Histology of Human Cementum: Structure, Function, and Development (2016) PDF
Document Details
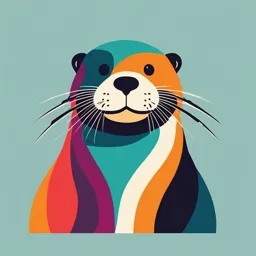
Uploaded by SplendidNephrite8490
South Bank University
2016
Tsuneyuki Yamamoto, Tomoka Hasegawa, Tomomaya Yamamoto, Hiromi Hongo, Norio Amizuka
Tags
Summary
This review article from Japanese Dental Science Review (2016) details the structure, function, and development of human cementum. It discusses various types, including cellular and acellular cementum, and examines the controversial conclusions drawn from previous research on human compared to rodent cementum .
Full Transcript
Japanese Dental Science Review (2016) 52, 63—74 Contents lists available at ScienceDirect Japanese Dental Science Review journal homepage: www.elsevier.com/locate/jdsr...
Japanese Dental Science Review (2016) 52, 63—74 Contents lists available at ScienceDirect Japanese Dental Science Review journal homepage: www.elsevier.com/locate/jdsr Review Article Histology of human cementum: Its structure, function, and development Tsuneyuki Yamamoto ∗, Tomoka Hasegawa, Tomomaya Yamamoto, Hiromi Hongo, Norio Amizuka Department of Developmental Biology of Hard Tissue, Hokkaido University Graduate School of Dental Medicine, Kita 13, Nishi 7, Kita-ku, Sapporo 060-8586, Japan Received 11 November 2015; received in revised form 1 February 2016; accepted 1 April 2016 KEYWORDS Summary Cementum was first demonstrated by microscopy, about 180 years ago. Since then the biology of cementum has been investigated by the most advanced techniques and equipment Acellular extrinsic at that time in various fields of dental sciences. A great deal of data on cementum histology have fiber cementum; been accumulated. These data have been obtained from not only human, but also non-human Cellular intrinsic fiber animals, in particular, rodents such as the mouse and rat. Although many dental histologists have cementum; reviewed histology of human cementum, some descriptions are questionable, probably due to Cellular mixed incorrect comparison of human and rodent cementum. This review was designed to introduce stratified cementum; current histology of human cementum, i.e. its structure, function, and development and to Extrinsic fibers; re-examine the most questionable and controversial conclusions made in previous reports. Intrinsic fibers; © 2016 Japanese Association for Dental Science. Published by Elsevier Ltd. This is an open access Human cementum article under the CC BY-NC-ND license (http://creativecommons.org/licenses/by-nc-nd/4.0/). Contents 1. Introduction............................................................................................................... 64 2. Classification of cementum................................................................................................ 64 3. Structure of cementum.................................................................................................... 64 3.1. Acellular extrinsic fiber cementum (AEFC).......................................................................... 64 3.2. Cellular intrinsic fiber cementum (CIFC)............................................................................ 65 3.3. Cellular mixed stratified cementum (CMSC).........................................................................65 3.4. Other varieties of cementum....................................................................................... 66 3.4.1. Acellular afibrillar cementum (AAC)........................................................................66 3.4.2. Intermediate cementum................................................................................... 66 ∗ Corresponding author. Tel.: +81 11 706 4224. E-mail address: [email protected] (T. Yamamoto). http://dx.doi.org/10.1016/j.jdsr.2016.04.002 1882-7616/© 2016 Japanese Association for Dental Science. Published by Elsevier Ltd. This is an open access article under the CC BY-NC-ND license (http://creativecommons.org/licenses/by-nc-nd/4.0/). 64 T. Yamamoto et al. 4. Composition of organic matrix............................................................................................. 68 4.1. Collagens........................................................................................................... 68 4.2. Non-collagenous proteins........................................................................................... 68 5. Function of cementum.....................................................................................................68 6. Development of cementum................................................................................................ 69 6.1. Acellular extrinsic fiber cementum (AEFC).......................................................................... 69 6.2. Cellular mixed stratified cementum (CMSC).........................................................................70 6.3. Origin of cementoblasts............................................................................................ 72 Conflict of interest........................................................................................................ 73 References................................................................................................................ 73 1. Introduction Cementum, or root cementum, is a mineralized tissue covering the entire root surface. According to Denton , cementum was first demonstrated microscopically by Fraenkel and Raschkow (1835) and Retzius (1836), and has since become a part of general knowledge in dentistry. Cementum exists fundamentally in mammalian teeth, which fit into alveolar sockets of alveolar bone, and functions as a tooth-supporting device in concert with the periodontal principal fibers and alveolar bone. The cementum covering the enamel surface also exists in several non-human ani- mals such as the horse, sheep, rabbit and guinea pig. This type of cementum is termed ‘‘coronal cementum’’ and dis- tinct from root cementum. Cementum is often referred to as a bone-like tissue. Cementum, however, is avascular, does not undergo dynamic remodeling, and increases in thick- ness throughout life. On these points, cementum is markedly different from bone. Figure 1 Full view of a mandibular molar (left) and a maxil- Knowledge of cementum histology has been accumu- lary incisor (right). Thin AEFC and thick CMSC cover cervical and lated with the advancement of investigating techniques and apical roots, respectively. CMSC is much thicker in the molar equipment. Most of the data, however, have been obtained than in the incisor. Hematoxylin-stained ground section. Bar from rodents such as the mouse and rat. Hence, previous 5 mm. reports on histology of human cementum have contained some questionable descriptions, probably due to incorrect extrinsic fiber cementum (AEFC)’’ contains densely packed comparison of human and rodent cementum. The purpose extrinsic fibers and no cementocytes (Fig. 2). AEFC corre- of this review is to introduce current histology of human sponds to classical acellular cementum. ‘‘Cellular intrinsic cementum and to re-examine the most questionable