorsal horn and (2) activate pain control pathways that descend from the midbrain, via the rostral ventromedial medulla to the spinal cord dorsal horn.1 The effect of opioids on electroencephalographic and evoked-potential activity is minimal, therefore neurophysiologic monitoring can be conducted during opioid anesthetic techniques. Opiate administration can indirectly cause an increase in intracranial pressure if respiratory depression-induced hypercarbia occurs. They have variable effects on cerebral vascular tone depending on the background anesthetic present. Possible untoward CNS effects when the opioids are used in neurosurgery are easily managed by controlling ventilation and maintaining adequate blood pressures.32 The comparative potency of the opioid agonists that are used in anesthesia is as follows: sufentanil > fentanyl = remifentanil > alfentanil. The sedative and euphoric effects of the opiates vary depending on the agent. Patients will exhibit sedation and euphoria that are different with μ- versus κ-agonists. Dysphoria can occur and appears more Opioid Agonists prominent with drugs that have strong kappa receptor effects or when opioids are taken in the absence of pain. Physical and psychological dependence occur with repeat administration as evidenced by physical withdrawal with abstinence and drug-seeking behaviors.1,33 The opiates are not anesthetics, so awareness under anesthesia is a concern when even high doses of opiates are used.34,35 Both acute and chronic tolerance occur with the opiates. Cross-tolerance among μ-agonists will occur. Usually a decrease in duration is noted first, followed eventually by a decrease in effect. The mechanism of tolerance is complex and does not appear to be due to a change in receptor number. Receptor internalization, activation of N- methyl- D- aspartate (NMDA) receptors, second messenger changes, and G protein uncoupling may all play a role. Hyperalgesia, which may result from chronic administration, may be related to these same mechanisms.1,33 Respiratory Depression All opiate agonists produce a dose-dependent depression of respirations via effects on mu and delta receptors in the respiratory centers in the brainstem. They reduce the responsiveness within the respiratory centers to increasing carbon dioxide and decreasing oxygen levels. Thus higher partial pressure of carbon dioxide (Pco2) levels are required to maintain adequate respiration. Stated in pharmacologic terms, they produce a shift to the right in the CO2 response curve for respiration. Respiratory rate is affected first, and a classic “narcotized” patient will take slow deep breaths. As doses increase, apnea is produced. Because both analgesia and respiratory depression are mediated via the same receptors, reversal of respiratory depression with antagonists such as naloxone also reverses analgesia. The goal of most clinical anesthetics is to leave some residual analgesia without respiratory depression upon emergence to address postoperative pain.1 Miosis Miosis, or pinpoint pupils, is a prominent action of opioids and is usually present under general anesthesia as a result of the high doses of high-potency opiates used. Minimal tolerance develops to this effect. It is produced by effects in the pupillary reflex arc. Opiate depression of inhibitory γ-aminobutyric acid (GABA) interneurons leads CHAPTER 11 Opioid and Nonopioid Analgesics to stimulation of the Edinger-Westphal nucleus, which sends a parasympathetic signal via the ciliary ganglion to the oculomotor nerve to constrict the pupil.1 Clinicians note the degree of miosis as somewhat indicative of the presence of opiates. Antitussive Effects The opiates produce cough suppression via a depressant effect on the cough center in the medulla. Protective glottal reflexes are not affected. Although they all produce this effect, codeine and heroin are especially good suppressants. D-isomers such as dextromethorphan are also effective. The clinical use of opiates can help patients better tolerate airway devices and ventilators.36 Nausea and Vomiting The emetic effect of the opioids is complex. They elicit nausea and vomiting by stimulating the chemoreceptor trigger zone in the area postrema of the medulla. The dominant receptors in this area are serotonin type 3 (5-HT3) and dopamine type 2 (D2). A vestibular component is also probable because the incidence is much higher in ambulatory patients. Nausea and vomiting are rare in the preoperative area and operating room where patients are recumbent. A separate action with higher repeat doses can have an