03. Membrane Transport - Overview.pdf
Document Details
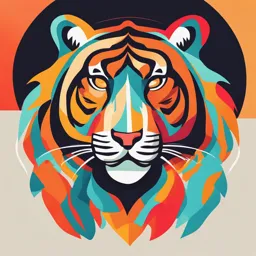
Uploaded by PerfectBowenite
Tags
Related
- Membrane Transport - BCCB2004 (Curtin University) PDF
- Module 4_Transport Mechanisms (1)-compressed.pdf
- Introduction to Medical Sciences 1 - Building Blocks of Cells Part 2 - Protein Functions PDF
- Chapter 4 - Membranes and Signalling PDF
- Chapter 3 Part 1: Cell Structure and Function PDF
- Ch. 3 Cells Part 1-2 PDF
Full Transcript
Module 3: Membrane Transport - Overview Slide 2: The learning objectives of this module are to explain the importance of the semi-permeable and selective nature of the plasma membrane; distinguish between tonicity and osmolality; differentiate between diffusion, osmosis, facilitated diffusion, and a...
Module 3: Membrane Transport - Overview Slide 2: The learning objectives of this module are to explain the importance of the semi-permeable and selective nature of the plasma membrane; distinguish between tonicity and osmolality; differentiate between diffusion, osmosis, facilitated diffusion, and active transport; compare the kinetics of facilitated diffusion and diffusion; differentiate between ion channels that are electrically activated, ligand-gated and mechanically sensitive; distinguish between uniporters, symporters, and antiporters; define the electrochemical gradient across the plasma membrane. Slide 3: The cell membrane is semi-permeable and selective. “Semi-permeable” means that not all molecules pass freely through the membrane. Only small uncharged molecules do this freely. The easiest pass is this for gasses such as carbon dioxide (CO2), oxygen (O2), etc. Next, some small, yet somewhat polar, molecules such as water (H2O) can also pass through. If the molecule is larger, uncharged, or nonpolar, it may also pass through the membrane. In contrast, a molecule that is large and polar such as glucose does not diffuse freely through the membrane. Finally, small molecules such as ions cannot cross the membrane unassisted due to their charge. As mentioned, water passes through the membrane; however, the movement is slow due to the polarity of the water molecules and the hydrophobic nature of the lipid bilayer. For faster transfer, water molecules cross the bilayer through channels called aquaporins. In addition to being semi- permeable, cell membranes are also selective. The membrane proteins may regulate the passage of molecules that otherwise cannot freely cross the membrane. What is the significance of this selective semi- permeability? By imposing barriers to entry, the cells preserve their composition and properties. These are requirements for functionality. Slide 4: There are four types of molecular movements across the cell membrane. Diffusion is the movement of a molecule (solute) from high to low concentration (“down the concentration gradient”). Osmosis describes the movement of water molecules (solvent) across a membrane. In this case, water molecules move from an area of low solute concentration to an area of higher solute concentration. In facilitated diffusion, the molecule moves down the concentration gradient (from high to low concentration), and the movement is aided by a channel or a carrier protein. Diffusion, osmosis, and facilitated diffusion do not require energy (they are passive). The fourth type of transport requires energy and is called “active”. In this case, the solute usually moves against its concentration gradient, from low to high concentration. In rare cases of active transport, the solute moves down its gradient; in these cases, the solute needs to be moved faster than via passive transport. Slide 5: The term “tonicity” describes the ability of the extracellular solution to make water molecules move in or out of a cell by osmosis. The tonicity depends on (1) the solute concentration and (2) the permeability of the cell membranes for the solute. As depicted in the figure, when the solutes in the extracellular solution are at a lower concentration than those in the cell, the extracellular solution is hypotonic, and the water molecules move into the cell. The extracellular solution is hypertonic, if its solute concentration is higher than this of the intracellular environment; then water exits the cell. In isotonic solutions, the number of water molecules moving in and out of the cell is the same. Related to tonicity are the terms designating the solute concentration. Osmolarity is the total concentration of all solutes, and it is measured by osmoles/liter. Osmolality is also the total concentration of all solutes; however, measured as osmoles/kg. One osmole is the amount of a substance that dissociates in solution to form one mole of osmotically active particles. These are particles that cannot move freely across a membrane. For example, 1 mol/liter NaCl in solution would result in 2 osmoles per liter – one osmole of sodium (Na+) and one of chloride (Cl-). Based on the description of osmosis, if two solutions are separated by a membrane permeable to water, but not to the solutes, water molecules will move from a solution of lower osmolarity to a solution of higher osmolarity. Slide 6 provides a link to a video on Molarity, Molality, Osmolarity, Osmolality, and Tonicity Slide 7: Osmotic pressure is the pressure caused by the imbalance of solutes on either side of the membrane; it is