and fiber cementum (CIFC)’’ contains intrinsic fibers and cemen- controversial conclusions made in previous reports. tocytes. ‘‘Cellular mixed stratified cementum (CMSC)’’ corresponds to classical cellular cementum. Typical CMSC is partitioned by intensely hematoxylin-stainable lines or 2. Classification of cementum incremental lines. The individual partitioned cementum is CIFC, and occasionally AEFC (Fig. 3A—D). Namely, CMSC Cementum has historically been classified into cellular and represents the whole of cellular cementum composed of acellular cementum by inclusion or non-inclusion of cemen- stratified CIFC and AEFC. Cellular cementum with both tocytes. Generally, acellular cementum is thin and covers intrinsic and extrinsic fibers is often found within CMSC the cervical root, whereas thick cellular cementum cov- (Fig. 3D). This type of cementum is not distinctively clas- ers the apical root (Fig. 1) Cementum contains two types sified and is regarded as a sub-variety of CIFC in the current of fibers, i.e. extrinsic (Sharpey’s) fibers which are embed- classification. This review will deal with the three major ded ends of the principal fibers and intrinsic fibers which types. are fibers of cementum proper. It is believed that the extrinsic fibers are secreted by fibroblasts and partly cemen- toblasts and that the intrinsic fibers are secreted by only 3. Structure of cementum cementoblasts. Jones added these fibers to items of cementum classification, and Schroeder established the 3.1. Acellular extrinsic fiber cementum (AEFC) current classification, which is now widely used in the den- tal field. In accordance with this classification, three major Generally, AEFC covers cervical root surfaces in both perma- types of cementum are distinguishable [2—5]. ‘‘Acellular nent and deciduous teeth. The covering range is different Histology of human cementum 65 orientation changes at the incremental lines. The change is considered to correlate with relative positional changes of tooth and alveolar bone during tooth eruption. Unminer- alized cementum is seen as thin precementum or cementoid on AEFC. 3.2. Cellular intrinsic fiber cementum (CIFC) As mentioned above, CIFC is usually present as a component of CMSC [3—5] (Fig. 3A—C). The structure of CIFC will be described in detail in the following section. 3.3. Cellular mixed stratified cementum (CMSC) CMSC is predominantly seen in the interradicular and apical regions of roots (Figs. 1 and 3A—C). According to Schroeder , in middle-aged individuals, its maximum thickness ranges between 400 and 600 m in incisors, around 500 m in canines, between 300 and 1000 m in premolars, and between 700 and 1500 m in molars. In molars, CMSC often covers apical two thirds of the roots (Fig. 1). CMSC usually consists of stratified CIFC, with each CIFC being demar- cated by intensely hematoxylin-stainable incremental lines Figure 2 Light (A, B) and transmission electron (C) micro- (Fig. 3A—D). Occasionally, AEFC also appears as a compo- graphs showing AEFC. (A) Principal fibers (PF) enter AEFC (*) nent of CMSC (Fig. 3B, C). CIFC often contains extrinsic as extrinsic fibers. D, dentin. Hematoxylin and eosin-stained fibers as well as intrinsic fibers, and the density of extrinsic paraffin section. Bar 50 m. (B) Extrinsic fibers are observed as fibers varies among individual CIFC. Hence, various types white lines in AEFC. Extrinsic fibers change their orientation at of CIFC, i.e. extrinsic fiber-rich CIFC, extrinsic fiber-poor the intensely stainable incremental lines (arrows). D, dentin. CIFC, and extrinsic fiber-free CIFC are subdivided on the Hematoxylin-stained ground section. Bar 50 m. (C) Extrinsic basis of the density of extrinsic fibers (Fig. 3D). In extrin- fibers are branching and anastomosing. D, dentin; CB, cemen- sic fiber-containing CIFC, the extrinsic fibers, like those in toblasts; PC, precementum; PF, principal fibers. Bar 5 m. AEFC, show branching and anastomosing, and are encircled by the intrinsic fibers [3,7,14] (Fig. 3E, F). The diameter of extrinsic fibers is under 10 m and thicker than those extrin- among types of teeth; 60—90% of the total root length in sic fibers in AEFC. The extrinsic fibers of CIFC often contain single-rooted teeth, and cervical half to one third in multi- an unmineralized central core, surrounded by a highly min- rooted teeth (Fig. 1). Its thickness, which increases with age, eralized cortical part [3,7]. It is presently unknown whether ranges from 50 to 200 m [3,4]. all extrinsic fibers in CIFC have such a core. AEFC contains collagen fibers and non-collagenous In hematoxylin-stained sections an alternation of darkly proteins as organic matrices, both of which are fully mineral- and faintly stainable lines, both of which are about ized. All collagen fibers belong to extrinsic fibers connecting 2.5 m thick, is seen within extrinsic fiber-poor and -free to the principal fibers in the periodontal ligament [2—4]. The CIFC [14—18] (Fig. 4A). For convenience in description, extrinsic fibers are densely packed and arranged nearly per- these lines will be referred to as ‘‘lamellae’’ in this pendicularly to the root surface (Fig. 2A, B). The diameter review. Scanning electron microscopy revealed that two of extrinsic fibers is roughly calculated at 3—6 m. They types of lamellae, i.e. lamellae with longitudinally and are not simple straight fiber bundles, and show branching near-longitudinally cut fibrils and those lamellae with and anastomosing (Fig. 2C). The structural correlation of transversely and near-transversely cut fibrils stratified the extrinsic fibers and dentin matrix fibers at the cemento- alternately and created the alternating lamellae [16—18] dentinal junction has been often disputed. A majority of (Fig. 4B). The alternating lamellae are very similar to those previous studies revealed interdigitation of extrinsic fibers in compact bone [19—21]. In compact bone a twisted ply- and dentin matrix fibers and thus suggested that cementum wood model has been proposed to explain the alternating and dentin were firmly united by the fiber interdigitation lamellae. According to this model, all collagen fibrils and mineralization [4,6—9]. In contrast, some investigators run parallel in a given plane, and their direction rotates from found a fibril-poor, non-collagenous protein-rich layer at the plane to plane. A 180◦ rotation of fibril arrays corresponds cemento-dentinal junction and proposed that the proteins to a period. This periodic change in fibril array orientation served as an adhesive of cementum and dentin [10—12]. appears as the alternating lamellae when observed in histo- Thick AEFC shows several incremental lines which are logical sections. The alternating lamellae in CIFC conform to intensely stained with hematoxylin (Fig. 2B). The incremen- the twisted plywood model [16—18] (Fig. 4B). In a single CIFC tal lines are highly mineralized and are thus regarded the alternating lamellae are more distinct on the periodon- as resting lines, formed in resting phases during intermit- tal ligament side than on the dentin side (Fig. 4A). In other tent AEFC formation. In