antiemetic effect by depressing the vomiting center. Clinically, when opiates are used as part of the anesthetic plan, there is an increased incidence of postoperative nausea and vomiting.37 The effect is less frequent with repeat doses although individual patient response is highly variable. Fig. 11.3 depicts the centers in the brain involved with nausea and vomiting and the types of receptors involved. Cardiac Effects The usual result of anesthetic use of opioids in healthy patients is bradycardia with little effect on blood pressure. Bradycardia results from medullary vagal stimulation.1 All opioids induce some degree of dose-dependent peripheral vasodilation. Myocardial contractility, baroreceptor function, and autonomic responsiveness are not affected. Opiate anesthesia is often used in patients with cardiovascular compromise because of this minimal depression. Much of the hypotension produced by morphine, codeine, and meperidine is attributed to histamine release, which is absent with fentanyl, sufentanil, alfentanil, and remifentanil. Histamine1 (H1) and H2 antagonists, when combined, block the cardiovascular effects of vasodilation, tachycardia, and hypotension that result.19 Opioids usually produce an antidiuretic effect. Opioids that are agonists at kappa receptors can cause diuresis. They decrease tone at the bladder detrusor muscle and constrict the urinary sphincter. This results in urinary retention. Urinary retention is a common side effect with intrathecal and epidural opioid administration. Muscle Rigidity Opioids have no major effects on nerve conduction at the neuromuscular junction or at the skeletal muscle membrane. A generalized hypertonus of skeletal muscle can be produced by large intravenous doses of most opioid agonists. Although morphine can produce rigidity, the problem is most often associated with fentanyl, alfentanil, sufentanil, and remifentanil. The difficulty is caused in part by loss of chest-wall compliance and by constriction of pharyngeal and laryngeal muscles. It becomes very difficult to ventilate the patient unless the rigidity is reversed. It is commonly referred to as tight chest or truncal rigidity. This effect most often occurs during anesthesia induction when high doses of potent opiates are administered rapidly. Remifentanil, which is administered by infusion, may be especially prone to producing this effect. Administration of nitrous oxide may increase the frequency.38 Opioid-induced muscle rigidity is thought to be mediated by central mu receptors interacting with dopamine and GABA pathways. This Fourth Ventricle Chemoreceptor Trigger Zone CHEMORECEPTOR TRIGGER ZONE (Area Postrema) Chemoreceptors D2 receptor NK1 receptor 5HT3 receptor VOMITING CENTER (Nucleus of Tractus Solitarius) Muscarinic (ACH) M1 receptor VESTIBULAR SYSTEM Muscarinic (ACH) M1 receptor HEART Mechanoreceptors 5HT3 receptor 143 Vomiting Center STOMACH Nausea, vomiting Vagus Nerve Fig. 11.3 The vomiting center and chemoreceptor trigger zone are shown. Inputs from sites outside the central nervous system are noted. Type and location of various receptors involved in nausea and vomiting are given. Antiemetic drugs act on these receptor targets. 5HT3, Serotonin; ACH, acetylcholine; D2, dopamine receptors; M1, muscarinic; NK1, neurokinin. 144 UNIT II Scientific Foundations rigidity is easily reduced or eliminated by the administration of neuromuscular blocking agents or naloxone.39 Pruritus Opiates frequently produce a rash, itching, and a feeling of warmth in the “blush” area of the face, upper chest, and arms. This occurs with both histamine and nonhistamine-releasing drugs and is especially prominent with neuraxial administration. The mechanism appears to be through central mu receptors and not local histamine release.40 Pruritus can be treated with antagonists such as naloxone or naltrexone; however, they also reverse analgesia. Nalbuphine, droperidol, antihistamines, and ondansetron may also be effective. Many clinical pain services have established protocols to treat this problem.41,42 The mixed μ/κ-opioid partial agonists seem to be the most effective medications that relieve neuraxial opioid-induced itch while preserving analgesia.43 In a 2016 review it was noted that nalbuphine is superior to placebo, diphenhydramine, naloxone, or propofol in treating opioid-induced pruritus and should be used as a first-line treatment.44 Gastrointestinal Effects Opioids have multiple effects on GI function. They decrease gastric motility and intestinal propulsive activity, prolong gastric emptying time, and reduce