proportional to the number of solute particles (atoms or molecules) and not dependent on their size. Electrolyte refers to a solute that dissociates into ions in water (e.g., NaCl); whereas, non-electrolyte, does not dissociate into ions in water. For example, glucose dissolves in water, but it does not dissociate into ions in the solution. Osmoregulation is the maintenance of osmotic balance (the concentration of electrolytes or non-electrolytes across membranes). Slide 8: Let us differentiate between diffusion and facilitated diffusion. In both mechanisms, the solute moves down its gradient: from high to low concentration; however, how this is accomplished differs. Facilitated diffusion is carried out by carrier proteins (transporters) or channels, whereas no such are required for simple diffusion through the lipid bilayer. The binding of the solute to the carrier changes the shape (conformation) of the transporter, and these conformational changes allow for the release of the solute on the other side of the membrane. Compared to simple diffusion, facilitated diffusion is slower. The binding, the changing of conformation, and the release of the molecule take time. The kinetics of the two movements also differ. This is illustrated in figures A and B. Figure A depicts simple diffusion. Figure B depicts the kinetics of facilitated diffusion. In Figure B, at high solute concentrations, the transporting protein reaches a maximum rate and becomes saturated, and this is similar to the kinetics exhibited by enzymes. No saturation is imposed on the process of simple diffusion, as there are no proteins that facilitate the movement. One example of facilitated diffusion is the movement of glucose by a carrier protein such as GLUT1. GLUT1 transports glucose across the membrane of red blood cells, for example. The movement is down the glucose gradient (passive transport). Slide 9: How do passive and active transport differ? Before answering this question, let us find out what the transport of uncharged and charged molecules depends on. Small uncharged molecules move freely across membranes down their concentration gradient. However, charged molecules establish not only a concentration gradient but also an electrical gradient. The combination of concentration and electrical gradients determines the electrochemical gradient of charged molecules. In the passive transport of uncharged molecules, the movement is down the concentration gradient. In the passive transport of charged molecules, the movement is down their electrochemical gradient. In active transport, the solute’s movement is against its concentration or electrochemical gradient. This movement is also referred to as the “uphill” movement and it requires energy. This could be chemical energy, such as the one derived from ATP hydrolysis, or electrochemical gradient energy generated by the transport of another solute. The figure depicts the concept that the forces acting upon potassium ions across a membrane are a combination of chemical and electrical forces and are referred to as the electrochemical driving force. Slide 10: Active transport is accomplished with the help of transporters. All transporters are classified according to their (1) energy source and (2) direction of transport. Depending upon whether a carrier transports a single solute or two solutes, there are uniporters, symporters, and antiporters. The uniporters transfer a single type of molecule across the membrane. Symporters and antiporters transport two different solutes. Symporters transfer one solute dependent upon the transfer of a second solute, and the transfer is in the same direction. Antiporters transfer one solute dependent upon the transfer of a second solute, but the transfer of the second solute is in the opposite direction. Slide 11: Based on their energy source, there are three groups of active transporters (note that passive transporters also exist, as mentioned above in facilitated diffusion). There are coupled carriers that combine the uphill transport of one solute with the downhill transport of another solute. These carriers combine energetically unfavorable to energetically favorable movement. Another type of transporter is the ATP-driven pump that couples the uphill transport of a solute to the hydrolysis of adenosine triphosphate (ATP, an energy- rich compound). These transporting proteins have ATPase activity (ATPase: an enzyme that catalyzes the ATP hydrolysis, ATP → ADP + Pi). The third group of active transporters are the light-driven pumps; these are mainly present in bacterial cells (we will not discuss these). Slide 12: Let us look closer at the active transport based on ion gradients. The coupled transport mechanism allows the use of energy stored in the electrochemical gradient of one solute to transport another solute. Sodium (Na+), for example, is a frequently co-transported ion with its electrochemical gradient providing energy for the transport of a second molecule. Its movement is down its electrochemical gradient. Once inside the cells, sodium ions (Na+) are pumped out of the cell by an ATP-driven Na+ pump. The figure explains why the transport of Na+ is energetically favorable: (1) sodium entry into the cell is down its concentration (chemical) gradient, and (2) it is also favored due to the negative charge inside the cell. Slide 13: How is exactly