many cases, the extrinsic fiber words, the lamellae are more obvious on the dentin side of 66 T. Yamamoto et al. Figure 3 (A—D) Micrographs showing structural variety of CMSC in hematoxylin-stained ground sections. CMSC is partitioned by many, intensely stainable incremental lines. RD, root dentin. Bars 100 m (A—C), 50 m (D). (A) CMSC consists of stratified CIFC. (B) AEFC (*) is present as the first formed cementum of CMSC. (C) AEFC (*) intervenes between CIFC. (D) Magnification of extrinsic fiber-rich (*), -poor (**), and -free CIFC (***). (E, F) Transmission electron micrographs showing extrinsic (EF) and intrinsic fibers (IF) in CIFC. Bars 5 m. (E) Extrinsic fibers are branching and anastomosing. Intrinsic fibers fill the space between extrinsic fibers. (F) Intrinsic fibers encircle extrinsic fibers or meander among them in a tangential section parallel to cementum surface. an incremental line, and less obvious on the periodontal lig- which are about to become Hertwig’s epithelial root sheath. ament side. How the periodic rotation of intrinsic fibers is (3) AAC is a mere precipitate derived from tissue fluid or created and how the structural disproportion appears will serum. be discussed later. 3.4.2. Intermediate cementum The intermediate cementum has confused dental histolo- 3.4. Other varieties of cementum gists since it first appeared in the literature and its origin is still controversial in the dental histological field. Hence, 3.4.1. Acellular afibrillar cementum (AAC) the intermediate cementum will be re-examined here. AAC consists of a mineralized matrix containing neither col- In 1927, Bencze first used the term ‘‘intermediate lagen fibers nor cementocytes. AAC is found as isolated cementum (intermediäre Zementscicht)’’ to indicate a patches or as the most cervical part of AEFC on enamel just narrow part containing cellular elements and/or lacunae coronal to the cemento-enamel junction [3,7,8]. Its compo- between dentin and CMSC (Fig. 5A, B). Earlier, Hopewell- sition of non-collagenous proteins is very similar to that of Smith had found a homogeneous layer between AEFC AEFC. However, its function and origin have not yet been and the granular layer of Tomes (Fig. 5C). The homogeneous determined. Furthermore, it is still unknown whether AAC layer is now referred to as the hyaline layer of Hopewell- is an essential tissue for the tooth. Three possible origins Smith. On the basis of elaborate histological observations, it have been proposed : (1) Connective tissue-derived cells is now established that the two tissues are homologous. The produce AAC. If this is true, connective tissue cells must structure in question is referred to as the hyaline layer of replace reduced enamel epithelium. (2) AAC is an epithe- Hopewell-Smith in the AEFC region and as the intermediate lial product, deposited by the inner enamel epithelial cells cementum in the CMSC region. With regard to its origin, Histology of human cementum 67 [25,26] observed the intermediate cementum in human pre- molars and molars and found the continuity of dentinal tubules between the intermediate cementum and dentin. Hence, he concluded that the intermediate cementum was a surface layer of dentin (= mantle dentin or a part of man- tle dentin). Schroeder and Yamamoto [10,27] agreed with this conclusion. In addition, Owens [25,26] and sub- sequently, Kawasaki elucidated that the hyaline layer of Hopewell-Smith started to be mineralized later than the deep part of dentin (= circumpulpal dentin). Afterwards, this finding became a significant key for another hypothesis of the second group. The second group believes that the intermediate cemen- tum is not a part of dentin, but an enameloid-like tissue produced by the epithelial root sheath. This hypothesis will be referred to as an epithelial origin hypothesis in this section. In brief, the epithelial origin hypothesis is based on the following logic [29—31]. (1) The cemento- dentinal junction or the innermost cementum layer in rodent teeth corresponds to the intermediate cementum in human teeth. (2) The cemento-dentinal junction is an epithelial sheath product in rodent teeth. (3) Hence, the interme- diate cementum in human teeth is of epithelial sheath origin. Regarding the premise (1), approximately 1 m thick superficial dentin layer shows delayed mineralization in Figure 4 (A) Magnification of extrinsic fiber-free CIFC par- initial acellular cementogenesis of rat molars [27,32,33]. titioned by incremental lines (arrows). In CIFC an alternation Thereafter, an intensely hematoxylin-stainable, PAS-positive of darkly and faintly stainable lamellae is obvious on the peri- matrix appears in the superficial dentin layer and forms the odontal ligament side (*) and non-obvious on the dentin side cemento-dentinal junction [27,32—35]. In addition, many (**). Bar 30 m. Hematoxylin-stained ground section. (B) Scan- epithelial sheath cells are embedded near the cemento- ning electron micrograph showing the alternating lamellae. The dentinal junction in rat cellular cementogenesis [36,37]. The specimen (a mandibular molar) has been treated by 10% NaOH cemento-dentinal junction of human teeth, like that of rat maceration method to observe individual collagen fibrils clearly. teeth, is intensely hematoxylin-stainable and PAS-positive Two types of lamellae, i.e. lamellae of longitudinally and near- (10). Nevertheless, based on the delayed mineralization and longitudinally cut fibril arrays (*) and lamellae of transversely cell inclusion, the second group considers the cemento- and near-transversely cut fibril arrays (**) create the alternat- dentinal junction in rat teeth to correspond to the interme- ing lamellae. Four types of fibril arrays are roughly recognized: diate cementum in human teeth. The superficial dentin layer 1, longitudinally cut fibril arrays; 2, obliquely cut fibril arrays in rat molars is a very narrow, fibril-poor tissue and obviously facing downward; 3, transversely cut fibril arrays; 4, obliquely different from the mantle dentin which consists of densely cut fibril arrays facing upward. As traced from left to right, the packed dentin matrix fibers [27,32—35]. Further, it is estab- fibril arrays appear to rotate clockwise. Bar 3 m. lished that the epithelial sheath cells are never embedded in the cementum in human cementogenesis [3,4,6—9]. Hence, the premise (1) of the epithelial origin hypothesis is lacking the two investigators proposed different views. Hopewell- supportive evidence. The premise (2) is still controversial, Smith described the homogenous layer as a peripheral part whether the hematoxylin-stainable matrix is a cementoblast of dentin. In contrast, Bencze insisted that the interme- product or epithelial sheath cell product is not yet deter- diate cementum was a part of cementum, although he had mined. Even though the matrix is an epithelial product, this earlier assumed it to be a part of dentin. The origin of the does not relate to the origin of the intermediate cemen- intermediate cementum has been a focus of many dental tum. For these reasons, the epithelial origin hypothesis is histologists. In histological sections where the cemento- questionable, based on uncertain premises. dentinal junction is clearly differentiated, the intermediate Fujita regarded the intermediate cementum as a cementum exists on the dentin side of the junction and peripheral part of dentin and added, ‘‘Most of the lacunae there is no boundary between the intermediate cementum in the intermediate cementum are considered to be swollen and dentin (Fig. 5A—C). This fact proves that the interme- dentinal tubules, and thus the cellular elements would be diate cementum is definitely a part of dentin. Nevertheless swollen processes of odontoblasts. If the lacunae contain some investigators have still emphasized that the inter- cell bodies, they would be those of odontoblasts which have mediate cementum is not dentin, and further, that it is failed to retreat.’’ Actually, Yamamoto found that the a particular tissue produced by Hertwig’s epithelial root lacunae in question were definitely continuous with den- sheath. tinal tubules. Hence, to date Fujita’s view appears most The investigators who studied the origin of the inter- reasonable. In conclusion in this section, the intermediate mediate cementum can be divided into two groups. The cementum is a part of dentin, not cementum, and this term first group believes that it is a part of dentin. Owens may be reconsidered to avoid unnecessary confusion. 68 T. Yamamoto et al. Figure 5 (A—C) Light micrographs showing the apical roots covered with CMSC (A, B) and cervical root covered with AEFC (C) in hematoxylin-stained ground sections. Bars 100 m. (A, B) The intermediate cementum (*) contains lacunae (small arrows) and exists on the dentin side of the cemento-dentinal junction (large arrow). Arrowheads in (B) indicate the granular layer of Tomes. (C) The hyaline layer of Hopewell-Smith (*) is present between the granular layer of Tomes (arrowheads) and cemento-dentinal junction (arrow). 4. Composition of organic matrix proteoglycans inhibit mineralization of collagen fibrils by possessing specific sites on collagen fibrils normally destined There have been no investigations which have detected the to be filled with hydroxyapatite. Thus the proteoglycan con- chemical composition separately in individual cementum tent becomes lower after mineralization. types. The following description is not for a particular type The presence of enamel-related proteins as constituents of cementum. of cementum is still controversial. Although some investiga- tors [43,44] proposed that these proteins in cementum have cementoblast-inducing activity, this view is still presently 4.1. Collagens unconfirmed. Great efforts have been made, to identify unique The organic matrix of cementum consists predominantly of cementum-specific marker proteins. Cementum-derived collagens. In bovine cementum type I collagen accounts for growth factor, cementum attachment protein, and cemen- more than 90% of the organic matrix and type III collagen tum protein-23 had been the candidates, but were all later approximately 5%. In human cementum type I colla- found in tissues other than cementum. To date, marker gen appears to be the only collagen type. The collagens proteins specific to only cementum are not yet discovered. form cross-striated fibrils in cementum and induce biological mineralization as a scaffold for the mineral crystals dur- 5. Function of cementum ing mineralization, and maintain the structural integrity of cementum after mineralization. The main function of cementum is tooth support or tooth anchorage together with the principal fibers and alveolar 4.2. Non-collagenous proteins bone. AEFC is therefore the most suitable cementum for tooth support [3—5,7]. The function of CIFC is more compli- Major non-collagenous proteins are bone sialoprotein and cated. Extrinsic fiber-poor and -free CIFC do not appear to osteopontin [7,8]. They play important roles in the mineral- contribute to tooth support. Instead their function is adapta- ization process, binding collagen fibrils and hydroxyapatite. tion, i.e. reshaping the root surface during tooth movement After mineralization, they serve to maintain structural and compensating for crown wear [2—5]. Such CIFC also integrity of cementum. By immunohistochemistry AEFC con- appears as reparative cementum which fills resorbed root tains the two glycoproteins more densely than CIFC. Their surfaces. As described previously, extrinsic fiber-poor and high density in AEFC is probably associated with its slower -free CIFC have the alternating lamellae, based on the formation speed. twisted plywood structure. In compact bone, the structure is Proteoglycans are complex macromolecules composed of considered to resist stresses from various directions. In a core protein to which glycosaminoglycans are covalently the same way, the alternating lamellae in CIFC may function attached. By immunohistochemistry dermatan sulfate, to resist multi-directional masticatory stresses. In contrast, chondroitin sulfate, and keratan sulfate are detected as extrinsic fiber-rich CIFC may serve as tooth support more glycosaminoglycans. Regarding the types of proteoglycans, than adaptation. When the adaptation is required, extrin- versican as large proteoglycan and decorin, biglycan, and sic fiber-poor or -free CIFC forms patch-wise on applicable lumican as small proteoglycans are detected. They exist portions. In contrast, when tooth anchorage is required, exclusively in cellular cementum [41,42]. It is proposed that extrinsic fiber-rich CIFC or AEFC forms. Histology of human cementum 69 CMSC is generally thicker in molars than in anterior teeth. The reason may be deduced simply; the thickness of CMSC is parallel to the masticatory stress loaded on the tooth. However, it is often found that impacted or pre-functional molars, like fully functioning molars, possess thick CMSC. In relation to the CMSC thickness, Schroeder commented, ‘‘CMSC distribution and thickness of a particular tooth may reflect its past history of eruption rather than masticatory function. Pre- and post-eruptive tooth movements may thus, necessitate CMSC deposition.’’ This view has not yet been established, but appears reasonable. 6. Development of cementum Due to ethical issues, there have been no reports which investigate human cementogenesis precisely. The follow- ing section is fundamentally based on previous reports [3,4,6—9,47], in which developing human premolars were observed from apical to cervical regions to follow cemento- genesis. In some portions, findings from rat cementogenesis will be used supplementally and to a minimum. 