secretory activity throughout the GI system. This leads to the common problems of opiate-induced constipation and postoperative ileus. The pharmacologic treatment approach to this problem led to the development of peripherally acting μ-opioid receptor antagonists (PAMORAs). Naldemedine (Symproic), alvimopan (Entereg), methylnaltrexone (Relistor), and naloxegol (Movantik) are locally acting opiate receptor antagonists approved for use in treating these GI side effects. They do not cross biologic compartments due to solubility barriers and therefore work locally in the GI system but do not affect centrally produced analgesia.45,46 Lubiprostone (Amitiza), a bicyclic fatty acid that activates intestinal chloride channel-2 (ClC-2) in the small intestine, helps to increase fluid secretion and gut motility.47–49 Opioids produce a dose-dependent increase in biliary duct pressure and sphincter of Oddi tone via opioid receptor–mediated mechanisms. They also increase urinary sphincter tone. This has led to a few clinical concerns. The use of opiates for the treatment of biliary or renal colic may be compromised by this action. Meperidine, which produces this effect the least among opiate agonists or nonsteroidal antiinflammatory drugs (NSAIDs), is considered the agent of choice for this situation.50 In anesthesia, the use of opiates during surgery may produce false- positive cholangiograms. The clinical relevance of this effect is questionable since opiates are used routinely without problems. Endocrine Effects Opiates reduce the stress response to surgery and have an immunosuppressant effect.3 Endocrinologic effects of opioids include the release of vasopressin and inhibition of the stress-induced release of corticotropin and gonadotropins from the pituitary. Release of thyrotropin (i.e., thyroid stimulating hormone) from the adenohypophysis is also inhibited. Basal metabolic rate and temperature may also be decreased in patients receiving chronic opioids, although animal data indicate that acute administration can either systemically or intrathecally increase temperature. Opioids slightly decrease body temperature by resetting the equilibrium point of temperature regulation in the hypothalamus.1 Opioids and Cancer Although controversial, mounting data have implicated inhalational anesthesia and opioid analgesia as independent risk factors for cancer recurrence. Conversely, local-regional anesthesia and propofol-based total intravenous anesthesia have been promoted as having potential “chemopreventative” effects. The immune system plays an important role in controlling cancer. Factors that influence protective antitumor immunity have a significant impact on the course of malignant disease. Opioids are essential for the management of cancer pain, and preclinical studies indicate that opioids have the potential to influence tumor immune surveillance mechanisms. It has been suggested that opioids have a protumor action and may have negative effects on cancer survival. This is due to actions that may promote cancer cell proliferation and migration, immunosuppression, inflammation, and angiogenesis or neovascularization. Opioids also inhibit the function of natural killer cells. A definitive conclusion has not been reached. Opioid type and dose, the underlying disease pathology, and organs involved are important factors.51–53 See Chapter 46 for a further discussion of anesthesia and cancer recurrence. Neuraxial Effects The epidural and intrathecal routes have become common for the administration of pain medication. Opioids delivered by epidural or subarachnoid routes behave differently in onset, duration, and side effects than the same drugs given systemically. Pain that is unresponsive to systemic opioids may respond to the same drugs given centrally, reducing some side effects while increasing the incidence of others. Systemic opioids suppress nociception in lamina II and V cells of the dorsal horn of the spinal cord, leaving lamina IV and VI cells (which mediate nonnociceptive information) relatively unaffected.54 Spinal administration of opioids is a selective and potent means of producing analgesia. Intrathecal administration allows injection of the opioids directly into the cerebrospinal fluid (CSF), a more efficient method of delivering the drug to the spinal cord opiate receptors. The analgesic response is the result of activity at spinal opiate receptors, especially kappa receptors in the substantia gelatinosa, lamina II of the dorsal horn.54 Opioids can be given with local anesthetics or other adjuncts intraoperatively at