Patterns of Single-Gene Inheritance 2024 PDF
Document Details
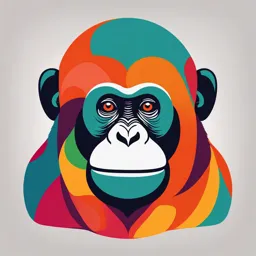
Uploaded by ToughestChlorine
2024
Akhobadze Madona
Tags
Summary
This presentation details patterns of single-gene inheritance, including Mendelian inheritance, factors affecting pedigree patterns, and genotype-phenotype correlations. It also covers various types of genetic diseases and their characteristics. The presentation is meant for a postgraduate audience and includes various diagrams to help better visualize the points made.
Full Transcript
Akhobadze Madona M.D. PhD assistant ☺ Patterns of Single-Gene Inheritance 2024 Variation in Genes Genotype and Phenotype Pedigrees Mendelian Inheritance Factors Affecting Pedigree Patterns Correlating Genotype And Phenotype Autosoma...
Akhobadze Madona M.D. PhD assistant ☺ Patterns of Single-Gene Inheritance 2024 Variation in Genes Genotype and Phenotype Pedigrees Mendelian Inheritance Factors Affecting Pedigree Patterns Correlating Genotype And Phenotype Autosomal Patterns Of Mendelian Inheritance Reading: Ch. 7. Thompson & Thompson Genetics in Medicine, Robert L. Nussbaum, Roderick R. McInnes TYPES OF GENETIC DISEASES single-gene, chromosomal, and complex Single-gene traits caused by mutations in genes in the nuclear genome are often called mendelian because, like the characteristics of garden peas studied by Gregor Mendel, they occur on average in fixed proportions among the offspring of specific types of matings. Mendelian Inheritance The number of diseases with known genetic causes and the number of genes in which mutations can cause disease are not the same because different mutations in the same gene can cause different diseases, and mutations in different genes can cause similar or indistinguishable diseases. TYPES OF GENETIC DISEASES Chromosome disorders, in which entire chromosomes (or large segments of them) are missing, duplicated, or otherwise altered. These disorders include diseases such as Down syndrome and Turner syndrome Disorders in which single genes are altered; these are often termed mendelian conditions, or single-gene disorders. Well-known examples include cystic fibrosis, sickle cell disease, and hemophilia Multifactorial disorders, which result from a combination of multiple genetic and environmental causes. Many birth defects, such as cleft lip and cleft palate, as well as many adult disorders, including heart disease and diabetes, belong in this category Mitochondrial disorders, a relatively small number of diseases caused by alterations in the small cytoplasmic mitochondrial chromosome Single-gene disorders are often considered to be primarily but by no means exclusively disorders of the pediatric age range; less than 10% manifest after puberty, and only 1% occur after the end of the reproductive period the mendelian forms are important in individual patients because of their significance for the health of other family members and because of the availability of genetic testing and detailed management options for many of them Variation in Genes Inherited variation in the genome is the cornerstone of human and medical genetics segment of DNA occupying a particular position or location on a chromosome is a locus If the segment contains a gene, that DNA segment is the locus for that gene. Alternative variants of a gene are called alleles The other versions of the gene are variant or mutant alleles that differ from the wild-type allele because of the presence of a mutation, a permanent change in the nucleotide sequence or arrangement of DNA given set of alleles at a locus or cluster of loci on a chromosome is referred to as a haplotype Variation in Genes If there are at least two relatively common alleles at the locus in the population, the locus is said to exhibit polymorphism (literally “many forms”) The term mutation is used in medical genetics in two senses: sometimes to indicate a new genetic change that has not been previously known in a family, and sometimes merely to indicate a disease-causing mutant allele. Mutation and mutant, however, are never used to refer to the human beings who carry mutant alleles Genotype and Phenotype The genotype of a person is the set of alleles that make up his or her genetic constitution, either collectively at all loci or, more typically, at a single locus the phenotype is the observable expression of a genotype as a morphological, clinical, cellular, or biochemical trait. The phenotype is usually thought of as the presence or absence of a disease, but phenotype can refer to any manifestation, including characteristics that can be detected only by blood or tissue testing. A phenotype may, of course, be either normal or abnormal in a given individual Genotype and Phenotype abnormal phenotypes—genetic disorders Although each gene usually encodes a polypeptide chain or RNA molecule, a single abnormal gene or gene pair often produces multiple diverse phenotypic effects and determines which organ systems are involved, which particular signs and symptoms occur, and when they occur. Under these circumstances, the expression of the gene defect is said to be pleiotropic Genotype and Phenotype A single-gene disorder is one that is determined primarily by the alleles at a single locus. When a person has a pair of identical alleles at a locus encoded in nuclear DNA, he or she is said to be homozygous (a homozygote); when the alleles are different, he or she is heterozygous (a heterozygote or carrier) Genotype and Phenotype The term compound heterozygote