6.1. Acellular extrinsic fiber cementum (AEFC) Premolars with roots developed to 50—60% of their presum- able final length were examined [4,6,7]. At the forming root tip Hertwig’s epithelial root sheath proliferates and Figure 6 Schematic diagram depicting AEFC genesis. Section grows apically, inducing dental papilla cells to differentiate 1: Cementoblasts appear and start to form fiber fringe on the into odontoblasts. Where the initial dentin mineralization unmineralized dentin. Periodontal ligament fibers are arranged starts, the external and internal surface of dentin is not in parallel with the root surface. Section 2: Fiber fringe with yet mineralized, and thus unmineralized dentin, i.e. pre- maximum density is established. Dentin mineralization reaches dentin, depicts a V-shape (Fig. 6, section 1). In premolars the base of fiber fringe and progresses into the fringe. Section the distance between mineralized dentin tip and predentin 3: Fiber fringe elongates and begins to connect with periodon- tip (= forming root tip) is about 50 m. Cervically, the epithe- tal ligament fibers. Section 4: The tooth anchorage system, or lial sheath separates from the root surface and disintegrates principal fiber-extrinsic fiber linkage, is established. CB, cemen- into cell clusters, i.e. epithelial cell rests of Malassez. toblasts; ERM, epithelial cell rests of Malassez; FF, fiber fringe; Cementoblasts with fibroblast-like shape and collagen fib- HERS, Hertwig’s epithelial root sheath; MD, mineralized dentin; rils subsequently appear on the exposed dentin surface. Ends PLF, periodontal ligament fibers; UMD, unmineralized dentin. of the fibrils intermingles with predentin matrix fibers. The Modified from Schroeder. fibrils aggregate into short bundles, which are arranged in parallel and oriented almost perpendicularly to the root sur- face (Fig. 6, section 1). At this point the fibril bundles are not same time bone sialoprotein and osteopontin, secreted from yet connected with periodontal ligament fibers which are cementoblasts, become detectable in the cementum. Min- arranged parallel to the root surface. Hence, in the strict eralized spherules also emerge as isolated patches in the sense the fibril bundles are not extrinsic fibers. In accor- vicinity of the fiber fringe. This means that early mineral- dance with previous reports [4,6,7], they will be referred to ization of the fiber fringe starts from two sites. After the as ‘‘fiber fringe’’ in this section. From findings of human two mineralization fronts coalesce, mineralization proceeds and rat AEFC formation [4,48,49], the fibril-aggregating outward with additional AEFC formation. or fringe-generating mechanism is presumed as follows. More cervically, AEFC increases in thickness and the fiber Cementoblasts as well as fibroblasts extend wing- or plate- fringe becomes more elongated. The periodontal ligament like processes and surround the immature fibril bundles. fibers develop further and change their arrangement to form They then form tubular compartments by connecting the principal fibers (Fig. 6, section 3). In premolars, when the processes in concert with other cementoblasts (Fig. 7), and cervical AEFC reaches about 15—20 m thickness or the add collagen fibrils linearly and laterally to the immature fib- tooth is about to start occlusion, the fiber fringe becomes ril bundles within the compartments. As a result, the fiber continuous with the principal fibers [4,7,9]. At this point fringe is generated on the root surface. the fiber fringe is organized into extrinsic fibers and the The mineralization of the external dentin proceeds out- tooth anchorage system is established in the cervical region ward and eventually reaches the base of fiber fringe. This (Fig. 6, section 4). Thereafter, cementoblasts concentrate point is about 300 m distant from the root tip in premo- on secretion of non-collagenous proteins to induce further lars. Then the fiber fringe begins to be mineralized and can AEFC mineralization and strengthen the anchorage system. be recognized as the AEFC matrix (Fig. 6, section 2). At the The mechanism by which the fiber fringe connects to the 70 T. Yamamoto et al. Figure 7 (A, B) Cementoblasts on established AEFC in rat molars by transmission (A) and scanning electron microscopy (B). Bars 30 m. (A) Cementoblasts encircle principal fibers with cytoplasmic processes in a tangential section through cementum surface. (B) The specimen has been treated by KOH-collagenase method and thereby collagen fibers and interfibrillar matrix are removed and only cementoblasts can be selectively observed. Cementoblasts are viewed from the cementum side. Cementoblasts form cylindrical compartments with wing-like processes. principal fibers is still unknown. As described previously, cementum increases in thickness with age. According to Sequeira et al. , the growth rate of cervical AEFC is approximately 2.9 m/year in maxillary first premolars and approximately 1.5 m/year in mandibular second premo- lars. 6.2. Cellular mixed stratified cementum (CMSC) CIFC usually forms as the first cementum in CMSC genesis [3—7] (Fig. 3A—C). It has been widely believed that occlusal stimuli trigger CISC genesis. However, Bosshardt and Selvig claimed, ‘‘It seems that the initiation of CIFC gene- sis does not depend on stimuli transmitted by masticatory forces and that influence by pressure may reduce the rate of matrix deposition.’’ The trigger for CIFC deposition is still unclear. The development of extrinsic fiber-free CIFC will be here described for the initial genesis of CMSC. Following find- ings were obtained from premolars with roots developed to 75% of their presumable final length [4,6,7]. At the forming Figure 8 Schematic diagram depicting the formation of root tip the CIFC deposition starts almost concurrently with extrinsic fiber-free CIFC as the initial CMSC genesis. Section dentin formation. Cementoblasts appear on the predentin 1: Cementoblasts appear and produce the cementum matrix immediately after Hertwig’s epithelial sheath detaches from rapidly in a multipolar mode on the unmineralized dentin. Sec- it (Fig. 8, section 1). The cementoblasts assemble densely tion 2: Dentin mineralization reaches the cementum matrix and and begin to secrete collagen fibrils in various directions. progresses into it. Cementoblasts produce cementum matrix These fibrils are therefore randomly arranged in the ini- slowly in a unipolar mode. Unmineralized cementum matrix is tial CIFC and intermingle with predentin matrix fibers. As recognized as precementum. CB/m, cementoblasts with mul- collagen fibrils are densely accumulated, cementoblasts tipolar matrix production; CB/u, cementoblasts with unipolar show concaves on the whole cell surface, which compart- matrix production; CM, cementum matrix; ERM, epithelial cell mentalize the newly formed fibril bundles. In accordance rests of Malassez; HERS, Hertwig’s epithelial root sheath; MCM, with Bosshardt and Schroeder , these cementoblasts mineralized cementum matrix; MD, mineralized dentin; UMD, are producing CIFC matrix in a multipolar mode causing unmineralized dentin; PC, precementum. rapid matrix deposition. Some cementoblasts are embedded Modified from Schroeder. Histology of human cementum 71 Figure 9 (A) Transmission electron micrographs showing the CIFC surface where alternating lamellae are generating. Flat cemen- toblasts cover a lamella (*) of transversely and near-transversely cut fibrils. Bar 2 m. (B) Magnification of the boxed area in (A). A long, thin process (large arrow) is in a close and parallel association with longitudinally cut fibrils (small arrows). Bar 2 m. as cementocytes