the initiation of spinal anesthesia or postoperatively for pain control.55 Side effects with spinal administration are similar to those described previously with systemic administration, except that pruritus and urinary retention occur with much greater frequency. Less lipid-soluble agents such as morphine and hydromorphone produce a delayed ventilatory depression, the result of migration of opioid via the CSF to the midbrain vestibular centers. Respiratory depression is the most common serious complication associated with intrathecally and epidurally administered opioids. Two different levels of respiratory depression can occur after neuraxial morphine administration. An early phase observed soon after administration reflects rapid systemic absorption and is similar to parenteral dosing. A later, more insidious depression that occurs over a period of 8 to 12 hours has been related to rostral flow of CSF and delivery of morphine to the brainstem respiratory center.56 Awareness of delayed respiratory depression has resulted in increased monitoring of patients and dose reductions, thereby greatly reducing the incidence of serious respiratory depression to that seen with patient-controlled analgesia (PCA) opioids. Generalized pruritus has been observed with neuraxial morphine and to a lesser extent with other opioids. Mild itching, usually involving the face or chest, is common; however, the intensity of itching can become so annoying that it interferes with sleep. Pruritus is commonly seen with opioids that do not release histamine, such as fentanyl and sufentanil. Pruritus can be treated with antihistamines or with opiate receptor partial agonists or antagonists. The incidence of postoperative nausea and vomiting increases for patients treated with neuraxial opioids. Nausea may result either from the rostral spread of the drug in the spinal fluid to the brainstem or vascular uptake and delivery to the chemoreceptor trigger zone in the area postrema of the medulla. CHAPTER 11 Opioid and Nonopioid Analgesics Urinary retention after spinal opioid analgesia has been related to inhibition of sacral parasympathetic outflow, which results in relaxation of the bladder detrusor muscle and an inability to relax the sphincter.54 Opioids with a higher lipid solubility (see Table 11.3) tend to be rapidly absorbed into the spinal tissues after central administration, resulting in a faster onset of action. However, higher lipid solubility is associated with a small area of distribution of the drug along the length of the spinal cord and therefore a more limited area of analgesia.4 Higher lipid solubility is also associated with faster clearance of the drug out of the epidural and intrathecal space, resulting in a shorter duration of action and higher blood concentrations of the opioid.54,56 Spinal opioids are advantageous in selective analgesia, which occurs in the absence of motor and sympathetic blockade. Epidural anesthesia and analgesia have been successfully used in obstetric patients and surgical patients. Epidural doses of opioids, however, are much higher than doses of opioids for intrathecal use. Small portions of epidural opioids cross the dura, enter the CSF, and penetrate spinal tissue in amounts proportional to their lipid solubility. The remaining drug is absorbed by the vasculature, producing plasma levels comparable to those after intramuscular injections and providing supraspinal analgesia.56 Neuraxial administration of opioids is a selective and potent means of producing analgesia in many surgical and obstetric procedures.57 Table 11.4 gives neuraxial opioid dosages.54,56 Opioid Techniques and Delivery In clinical practice, opioids are used to relieve pain during monitored anesthesia care, as regional anesthesia, and as a component of balanced anesthesia, as well as a primary component of general anesthesia. The inclusion of opioids reduces pain and anxiety, decreases somatic and autonomic responses to airway manipulation, improves hemodynamic stability, lowers requirements for inhaled anesthetic agents, and provides postoperative analgesia.58 The most common method of administering opioids in the practice of anesthesia is intermittent bolus injection, which, although effective for many procedures, produces wide swings in drug plasma concentration. Intermittent periods of deep and light anesthesia are produced. Continuous opioid infusion results in plasma concentration that can be maintained more accurately and consistently. Continuous infusion of opioids is associated with hemodynamic stability, reduces the total necessary dose of opioids, and decreases the need for opioid reversal agents. TABLE 11.4 Doses of Neuraxial Opioids* Opioid Single Dose Infusion Rate Epidural Morphine Meperidine Methadone Fentanyl Sufentanil Butorphanol 2–5 mg 25–50 mg 5 mg 50–100 mcg 25–50 mcg 2–4 mg 0.1–1 mg/h 5–20 mg/h 0.3–0.5 mg/h 25–100 mcg/h 10–50 mcg/h 0.2–0.4 mg/h Subarachnoid Morphine Meperidine Fentanyl 0.25–0.3 mg 10 mg 10–20 mcg Sufentanil 5–10 mcg *Doses adjusted for age and level of regional injection. 