is used to describe a genotype in which two different mutant alleles of the same gene are present, rather than one normal and one mutant These terms (homozygous, heterozygous, and compound heterozygous) can be applied either to a person or to a genotype In the special case in which a male has an abnormal allele for a gene located on the X chromosome and there is no other copy of the gene, he is neither homozygous nor heterozygous and is referred to as hemizygous Genotype and Phenotype Mitochondrial DNA is still another special case. In contrast to the two copies of each gene per diploid cell, mitochondrial DNA molecules, and the genes encoded by the mitochondrial genome, are present in tens to thousands of copies per cell. For this reason, the terms homozygous, heterozygous, and hemizygous are not used to describe genotypes at mitochondrial loci Pedigrees Single-gene disorders are characterized by their patterns of transmission in families. To establish the pattern of transmission, a usual rst step is to obtain information about the family history of the patient and to summarize the details in the form of a pedigree, a graphical representation of the family tree The extended family depicted in such pedigrees is a kindred. The member through whom a family with a genetic disorder is rst brought to the attention of the geneticist is the proband if he or she is affected. The person who brings the family to attention by consulting a geneticist is referred to as the consultand; the consultand may be an affected individual or an unaffected relative of a proband Brothers and sisters are called sibs Pedigrees Relatives are classied as rst degree - parents, sibs, and offspring of the proband, second degree - grandparents and grandchildren, uncles and aunts, nephews and nieces, and half-sibs third degree - rst cousins), and so forth, depending on the number of steps in the pedigree between the two relatives. The offspring of rst cousins are second cousins, and a child is a “rst cousin once removed” of his or her parents’ rst cousins Pedigrees Couples who have one or more ancestors in common are consanguineous. If there is only one affected member in a family, he or she is an isolated case if the disorder is determined to be due to new mutation in the propositus, a sporadic case Pedigrees In many disorders, whether or not a condition will demonstrate an obvious familial pattern of transmission in families depends on whether individuals affected by the disorder can reproduce Geneticists coined the term tness as a measure of the impact of a condition on reproduction Fitness - the reproductive success of a genotype - usually measured as the number of offspring produced by an individual that survive to reproductive age relative to the average for the population Pedigrees Fitness is not a measure of physical or mental disability. For example, in some disorders, an affected individual can have normal mental capacities and health but yet have a tness of 0 because the condition interferes with normal reproduction. In other cases, a severe, debilitating genetic disorder may have normal tness because the onset of the disease is well past the usual reproductive age MENDELIAN INHERITANCE The patterns shown by single-gene disorders in pedigrees depend chiey on two factors: whether the phenotype is dominant (expressed when only one chromosome of a pair carries the mutant allele and the other chromosome has a wild-type allele at that locus) or recessive (expressed only when both chromosomes of a pair carry mutant alleles at a locus); the chromosomal location of the gene locus, which may be on an autosome (chromosomes 1 to 22) or on a sex chromosome (chromosomes X and Y) Thus, there are four basic patterns of single-gene inheritance : Autosomal and -Linked Inheritance Whether an abnormal gene is on an autosome or is X linked has a profound effect on the clinical expression of the disease. First, autosomal disorders, in general, affect males and females equally. For X-linked disorders, the situation is quite different. Males have only a single X and are therefore hemizygous with respect to X-linked genes; 46,XY males are never heterozygous for alleles at X linked loci, whereas females can be heterozygous or homozygous at X-linked loci. Second, to compensate for the double complement of X-linked genes in females, alleles for most X-linked genes are expressed from only one of the two X chromosomes in any given cell of a female Dominant Inheritance A phenotype expressed in both homozygotes and heterozygotes for a mutant allele is inherited as a dominant Dominant disorders occur whether or not there is normal gene product made from the remaining normal allele. In a pure dominant disease, homozygotes and heterozygotes for the mutant allele are both affected equally Pure dominant disorders rarely if ever exist in medical genetics Dominant Inheritance On occasion, phenotypic expression of two different alleles for a locus occurs, in which case the two alleles are termed codominant. One well-known example of codominant expression is the ABO blood group system. Most commonly, dominant disorders are more severe in homozygotes than in heterozygotes, in which case the disease is called incompletely dominant (or semidominant) Recessive Inheritance As classically dened, a phenotype expressed only in homozygotes (or, for X-linked traits, male hemizygotes) and not in heterozygotes is recessive Most of the recessive disorders described to date are due to mutations that reduce or eliminate the