in the rapidly growing matrix. At this the CIFC surface where the alternating lamellae were gen- point the CIFC matrix is still unmineralized, meaning it is erating [16—18] (Fig. 9). These findings lead to the following still precementum (Fig. 8, section 1). The external dentin conclusion: the cementoblasts control the intrinsic fiber is mineralized much faster than in AEFC genesis. After arrangement with the finger-like processes. They move the the dentin mineralization extends to CIFC (approximately processes synchronously and periodically and cause a peri- 100—200 m from the root tip), cementoblasts shift their odic change in intrinsic fiber arrangement. This dynamic matrix-producing mode from multipolar to unipolar, and pro- sequence results in the alternating lamellae. Osteoblasts duce CIFC matrix slowly only in parallel with root surface. At were also recently suggested to create the alternating the same time, bone sialoprotein and osteopontin become lamellae in a similar fashion in rat compact bone. detectable in the mineralized CIFC. A narrow precementum The structural disproportion of the lamellae has been inter- containing numerous mineralized spherules appears on the preted as follows: whenever cementoblasts start to deposit CIFC surface (Fig. 8, section 2). cementum matrix again after a resting phase, the cells can- After the initial CIFC is established on the root surface not yet organize intrinsic fibers sufficiently. The intrinsic remote from the root tip, CIFC and AEFC form in unpre- fibers are accordingly poorly organized. As the cemento- dictable order with resting phases. As described previously, blasts recovers fiber-organizing activity, the intrinsic fibers the surrounding environment or requirement (adaptation become well-organized to create the alternating lamellae and tooth anchorage) determines the type of cementum. In [15—18]. Cementum increases in degree of mineralization other words, according to the ratio of the two requirements, toward the periodontal ligament side between adjoining various types of cementum, i.e. AEFC, extrinsic fiber-rich incremental lines , which suggests that cementoblasts CIFC, extrinsic fiber-poor CIFC, and extrinsic fiber-free CIFC, recover mineralization-inducing activity as well. are generated. At the same time, the matrix-producing The second type had wing-like and finger-like processes. modes may also be determined to control the cemento- The cementoblasts connected the wing-like processes to genesis speed. When the cementum deposition shifts form tubular compartments surrounding the principal fibers. from extrinsic fiber-rich CIFC to extrinsic fiber-poor and - The finger-like processes, arranged in parallel with the free CIFC, all or part of the extrinsic fibers lose continuity cementum surface, were formed on the cementum-facing with the principal fibers. side of the wing-like processes. Intrinsic fibers emerged in In a series of studies of human and rat cementogene- close and parallel association with the finger-like processes sis, two morphologically different types of cementoblasts (Fig. 10). From these findings the following was suggested were found in the advanced CIFC formation [16—18]. The (Fig. 11): when the cementoblasts move away from the first type was found on the extrinsic fiber-poor or -free cementum or are embedded in the cementum, these cells CIFC, and second type on the extrinsic fiber-rich CIFC. The retract the wing-like processes, dividing them into finger- first type had long finger-like processes and was suggested like processes. At the same time they secrete fibrils along to secrete intrinsic fibers in close and parallel associa- the finger-like processes to fill the spaces around the prin- tion with the processes. The cementoblasts of this type cipal fibers. As a result, the intrinsic fibers encircle the extended the processes together in the same direction on extrinsic fibers in extrinsic fiber-rich CIFC [18,53,54]. 72 T. Yamamoto et al. referred to as a classical mesenchymal hypothesis in this section. In the 1980s and 1990s a different hypothesis has arisen from in vitro and in vivo experiments using rats and mice, namely that some epithelial sheath cells transd- ifferentiate into cementoblasts by epithelial-mesenchymal transition (EMT). This hypothesis will be referred to as an alternative epithelial hypothesis. The alternative epithelial hypothesis has now almost surpassed the classical mesenchymal hypothesis, because the alternative epithelial hypothesis can explain why epithelial sheath cells decrease in number during epithelial sheath disintegration [56—59]. EMT is a normal phenomenon in embryos in several devel- oping organs, e.g. in palatogenesis [60,61]. However, EMT of epithelial sheath during cementogenesis is not yet deter- mined. The alternative epithelial hypothesis has been first pro- posed in an in vitro study by Thomas. He found that cultured epithelial sheath cells changed shape into mes- Figure 10 Transmission electron micrograph showing the enchymal cell phenotype and co-expressed vimentin and extrinsic fiber-rich CIFC surface in a tangential section through keratin, which are markers of mesenchymal and epithelial the cementum surface. Three sections are divided. Section 1 cells, respectively. Based on the fact that epithelial cells indicates the interior of cementum, where intrinsic fibers (IF) undergoing EMT co-express vimentin and keratin [60,61], surround extrinsic fibers (EF). Section 2 indicates the cementum Thomas proposed that the cultured epithelial sheath surface. Intrinsic fibers and cementoblasts (CB) surround prin- cells transformed into mesenchymal cementoblasts, and cipal fibers. Section 3 indicates an area slightly distant from further that epithelial sheath cells similarly transformed the cementum surface. Cementoblasts (CB) surrounds principal into cementoblasts through EMT during in vivo normal fibers with cytoplasmic processes. Only a few or no intrinsic cementogenesis. Subsequently, findings supportive of the fibers are seen around principal fibers. Bar 5 m. alternative epithelial hypothesis have been accumulated in in vitro [63—65] and in vivo studies [66—68]. It is likely 6.3. Origin of cementoblasts that cultured epithelial sheath cells undergo EMT under special conditions facilitating EMT. However, results of the Cementoblasts have long been believed to derive from mes- in vivo studies are questionable, because in these studies enchymal dental follicle [3,45,55,56]. This concept will be cementoblasts and epithelial cells were probably misiden- tified. In the in vivo studies, which used rat and mouse teeth [66—68], some cementoblasts and cementocytes were keratin-immunoreactive, and thus these cells were regarded as the epithelial sheath cells which were undergoing EMT at that exact moment. However, in rat and mouse cemento- genesis [36,37,56,57], it is established that epithelial cell rests of Malassez coexist with true cementoblasts on the cementum surface and that many epithelial sheath cells are embedded in the cellular cementum. Therefore, in the in vivo studies epithelial cell rests and embedded