145 Continuous intravenous administration involves the infusion of a loading dose that fills the volume of distribution, followed by continuous drug replacement that keeps the volume of distribution filled as the drug is eliminated.59 Loading dose (mcg/kg) = Vd (mL/kg) × Cp (mcg/mL) Loading dose (mcg/kg) = Vd (mL/kg) × Cp (mcg/mL) where Vd is volume of distribution, Cp is plasma concentration, and Cl is drug clearance. See Table 11.3 for the Vd and Cl of various opioids. The rate of continuous infusion does not remain constant, but rather is adjusted to meet the patient’s needs and to control varying surgical stimuli. The volume of distribution is decreased for patients with hypovolemia and trauma, and for geriatric patients. Factors such as enzyme induction, hepatic failure, and adjunctive drug use are also considered. Table 11.5 provides dose ranges for continuous infusions. Continuous intravenous infusion can be administered by gravity flow with a manual control device (e.g., Buretrol), an infusion pump, or a syringe pump. Syringe pumps are advantageous because they are programmed to administer drug in units of micrograms per kilogram per minute. Some advantages of continuous infusion techniques are listed in Box 11.4. Nonparenteral routes of opioid delivery are also used. Fentanyl is the prototypic opioid for transdermal application. Transdermal administration of fentanyl does not require cooperation from the patient; in addition, first-phase hepatic metabolism is not a factor, and the route does not produce discomfort. Currently available formulations permit delivery of 25 to 100 mcg/hour for 24 to 72 hours. The transdermal fentanyl patch provides a relatively constant plasma concentration for 72 hours. It is not currently recommended for use in managing postoperative pain. Rapid-onset opioids provide new options for effective management of pain. There are various formulations of transmucosal immediate-release fentanyl and, although they were originally developed and approved for use in children before painful procedures, are now used in opioid- tolerant adult patients with breakthrough cancer pain. The formulation options include oral lozenge, buccal tablet, buccal film, sublingual tablet, nasal spray, and a sublingual spray; each has practical considerations that TABLE 11.5 Infusion Rates* Opioid Induction (mcg/kg) Maintenance (mcg/kg/min) Fentanyl 5–10 0.01–0.05 Sufentanil Alfentanil 1–30 8–100 0.005–0.015 0.5–3 Remifentanil 0.5–1 0.05–2 *Doses will vary depending on coadministered drugs, patient characteristics, and surgical procedure. BOX 11.4 Advantages of Continuous Opioid Infusion • • • • • Hemodynamic stability Decreased side effects Reduced need for opioid-reversal agents Reduced use of vasopressor drugs Suppression of cortisol and vasopressin response to cardiopulmonary bypass: stress-free anesthesia • Reduced total dosage of opioids • Decreased recovery time 146 UNIT II Scientific Foundations vary with the product and route of administration.60,61 All have the common advantage of rapid entry into the systemic circulation via transmucosal absorption, avoiding hepatic and intestinal first-pass metabolism, which allows for a rapid onset of action that rivals intravenous injections. A high incidence of pruritus is a problematic side effect with transmucosal administration. Fentanyl buccal tablet (Fentora) is indicated for the management of breakthrough pain in cancer patients who are tolerant to 60 mg or greater of oral morphine equivalents.62 The Food and Drug Administration (FDA) has approved a nasal spray formulation of fentanyl (Lazanda) for management of breakthrough pain in adult cancer patients who are already receiving and are tolerant to opioid therapy. Nasal administration of sufentanil preoperatively in the pediatric patient has also been studied. After administration, the children remained calm, and some experienced somewhat decreased ventilatory compliance. Recovery room time was not increased, and the highest incidence of nausea and vomiting occurred in the group that received the highest dose of sufentanil. Nasal