function of the gene product, so-called loss-of-function mutations Typical pedigree showing autosomal recessive inheritance Recessive Inheritance For example, many recessive diseases are caused by mutations that impair or eliminate the function of an enzyme. These are usually inherited as recessive diseases because heterozygotes, with only one of a pair of alleles functioning and the other (abnormal) allele not, can typically make sufcient product (∼50% of the amount made by wild-type homozygotes) to carry out the enzymatic reaction required for normal physiological function, thereby preventing disease FACTORS AFFECTING PEDIGREE PATTERNS Penetrance is the probability that a gene will have any phenotypic expression at all. When the frequency of expression of a phenotype is less than 100%—that is, when some of those who have the appropriate genotype completely fail to express it—the gene is said to show reduced penetrance. It is the percentage of people with a predisposing genotype who are actually affected, at least to some degree FACTORS AFFECTING PEDIGREE PATTERNS Expressivity is the severity of expression of the phenotype among individuals with the same disease causing genotype. When the severity of disease differs in people who have the same genotype, the phenotype is said to have variable expressivity. Even in the same kindred, two individuals carrying the same mutant genes may have some signs and symptoms in common, whereas their other disease manifestations may be quite different, depending on which tissues or organs happen to be affected Pedigree of split-hand deformity demonstrating failure of penetrance in the mother of the consultand (arrow) Reduced penetrance must be taken into account in genetic counseling FACTORS AFFECTING PEDIGREE PATTERNS Age at Onset Genetic disorders can appear at any time in the lifetime of an individual, ranging from early in intrauterine development all the way to the post reproductive years, and all ages in between. Some may be lethal prenatally, whereas others may interfere with normal fetal development and can be recognized prenatally (e.g., by ultrasonography) but are consistent with a full-liveborn infant; still others may be recognized only at birth (congenital) FACTORS AFFECTING PEDIGREE PATTERNS The terms genetic and congenital are frequently confused. genetic disorder is one that is determined by genes, whereas a congenital disorder is merely one that is present at birth and may or may not have a genetic basis CORRELATING GENOTYPE AND PHENOTYPE When a genetic disorder that appears to be inherited as a single-gene disorder is thoroughly analyzed, it is frequently found to be genetically heterogeneous; that is, it includes a number of phenotypes that are similar but are actually determined by different genotypes at different loci Genetic heterogeneity may be the result of different mutations at the same locus (allelic heterogeneity), mutations at different loci (locus heterogeneity), or both CORRELATING GENOTYPE AND PHENOTYPE Recognition of genetic heterogeneity is an important aspect of clinical diagnosis and genetic counseling. On the other hand, distinct phenotypes inherited in different families can result from different mutant alleles in the same gene. This phenomenon, known as clinical or phenotypic heterogeneity, is well known and must be taken into account in correlating genotype and phenotype Allelic Heterogeneity Allelic heterogeneity is an important cause of clinical variation. Many loci possess more than one mutant allele; in fact, at a given locus, there may be several or many mutations As one example, nearly 1400 different mutations have been found worldwide in the cystic brosis transmembrane conductance regulator (CFTR) among patients with cystic brosis. Sometimes, these different mutations result in clinically indistinguishable disorders Allelic Heterogeneity In other cases, different mutant alleles at the same locus produce a similar phenotype but along a continuum of severity; for example, some CFTR mutations cause patients to have classic cystic brosis with pancreatic insufciency, severe progressive lung disease, and congenital absence of the vas deferens in males, whereas patients carrying other mutant alleles have lung disease but normal pancreatic function, and still others have only the abnormality of the male reproductive tract. Since any particular mutant allele is generally uncommon in the population, most people with rare autosomal recessive disorders are compound heterozygotes rather than true homozygotes Locus Heterogeneity For many phenotypes, pedigree analysis alone has been sufcient to demonstrate locus heterogeneity For example, retinitis pigmentosa, a common cause of visual impairment due to photoreceptor degeneration, has long been known to occur in autosomal dominant, autosomal recessive, and X-linked forms. In recent years, the heterogeneity has been shown to be even more extensive; pedigree analysis combined with gene mapping has demonstrated that there are at least 43 loci responsible for 5 X-linked forms, 14 autosomal dominant forms, and 24 autosomal recessive forms of retinitis pigmentosa that are not associated with other phenotypic abnormalities. If one includes disorders in which retinitis pigmentosa is found in conjunction with other defects such as mental retardation or deafness, there are nearly 70 different genetic diseases manifesting retinitis pigmentosa. Phenotypic Heterogeneity Different mutations in the same gene can sometimes give rise to strikingly