epithe- lial cells were probably misidentified as cementoblasts and cementocytes, respectively. Findings supportive of the clas- sical mesenchymal hypothesis have also accumulated in in vivo studies using mouse and rat teeth [56,58,59,69—71]. In these studies any epithelial sheath-derived cells did not express mesenchymal or cementoblast characteristics, e.g. immunoreactivity for vimentin, bone sialoprotein, and osteopontin. Taken together, to date there is no evidence to support the alternative epithelial hypothesis in in vivo Figure 11 Schematic diagram depicting how cementoblasts cementogenesis. produce intrinsic fibers around principal fibers on extrinsic The epithelial cells decrease in number during epithelial fiber-rich CIFC. Cementoblasts surround principal fibers (PF) in sheath disintegration when observed in histological sections cylindrical compartments with wing-like processes. With fur- [56—59]. If EMT does not occur, how can the cell number ther cementogenesis, they retract the wing-like processes and reduction be explained? Apoptosis may be another possible divide them into finger-like processes. At the same time they cause for the cell number reduction, but it has been shown secrete intrinsic fibers (IF) along the finger-like processes. As that apoptosis occurs in epithelial cell rests of Malassez and a result, the intrinsic fibers encircle the extrinsic fibers (EF) in epithelial cells embedded in cementum, but does not in the the cementum. disintegrating epithelial sheath [59,72,73]. Recent studies Modified from Yamamoto et al.. [56,58,59] have suggested that the cell number reduction Histology of human cementum 73 Bosshardt DD, Schroeder HE. Cementogenesis reviewed. A com- parison between human premolars and rodent molars. Anat Rec 1996;245:267—92. Bosshardt DD, Selvig KA. Dental cementum: the dynamic tissue covering of the root. Periodontology 2000 1997;13:41—75. Bosshardt DD, Zalzal S, Mckee MC, Nanci A. Developmental appearance and distribution of bone sialoprotein and osteo- pontin in human and rat cementum. Anat Rec 1998;250:13—33. Nanci A, Bosshardt DD. Structure of periodontal tissues in health and disease. Periodontology 2000 2006;40:11—28. Yamamoto T, Domon T, Takahashi S, Islam N, Suzuki R, Wakita M. The structure and function of the cemento-dentinal junction Figure 12 Schematic diagrams depicting the disintegration in human teeth. J Periodontal Res 1999;34:261—8. of Hertwig’s epithelial root sheath during root development. Yamamoto T, Domon T, Takahashi S, Islam MN, Suzuki R. The (A) Hertwig’s epithelial root sheath (HERS) bends inside toward fibrous structure of the cemento-dentinal junction in human dental pulp (DP) at the tip of developing root (R). (B) Three- molars shown by scanning electron microscopy combined with dimensionally, the epithelial sheath forms a tapered cylinder. NaOH-maceration. J Periodontal Res 2000;35:59—64. (C) Root formation proceeds almost straight, whereas the Yamamoto T, Domon T, Takahashi S, Islam MN, Suzuki R. The epithelial sheath maintains the tapered shape. As a result, fibrillar structure of the cemento-dentinal junction in different due to the discrepancy in surface area, the epithelial sheath kinds of human teeth. J Periodontal Res 2001;36:317—21. Fujii Y. Mineralization pattern of human cementum. Shigaku is stretched out and fragmented into epithelial cell rests of 1969;57:206—28 [in Japanese with English abstract]. Malassez. Yamamoto T, Domon T, Takahashi S, Islam MD, Suzuki R, Modified from Yamamoto et al.. Wakita M. The regulation of fiber arrangement in advanced cellular cementogenesis of human teeth. J Periodontal Res 1998;33:83—90. may be related to the growth rate and shape of the epithe- Chen M. Observations on the structure of matrix fibers in human lial sheath (Fig. 12). In brief, the epithelial sheath bends cementum. J Stomtol Soc Jpn 1987;54:635—75 [in Japanese toward the dental papilla and forms a tapered cylinder. with English abstract]. During root formation the epithelial sheath maintains the Yamamoto T, Domon T, Takahashi S, Wakita M. Formation of alternate lamellar pattern in the advanced cellular cemento- tapered shape and proliferates only at the apical end. In genesis in human teeth. Anat Embryol 1997;196:115—21. contrast, the root grows quite fast and almost straight. In Yamamoto T, Domon T, Takahashi S, Islam N, Suzuki R. Twisted this situation, the discrepancy in surface area could be gen- plywood structure of an alternating lamellar pattern in cellular erated between the epithelial sheath and the growing root. cementum of human teeth. Anat Embryol 2000;202:25—30. As a result, the epithelial sheath is stretched out and cov- Yamamoto T, Li M, Liu Z, Guo Y, Hasegawa T, Masuki H, et al. ers only small portions of the root surface. Accordingly, the Histological review of the human cellular cementum with spe- cell numbers are observed to reduce in histological sections, cial reference to an alternating lamellar pattern. Odontology although the actual cell number is unchanged. 2010;98:102—9. In concluding this section, the classical mesenchymal Giraud-Guille MM. Twisted plywood architecture of collagen hypothesis is still convincing, namely cementoblasts derive fibrils in human compact bone osteons. Calcif Tissue Int 1988;42:167—80. from dental follicle and no epithelial sheath cells transform Ziv V, Sabanay I, Arad T, Traub W, Weiner S. Transitional struc- into cementoblasts. tures in lamellar bone. Microsc Res Tech 1996;33:203—13. Weiner S, Traub W, Wagner HD. Lamellar bone: structure- function relations. J Struct Biol 1999;126:241—55. Conflict of interest Bencze L. Befunde an der Dentinzementgrenze. Z Stomatol 1927;25:877—96. None. Hopewell-Smith A. Concerning human cementum. J Dent Res 1920;2:59—76. Avery JK, Chiergo Jr DJ. Cementum. In: Avery JK, Chiergo Jr DJ, editors. Essential of oral histology and embryology. A clinical References approach. 3rd ed. St. Louis: Mosby Inc.; 2006. p. 137—44. Owens PDA. Light microscopic observations on the formation Denton GB. The discovery of cementum. J Dent Res of the layer of Hopewell-Smith in human teeth. Arch Oral Biol 1939;18:239. 1972;17:1785—8. Jones SJ. Cement. In: Osborn JW, editor. Dental anatomy and Owens PDA. The root surface in human teeth: a microradio- embryology. Oxford: Blackwell; 1981. p. 193—205. graphic study. J Anat 1976;122:389—401. Schroeder HE. Cementum. In: Schroeder HE, editor. The peri- Yamamoto T. The structure and origin of Hopewell-Smith Hya- odontium. Berlin: Springer; 1986. p. 23—127. line layer and intermediate cementum in human and rat teeth. Schroeder HE. Biological problems of regenerative cemento- J Jpn Soc Periodontol 2000;42:298—306 [in Japanese with genesis: synthesis and attachment of collagenous matrices English abstract]. on growing and established root surface. Int Rev Cytol Kawasaki K. On the configuration of incremental lines in 1992;142:1—59. human dentine as revealed by tetracycline labelling. J Anat Schroeder HE. Human cellular mixed stratified cementum: a 1975;119:61—6. tissue with alternating layers of acellular extrinsic- and cel- Lindskog S. Formation of intermediate cementum. I. Early min- lular intrinsic fiber cementum. Schweiz Monatsschr Zahnmed eralization of aprismatic enamel and intermediate cementum 1993;103:550—60. in monkey. J Craniofac Genet Dev Biol 1982;2:147—60. 