butorphanol is currently available and is widely used in the management of migraine headaches. Table 11.6 compares dosages for various routes of opioid administration.63 INDIVIDUAL OPIOIDS Agonists Naturally Occurring Opioids Morphine. Morphine, the prototype for opioid agonists, is the most abundant alkaloid in raw opium. The primary therapeutic use TABLE 11.6 Opioid Dose Comparisons Opioid Route Onset Peak Duration of Action Half-Life Morphine` PO 60 min 30–60 min 4–5 hr Neonates 4.5–13 hr IM IV Epidural PO IM IV PO PO IV PO IM IV IV Transmucosal IM IV Epidural IV IV PO IM IV IM IV IM IV IM IV PO IM IV IM IV IM IV 30–60 min 20 min 60–90 min 30–60 min 10–30 min 15–30 min 10–15 min 30–60 min 5–10 min 10–15 min 10–15 min 5 min Immediate 5–15 min 7–15 min 2–5 min 20–30 min 1 min 1–3 min 60 min 10–30 min 10–30 min 10–15 min Immediate 15–30 min 15–30 min 30–60 min 30–60 min 30–60 min 60–90 min 30–60 min 30–90 min 30–60 min 30–60 min 15–20 min 30–60 min 30–60 min 30–60 min Immediate 20–30 min 20–30 min 20–30 min 4–5 hr 4–5 hr 8–24 hr 4–6 hr 4–6 hr 4–5 hr 4–5 hr 6–8 hr 4–6 hr 2–4 hr 2–4 hr 2–4 hr Adults 3–5 hr 15–30 min 15–30 min 2–3 min 5 min 2 min 5–15 min 2 min 5–15 min 5–15 min 120 min 2–3 min 4–5 hr 2–3 hr 2–3 hr 20–60 min 20–60 min 8 hr 8 hr PO 45–60 min 60 min 24–72 hr Codeine Hydromorphone Oxycodone Methadone Meperidine Alfentanil Fentanyl Remifentanil Sufentanil Tramadol Buprenorphine Butorphanol Dezocine Nalbuphine Pentazocine Naloxone Nalmefene Naltrexone IM, Intramuscularly; IV, intravenously; PO, by mouth. 1 min 120 min 60 min 60 min 30–60 min 30–60 min 60 min 60 min 30 min 1–3 min Related to blood levels 1–2 hr 0.5–1 hr 2–3 hr 5–10 min Dose dependent 9 hr 6 hr 6 hr 3–4 hr 3–4 hr 4–6 hr 4–6 hr 2.5–3.5 hr 2.5–3.5 hr 1–3 hr 3.2–4.5 hr 15–30 hr 15–30 hr 2.5–4 hr 2.5–4 hr 2.5–4 hr 1.5 hr 6.6 hr 2–4 hr 2–4 hr 2–4 hr 9 min 6 hr 2–3 hr 2–3 hr 2.5–4 hr 2.5–4 hr 2.6–2.8 hr 2.6–2.8 hr 3.5–5 hr 3.5–5 hr 2–3 hr 2–3 hr 2–3 hr Neonates 1.2–3 hr Adults 1–1.5 hr 10.8 hr 10.8 hr 6–10 hr CHAPTER 11 Opioid and Nonopioid Analgesics of morphine is the abatement of moderate to severe pain. It is more effective in relieving continuous dull pain than sharp intermittent pain. Morphine can be administered via the intramuscular, intravenous, subcutaneous, oral, intrathecal, and epidural routes. Effects of intravenous morphine on the time course of sedation and analgesia occur with sedation first, followed by analgesia.64 Morphine- induced sedation therefore should not be considered as an indicator of appropriate analgesia. When given intrathecally, morphine has the longest duration of action of the specific opioids. Morphine is among the least lipophilic of the opioids, resulting in slow penetration of biologic membranes, less accumulation in lipid membranes or fatty tissues, and slower onset. Morphine undergoes phase 2 glucuronide conjugation in the liver at both the 3 position (which produces morphine- 3- glucuronide [M3G]) and the 6 position (which produces morphine-6-glucuronide [M6G]).65 As a result of the active metabolite, M6G, morphine appears to produce a more prolonged effect, often excessive sedation, in the patient with renal failure. Within the CNS, M6G metabolite is more potent than the parent drug, whereas M3G metabolite is inactive.65,66 The greater hydrophilicity of M6G than the parent drug normally impedes its passage into the CNS. However, after chronic administration or in patients with renal failure, M6G (at a high blood level) can enter the CNS. Morphine produces a nonimmunologic release of histamine from tissue mast cells, resulting in local itching, redness, or hives near the site of intravenous injection or generalized flushing. When sufficient histamine is released, the patient may exhibit signs of decreased systemic vascular resistance, hypotension, and tachycardia. Localized histamine release after a morphine injection is not uncommon. Morphine is primarily used for preoperative or postoperative pain relief. The delayed onset and peak effects and large patient variability make it less useful for intraoperative use than fentanyl and its analogs. Codeine. Considered a weak opioid, codeine is generally not used for treatment of severe pain. Approximately 10% of the administered dose of codeine is O-demethylated to morphine, which accounts for most of its analgesic activity.57 It has good antitussive activity, but on a weight basis, codeine is a less potent antitussive than morphine. Combinations of codeine with acetaminophen remain very popular as prescribed analgesics. Codeine is contraindicated in children