different phenotypes. For example, certain loss-of-function mutations in the RET gene, which encodes a receptor tyrosine kinase, can cause dominantly inherited failure of development of colonic ganglia, leading to defective colonic motility and severe chronic constipation (Hirschsprung disease) Other mutations in the same gene result in unregulated hyperfunction of the kinase, leading to dominantly inherited cancer of the thyroid and adrenal glands (multiple endocrine neoplasia type 2A and 2B) A third group of mutations in RET causes both Hirschsprung disease and multiple endocrine neoplasia in the same individuals Sex-Inuenced Disorders Since males and females both have the same complement of autosomes, autosomal recessive disorders generally show the same frequency and severity in males and females. There are, however, exceptions Some autosomal recessive phenotypes are sex-inuenced, that is, expressed in both sexes but with different frequencies or severity. Among autosomal disorders, hemochromatosis is an example of a phenotype more common in males Sex-Inuenced Disorders This autosomal recessive disorder of iron metabolism occurs most commonly in the approximately 0.5% of individuals of northern European extraction that are homozygous for a missense mutation replacing cysteine at position 282 with a tyrosine (Cys282Tyr) in the HFE gene. Cys282Tyr homozygotes have enhanced absorption of dietary iron and often demonstrate laboratory abnormalities suggestive of excessive body stores of iron, although the condition only rarely leads to iron overload and serious damage to the heart, liver, and pancreas. The lower incidence of the clinical disorder in females (one fth to one tenth that of males) is believed to be related, among other factors, to lower dietary intake of iron, lower alcohol usage, and increased iron loss through menstruation among females. Consanguinity It is believed that everyone carries at least 8 to 10 mutant alleles, of which perhaps half are lethal in homozygotes before birth. The remainder cause well-known, easily recognizable autosomal recessive disorders in homozygotes The chance that both parents are carriers of a mutant allele at the same locus is increased substantially if the parents are related and could each have inherited the mutant allele from a single common ancestor, a situation called consanguinity Consanguinity is dened arbitrarily as a union of individuals related to each other as close as or closer than second cousins Consanguinity Consanguinity of the parents of a patient with a genetic disorder is strong evidence (although not proof) for the autosomal recessive inheritance of that condition For example, the disorder in the pedigree is likely to be an autosomal recessive trait, even though other information in the pedigree may seem insufcient to establish this inheritance pattern Consanguinity The genetic risk to the offspring of marriages between related people is not as great as is sometimes imagined For marriages between rst cousins, the absolute risks of abnormal offspring, including not only known autosomal recessive diseases but also stillbirth, neonatal death, and congenital malformations, is 3% to 5%, about double the overall background risk of 2% to 3% for offspring born to any unrelated couple Consanguinity Consanguinity at the level of third cousins or more remote relationships is not considered to be genetically signicant, and the increased risk of abnormal offspring is negligible in such cases. Although the incidence of cousin marriage is low (∼1 to 10 per 1000) in many populations in Western societies today, it remains relatively common in some ethnic groups, for example, in families from rural areas of the Indian subcontinent, in other parts of Asia, and in the Middle East, where between 20% and 60% of all marriages are between cousins. In general, however, the frequency of rst-cousin marriages, and consanguinity in general, is declining in many traditional societies Consanguinity Consanguinity is not the most common explanation for an autosomal recessive trait. The mating of unrelated persons, each of whom happens by chance to be a carrier, accounts for most cases of autosomal recessive disease, particularly if a recessive trait has a high frequency in the population most affected persons with a relatively common disorder, such as CF, are not the result of consanguinity, because the mutant allele is so common in the general population. However, consanguinity is more frequently found in the background of patients with very rare conditions. For example, in xeroderma pigmentosum, a very rare autosomal recessive condition of DNA repair, more than 20% of cases occur among the offspring of marriages between rst cousins The Measurement of Consanguinity The measurement of consanguinity is relevant in medical genetics because the risk of a child’s being homozygous for a rare recessive allele is proportional to how related the parents are Consanguinity is measured by the coefcient of inbreeding (F), the probability that a homozygote has received both alleles at a locus from the same ancestral source; it is also the proportion of loci at which a person is homozygous for an allele from the same ancestral source, a situation referred to as identity by descent individual IV-1 is the offspring of a rst-cousin mating. Each of the four alleles at locus A (A1, A2, A3, and A4) in generation I has a 1/8 × 1/8 = 1/64 chance of being homozygous in IV-1; thus, the probability that IV-1 is homozygous for any one of the