the concentration of sodium ions maintained in cells? The maintenance is accomplished by the plasma membrane Na+-K+ pump. This is a P-type transport ATPase (called also Na+/K+ - ATPase). The importance of this pump is underscored by the fact that approximately one-third of the cell’s energy (in the form of ATP) is used by this pump. The pump maintains higher [K+] inside the cells, and higher [Na+] outside the cells. To do this, the pump hydrolyzes ATP, becomes auto-phosphorylated, and this is why it is called a P-type transport ATPase. With the help of this pump, there is (1) the transport of nutrients into the cells, and (2) the regulation of cytosolic pH and cell volume. In step 1, the transporter accommodates three sodium cations from the cytosol. In step 2, the ATP hydrolysis and autophosphorylation of the pump allow for its conformational change and the release of sodium ions into the extracellular space. In step 3, two potassium cations from outside the cells bind the pump. In step 4, the dephosphorylation of the pump changes its conformation and allows the release of potassium ions into the cytosol. Please, watch the five-minute video that demonstrates in detail the activity of the Na+/K+ ATPase. (Note that by exporting three positive ions and importing two positive ions, the pump is electrogenic) Slide 14: In addition to its effects on major cell functions, the Na+/K+ pump maintains the osmotic balance. Cells contain high concentrations of negatively charged organic molecules, and the membrane is impermeable to these. To balance the negatively charged organic molecules, the cell retains cations. This solute composition inside the cell is counteracted by an opposing osmotic gradient of inorganic ions such as Na+ and Cl- in the extracellular fluid. The Na+/K+ pump maintains the described osmotic balance by pumping Na+ out. The Cl- anions are kept out of the cells by the negative charges inside of the cells. Slide 15: More examples of P-type ATPases are the Ca2+ and H+ pumps (proton pumps). Calcium is usually moved out of the cytosol to keep the cytosolic calcium ion concentrations low. The low cytosolic Ca2+ levels allow the cells to respond to various signals that rely on a spike in calcium ion concentration. The low cytosolic [Ca2+] is maintained by a Ca2+ ATPase and a Na+-Ca2+ antiporter driven by the Na+ electrochemical gradient. The Ca2+-ATPases remove Ca2+ from the cytoplasm to either the outside of the cells or into internal organelles. One example is the Ca2+ ATPase in the sarcoplasmic reticulum membrane of skeletal muscle cells. The sarcoplasmic reticulum is a specialized type of smooth endoplasmic reticulum (ER) in the muscle cells; it stores Ca2+. In skeletal muscle cells, Ca2+ is released from the sarcoplasmic reticulum into the cytosol through Ca2+-release channels. The result is the stimulation of muscle contractions. During muscle relaxation, however, the removal of Ca2+ back into the reticulum is mediated by the Ca2+ ATPase pump. Slide 16: In addition to P-type ATPases, there are also F-type ATPases. The F-type ATPases are localized in the inner membrane of mitochondria. In this type of transport, the proton (H+) gradients across the mitochondrial membranes drive the synthesis of adenosine triphosphate (ATP) from adenosine diphosphate (ADP) and phosphate (in this case, ATP is synthesized, not hydrolyzed). The proton (H+) gradients are generated during the electron-transport steps of oxidative phosphorylation, which is a topic discussed in the Biochemistry course. Interestingly, similar ATPases can work in either direction (and therefore, either synthesize or hydrolyze ATP). The V-type ATPase pump drives the movement of hydrogen ions (H+) against their gradient (in this case, ATP is hydrolyzed to allow for the movement of protons against the gradient). Slide 17: Another type of ATPase is the ABC Transporters (ABC ATPases). As shown in the figure, two ATP- binding cassettes dimerize upon ATP binding and transport specific substrates. These transporters also catalyze the flipping of lipids from one side of the lipid bilayer to the other side. Other ABC transporters pump hydrophobic drugs out of the cells. Some of these transporters (called also MDR proteins) are responsible for the acquisition of multidrug resistance in cancer cells. The transporter associated with antigen processing (TAP) is also an ABC transporter. It is localized in the endoplasmic reticulum (ER) membrane and transports peptides from the degradation of foreign proteins to the cell surface, where the presented peptides are “scanned” by cytotoxic T lymphocytes. In cystic fibrosis, there is a mutation in an ABC transporter that regulates a chloride (Cl-) channel in the plasma membrane of epithelial cells; when both copies of the gene are mutant, the disease is manifested. Slide 18: Putting it together: carrier proteins transfer solutes across the lipid bilayer by undergoing conformational changes some carrier proteins transport a single solute “downhill,” others transport a solute against its electrochemical gradient, using energy from ATP hydrolysis, or the downhill flow of another solute (e.g., Na+ or H+) the family of P-type transport ATPases includes the Na+-K+ pump; each ATPase sequentially phosphorylates and dephosphorylates itself during the pumping cycle the ABC transporters are clinically important.