due to the possibility of excessive respiratory depression. Codeine toxicity due to extensive conversion of the prodrug into morphine is discussed in the pharmacogenetics section and in Chapter 6. Semisynthetic Opioids Hydromorphone. Derived from morphine in the 1920s, hydromorphone has a pharmacokinetic profile similar to that of morphine, but it is more potent. Hydromorphone is absorbed from the oral, rectal, and parenteral sites. Because of its lipid solubility, it is sometimes used instead of morphine for epidural or spinal administration when a wide area of analgesia is needed.67 Studies performed on parenteral hydromorphone relative to morphine tend to demonstrate similar analgesia and side effect profiles. Because of the lack of any known active metabolites, it is often recommended for patients with renal failure.1 It is available in high potency and sustained release preparations. Synthetic Opioids Methadone. Introduced in the 1940s, methadone is used primarily for relief of chronic pain, treatment of opioid abstinence syndromes, and treatment of opioid use disorders. Compared to other common opioids, it is well absorbed orally and produces less euphoria. Supplied as a racemic mixture of two optical isomers, most of methadone’s 147 activity comes from the l-isomer. Unlike most opioids, it has a long half-life, allowing for less frequent dosing. It has a prolonged effect in part due to extensive protein binding (90%) with slow release and a lower intrinsic ability of the liver to metabolize it. It also has the advantage of a high bioavailability and no active metabolites. Disadvantages include accumulation and a longer time to reach steady state than other opioids.68 Meperidine. Meperidine is a synthetic mu receptor agonist. It is structurally similar to atropine and has an atropine-like antispasmodic effect. After demethylation in the liver, meperidine is partially metabolized to normeperidine, which is half as analgesic as meperidine but lowers the seizure threshold and induces CNS excitability. Normeperidine’s elimination half-life is significantly longer than that of meperidine. With accumulation of normeperidine, subjects may experience a CNS excitation characterized by tremors, muscle twitches, and seizures. Due to accumulation of normeperidine, limitations on its use should be considered in patients with renal failure, the elderly, and for chronic use in cancer patients who may require high doses. There is a significant drug interaction that can occur between meperidine and the first- generation monoamine oxidase (MAO)–inhibiting drugs isocarboxazid (Marplan, others), phenelzine (Nardil, others), and tranylcypromine (Parnate, others). Hyperthermia, seizures, and death have been reported.60–71 See Chapter 14 for further discussion of meperidine drug interactions. Meperidine is effective in reducing shivering from diverse causes, including general and epidural anesthesia.72 This appears to result from kappa receptor stimulation. It reduces or eliminates visible shivering and the accompanying increase in oxygen consumption.33 Anesthetic uses of meperidine have declined in recent years due to the availability of safer, more convenient techniques. Alfentanil. After bolus injection, alfentanil has a more rapid onset of action and shorter duration than fentanyl, even though it is less lipid soluble. The high nonionized fraction (90%) of alfentanil at physiologic pH and its small volume of distribution increase the amount of drug available for binding in the brain. Although alfentanil is effective epidurally, the duration of analgesia is short, and for this reason it has never achieved popularity. Alfentanil is metabolized in the liver by oxidative N-dealkylation and O-demethylation in the cytochrome P-450 system, and the inactive metabolites are excreted in the urine. Alfentanil has great patient- to- patient variability as seen in the original studies, in which a high coefficient of variation was reported. Erythromycin has been shown to prolong the metabolism of alfentanil and interact with alfentanil to produce clinical symptoms of prolonged respiratory depression and sedation. Its popularity in current practice is limited.33 Fentanyl. Fentanyl is the most widely used opioid analgesic in anesthesia. A single administered dose of fentanyl has a short duration of action (approximately 20–40 minutes). It produces a profound dose- dependent analgesia, ventilatory depression, and sedation. The action of a single dose of fentanyl is terminated by redistribution. The high lipid solubility of fentanyl allows for