four alleles is 4 × 1/64 = 1/16 Types of consanguineous mating. The probability that the offspring in each of these matings is homozygous by descent at any one locus is equal to the coefcient of inbreeding Inbreeding Inbreeding is closely related to consanguinity. Inbreeding describes the situation in which individuals from a small population tend to choose their mates from within the same population for cultural, geographical, or religious reasons. In this situation, the parents may consider themselves unrelated but still may have common ancestry within the past few generations. Just as with consanguinity, The Hapsburg Jaw inbreeding increases the chance that individuals will be homozygous for an allele inherited from a common ancestor. Thus, in taking a family history, it is important to ask not only about consanguinity but also about the geographical origins of ancestors, especially if a couple seeking counseling is of similar ethnic or geographical origin Inbreeding As with consanguineous matings, it is possible to estimate a coefcient of inbreeding for individuals in a population even if they are not known to be related to each other. Although we make a distinction between consanguinity occurring within a family and inbreeding, which occurs between unrelated individuals from the same small ethnic group, an increased risk for mating between heterozygous carriers of autosomal recessive disorders exists in both situations. Rare Recessive Disorders in Genetic Isolates There are many small groups in which the frequency of certain rare recessive genes is greater than that in the general population. Such groups, genetic isolates, may have become separated from their neighbors by geographical, religious, or linguistic barriers. Although such populations are not consanguineous, the chance of mating with another carrier of a particular recessive condition may be as high as that observed in cousin marriages. Rare Recessive Disorders in Genetic Isolates Tay-Sachs disease (GM2 gangliosidosis) is an example of an autosomal recessive disease with increased frequency in certain genetic isolates (The disease is a neurological degenerative disorder that develops when a child is about 6 months old. Affected children become blind and regress mentally and physically). The disease is fatal in early childhood. Among Ashkenazi Jews in North America, for example, Tay-Sachs disease is 100 times more frequent (1 in 3600) than in other groups of European ancestry. This increased disease frequency is because the Tay-Sachs carrier frequency among Ashkenazi Jews, approximately 1 in 30, is 10-fold higher than in similar non-Ashkenazi European populations Rare Recessive Disorders in Genetic Isolates When the mutant alleles causing a recessive disease are relatively frequent in a particular population, unrelated spouses have a reasonable chance of both being heterozygous, and therefore consanguinity is generally not a striking feature in the families with affected children. For example, among Ashkenazi Jews, the parents of children with Tay-Sachs disease are usually not closely related. When the mutant allele is rare, however, the frequency of carriers is very low and consanguinity is often the explanation for how both members of a couple came to be heterozygotes. For example, consanguinity is often present in the parents of Tay-Sachs patients in the population of French ancestry in Quebec, Canada, where mutant alleles for Tay-Sachs disease are rare. Autosomal Dominant Inheritance More than half of all mendelian disorders are inherited as autosomal dominant traits. The incidence of some autosomal dominant disorders is high, at least in specic geographical areas for example, 1 in 500 for familial hypercholesterolemia in populations of European or Japanese descent Pedigree showing typical inheritance of a form of progressive sensorineural deafness (DFNA1) inherited as an autosomal dominant trait Achondroplasia - an incompletely dominant (or semidominant) trait Incompletely Dominant Inheritance Familial hypercholesterolemia, an autosomal dominant disorder leading to premature coronary heart disease. In this disorder, the rare homozygous patients have a more severe disease, with an earlier age at onset and much shorter life expectancy, than do the relatively more common heterozygotes New Mutation in Autosomal Dominant Inheritance and Relationship Between New Mutation and Fitness in Autosomal Dominant Disorders The most dominant conditions of any medical importance come about not only through transmission of the mutant allele from a carrier parent but also through inheritance of a spontaneous, new mutation in a gamete inherited from a nonheterozygous parent Once a new mutation has arisen, its survival in the population depends on the tness of persons carrying it At one extreme are disorders that have a tness of zero; in other words, patients with such disorders never reproduce, and the disorder is referred to as a genetic lethal All autosomal dominant genetic lethal diseases must be due to new mutations because these mutations cannot be inherited The affected individual will appear as an isolated case in the pedigree At the other extreme are disorders that have virtually normal reproductive tness because of a late age at onset or a mild phenotype that does not interfere with reproduction. If the tness is normal, the disorder is rarely the result of fresh mutation; a patient is much more likely to have inherited the disorder than to have a new mutant gene გმადლობთ , ყურადღებისთვის !!!