74 T. Yamamoto et al. Lindskog S. Formation of intermediate cementum. II. A scan- Yamamoto T, Hinrichsen KV. The development of cellular ning electron microscopic study of the epithelial root sheath of cementum in rat molars, with special reference to the fiber Hertwig in monkey. J Craniofac Genet Dev Biol 1982;2:161—9. arrangement. Anat Embryol 1993;188:537—49. Lindskog S, Hammarström L. Formation of intermediate Yamamoto T, Domon T, Takahashi S, Wakita M. Cellular cemen- cementum. III. 3 H-tryptophan and 3 H-proline uptake into the togenesis in rat molars: the role of cementoblasts in the epithelial root sheath of Hertwig in vitro. J Craniofac Genet deposition of intrinsic matrix fibers of cementum proper. Anat Dev Biol 1982;2:171—7. Embryol 1996;193:495—500. Owens PDA. A light and electron microscopic study of the early Cho MI, Garant PR. Development and general structure of the stages of root surface formation in molar teeth in the rat. Arch periodontium. Periodontology 2000 2000;24:9—27. Oral Biol 1980;24:901—7. Diekwisch TGH. The developmental biology of cementum. Int Yamamoto T, Wakita M. Initial attachment of principal fibers J Dev Biol 2001;45:695—706. to the root dentin surface in rat molars. J Periodontal Res Luan X, Ito Y, Diekwisch TGH. Evolution and development 1990;25:113—9. of Hertwig’s epithelial root sheath. Dev Dyn 2006;235: Paynter KJ, Pudy G. A study of the structure, chemical 1167—80. nature, and development of cementum in the rat. Anat Rec Yamamoto T, Yamamoto T, Yamada T, Hasegawa T, Hongo H, Oda 1958;131:233—51. K, et al. Hertwig’s epithelial root sheath cell behavior during Yamamoto T. The innermost layer of cementum in rat molars: initial acellular cementogenesis in rat molars. Histochem Cell its ultrastructure, development, and calcification. Arch Histol Biol 2014;142:489—96. Jpn 1986;49:459—81. Yamamoto T, Yamada T, Yamamoto T, Hasegawa T, Hongo H, Oda Lester KS. The incorporation of epithelial cells by cementum. K, et al. Hertwig’s epithelial root sheath fate during initial cel- J Ultrastruct Res 1969;27:63—87. lular cementogenesis in rat molars. Acta Histochem Cytochem Lester KS. The unusual nature of root formation in molar teeth 2015;48:95—101. of the laboratory rat. J Ultrastruct Res 1969;28:481—506. Fitchett JE, Hay ED. Medial edge transform to mes- Fujita K. Cementum. In: Fujita K, editor. Histology of tooth. enchyme after embryonic palatal shelves fuse. Dev Biol 1st ed. Tokyo: Ishiyaku Shuppan; 1983. p. 133—46. 1989;131:455—74. Birkedal-Hansen H, Butler WT, Taylor RE. Proteins of the peri- Gibbins JR, Manthey A, Tazawa YM, Scott B, Bloch-Zupan odontium. Characterization of the insoluble collagens of bovine A, Hunter N. Midline fusion in the formation of secondary dental cementum. Calcif Tissue Res 1977;23:39—44. palate anticipated by upregulation of keratinK5/6 and local- Christner P, Robinson P, Clark CC. A preliminary charac- ized expression of vimentin mRNA in medial edge epithelium. terization of human cementum collagen. Calcif Tissue Res Int J Dev Biol 1999;43:237—44. 1977;23:147—50. Thomas HF. Root formation. Int J Dev Biol 1995;39:231—7. Ababneh KT, Hall RC, Embry G. Immunolocalization of gly- Zeichner-David M, Oishi K, Su Z, Zakartchenco V, Chen LS, cosaminoglycans in aging, healthy and periodontally diseased Arzate H, et al. Role of Hertwig’s epithelial root sheath cells human cementum. Arch Oral Biol 1998;43:235—46. in tooth root development. Dev Dyn 2003;228:651—63. Ababneh KT, Hall RC, Embery G. The proteoglycans of human Sonoyama W, Seo BM, Yamaza T, Shi S. Human Hertwig’s epithe- cementum: immunohistochemical localization in healthy, lial root sheath cells play crucial roles in cementum formation. periodontally involved and aging teeth. J Periodontal Res J Dent Res 2007;86:594—9. 1999;34:87—96. Akimoto T, Fujiwara N, Kagiya T, Otsu K, Ishizeki K, Harada H. Hammarström L. Origins of cementum. Oral Dis 1996;2:63—9. Establishment of Hertwig’s epithelial root sheath cell line from Hammarström L. Enamel-matrix, cementum development and cells involved in epithelial-mesenchymal transition. Biochem regeneration. J Clin Periodontol 1997;24:658—68. Biophys Res Commun 2011;404:308—12. Foster BL, Popowics TE, Fong HK, Somerman MJ. Advances in Webb PP, Moxham BJ, Benjamin M, Ralphs JR. Changing expres- defining regulators of cementum development and periodontal sion of intermediate filaments in fibroblasts and cementoblasts regeneration. Curr Top Dev Biol 2007;78:47—126. of the developing periodontal ligament of the rat molar teeth. Ascenzi A, Bonnuci E. The compressive properties of single J Anat 1996;188:529—39. osteons. Anat Rec 1968;161:377—92. Lézot F, Davideau J-L, Thomas B, Sharpe P, Forest N. Epithelial Bosshardt DD, Schroeder HE. Evidence for rapid multipolar Dlx-2 homeogene expression and cementogenesis. J Histochem and slow unipolar production of human cellular and acellu- Cytochem 2000;48:277—83. lar cementum matrix with intrinsic fibers. J Clin Periodontol Huang X, Bringas Jr P, Slavkin HC, Chai Y. Fate of 1990;17:663—8. HERS during tooth root development. Dev Biol 2009;334: Yamamoto T, Wakita M. Bundle formation of principal fibers in 22—30. rat molars. J Periodontal Res 1992;27:20—7. Hirata A, Nakamura H. Localization of perlecan and heparanase Yamamoto T, Domon T, Takahashi S, Islam N, Suzuki R, Wakita in Hertwig’s epithelial root sheath during root formation in M. The structure and function of periodontal ligament cells in mouse molars. J Histochem Cytochem 2006;54:1105—13. acellular cementum in rat molars. Ann Anat 1998;180:519—22. Yamamoto T, Domon T, Takahashi S, Anjuman KAY, Fukushima C, Sequeira P, Bosshardt DD, Schroeder HE. Growth of acellu- Wakita M. Mineralization process during acellular cementoge- lar extrinsic fiber cementum (AEFC) and density of inserting nesis in rat molars: a histochemical and immunohistochemical fibers in human premolars of adolescents. J Periodontal Res study using fresh-frozen sections. Histochem Cell Biol 1992;27:134—42. 2007;127:303—11. Yamamoto T, Hasegawa T, Sasaki M, Hongo H, Tabata C, Liu Yamamoto T, Takahashi S. Hertwig’s epithelial root sheath cells Z, et al. Structure and formation of the twisted plywood pat- do not transform into cementoblasts in rat molar cementoge- tern of collagen fibrils in rat lamellar bone. J Electron Microsc nesis. Ann Anat 2009;191:547—55. 2012;61:113—21. Kaneko H, Hashimoto S, Enokiya Y, Ogiuchi H, Shimono M. Cell Matsuo A. Study of the lamellar structure of cementum, its proliferation and death of Hertwig’s epithelial root sheath in degree of mineralization and fibrous components by light and the rat. Cell Tissue Res 1999;298:95—103. scanning electron microscopy and by contact microradiogra- Cerri PS, Freymüller E, Katchburian E. Apoptosis in the phy. Higashi Nippon Dent J 1993;12:193—217 [in Japanese with early developing periodontium of rat molars. Anat Rec English abstract]. 2000;255:136—44.