rapid tissue uptake.19 Fentanyl and its derivatives all undergo significant first-pass uptake in the lungs with temporary accumulation before release. When fentanyl is given in multiple doses or as a continuous infusion, the termination of action reflects elimination but not redistribution. Clearance of fentanyl is dependent on hepatic blood flow. Fentanyl is metabolized by N-dealkylation and hydroxylation to inactive metabolites that are eliminated in urine and bile. Fentanyl elimination is prolonged in the elderly and the neonate. Initially used intravenously during surgery, fentanyl has many other uses. It is administered for intrathecal, epidural, and postoperative PCA intravenous use. Fentanyl transdermal patches deliver 75 to 148 UNIT II Scientific Foundations 100 mcg/hour, resulting in peak plasma concentrations in approximately 18 hours because a subcutaneous depot of drug must be saturated before the drug is consistently absorbed into the bloodstream.19 The dose remains stable during the presence of the patch. After removal, the decline in blood concentration follows an apparent 17-hour half-life; the true elimination half-life of fentanyl remains at approximately 3 hours, but continued absorption from the subcutaneous depot during elimination makes it appear longer. Remifentanil. Remifentanil use in anesthesia has increased as new applications are being discovered for its unique profile.73,74 Its rapid onset and ultrashort duration, titratability, and simple metabolism make it very convenient for many modern clinical perioperative situations. Remifentanil is a moderately lipophilic, piperidine-derived opioid with an ester link. The addition of the ester group allows the drug to be easily and rapidly metabolized by blood and tissue esterases. Kinetic studies indicate that the drug has a small volume of distribution (Vd 0.39 ± 0.25) and an elimination half-life of 8 to 20 minutes. It is metabolized by hydrolysis catalyzed by general esterase enzymes to a less active compound. It is not dependent on cholinesterase enzyme for metabolism, and therefore it is not influenced by quantitative or qualitative changes in cholinesterase. Succinylcholine metabolism does not influence remifentanil breakdown. Due to the potential for respiratory depression and muscle rigidity, bolus dosing in the preoperative or postoperative care unit is not recommended. Due to its unique metabolic pathway, remifentanil has a short duration of action, a precise and rapid titratable effect because of rapid onset, and noncumulative effects and results in rapid recovery after discontinuation of its administration by infusion. However, because remifentanil has such a prompt offset, it is important to begin alternative analgesic therapy in the postoperative period.75 The commercial preparation of remifentanil is a water-soluble, lyophilized powder that contains a free base and glycine as a vehicle to buffer the solution. Because of potential glycine neurotoxicity, remifentanil should not be administered epidurally or intrathecally.1 Sufentanil. Sufentanil is the most potent of the phenylpiperidines and is used for situations in which profound analgesia is required, such as in cardiac or other major surgical procedures. The patients are usually in-hospital patients requiring significant analgesia and postoperative care. Sufentanil is a μ-agonist that produces effective analgesia via both the intravenous and intrathecal routes. It is highly lipophilic and potent, with a shorter elimination half-life than fentanyl. Hepatic clearance of sufentanil approaches liver blood flow. Sufentanil metabolism involves O- demethylation and N- dealkylation, with minimal amounts being excreted unchanged in the urine. The effects of age on the distribution and elimination of sufentanil are reflected in a decrease in the initial volume of distribution for the elderly. The reduced volume of distribution of sufentanil in elderly patients is associated with increased respiratory depression.33 Tramadol. Tramadol is a synthetic codeine analog that is a weak μ- opioid receptor agonist, with analgesic effects produced by inhibition of norepinephrine and serotonin neuronal reuptake, as well as presynaptic stimulation of 5- hydroxytryptamine release. Tramadol is a racemic mixture; the (+) enantiomer binds to the mu receptor and inhibits serotonin uptake, whereas the (−) enantiomer inhibits norepinephrine uptake and stimulates α2-adrenergic receptors. It has an elimination half-life of 5 to 6 hours and is an effective analgesic for the treatment of mild to moderate pain. Trama