Molecular Biology Introduction WS24/25 PDF
Document Details
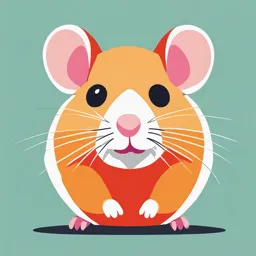
Uploaded by ProductiveSard6609
University of Salzburg
2024
Elfriede Dall
Tags
Summary
This document presents an introduction to Molecular Biology, specifically aimed at the winter semester of 2024/2025 at the University of Salzburg. It outlines the course structure, recommended readings, and a timeframe for examinations.
Full Transcript
STEOP Einführung in die Molekularen Biowissenschaften WINTERSEMESTER 24/25 Prof. Dr. Elfriede Dall Hellbrunnerstrasse 34 [email protected] Studieneingangs- und Orientierungsphase 1. Der Studienplan –siehe File in PLUS online 2. Das erste Semester in der Übersicht...
STEOP Einführung in die Molekularen Biowissenschaften WINTERSEMESTER 24/25 Prof. Dr. Elfriede Dall Hellbrunnerstrasse 34 [email protected] Studieneingangs- und Orientierungsphase 1. Der Studienplan –siehe File in PLUS online 2. Das erste Semester in der Übersicht 2 Klassische/ Molekulare/Zellbiolo Molekulare Genetik gie Molekulare Biowissenschaften Chemie/Biochemie Biophysik 3 Buchempfehlung Begleitwerk für den ganzen Bachelor und Master Alberts et. al. Essential Cell Biology, 5e,2018 4 Allgemeine Informationen Prüfungstermine: Erster Prüfungstermin: Mitte Jänner Zweiter Prüfungstermin: Ende Jänner Dritter Prüfungstermin: Anfang Februar Multiple-Choice (Single Choice) Format, auf DEUTSCH 5 Inhalt der Vorlesung Vorlesung Datum Inhalt VO 1 Concept of Scales VO 2 Concept of the Cell VO 3 Concept of Chemical Bonds VO 3 Concept of Acids and Bases VO 4 Concept of Chemical Components of the Cell VO 5 Concept of Energy VO 6 Concept of Information flow in the cell 6 Chapter 1 Cells: The fundamental Units of Life UNITY AND BACKGROUND AND THE EUKARYOTIC MODELL ESSENTIAL DIVERSITY OF CELLS SCALES CELL ORGANISMS CONCEPTS 7 Figure 1–1 Cells come in a variety of shapes and sizes. Note the very different scales of these micrographs. (A) Drawing of a single nerve cell from a mammalian brain. This cell has a single, unbranched extension (axon), projecting toward the top of the image, through which it sends electrical signals to other nerve cells, and it possesses a huge branching tree of projections (dendrites) through which it receives signals from as many as 100,000 other nerve cells. (B) Paramecium. This protozoan—a single giant cell—swims by means of the beating cilia that cover its surface. (C) The surface of a snapdragon flower petal displays an orderly array of tightly packed cells. (D) A macrophage spreads itself out as it patrols animal tissues in search of invading microorganisms. (E) A fission yeast is caught in the act of dividing in two. The medial septum (stained red with a fluorescent dye) is forming a wall between the two nuclei (also stained red) that have been separated into the two daughter cells; in this image, the cells’ membranes are stained with a green fluorescent dye. Alberts et. al. Essential Cell Biology, 5e,2018 Unity and Diversity of Cell 8 How big are cells and their components? Figure 1–9 How big are cells and their components? (A) This chart lists sizes of cells and their component parts, the units in which they are measured, and the instruments needed to visualize them. (B) Drawings convey a sense of scale between living cells and atoms. Each panel shows an image that is magnified by a factor of 10 compared to its predecessor—producing an imaginary progression from a thumb, to skin, to skin cells, to a mitochondrion, to a ribosome, and ultimately to a cluster of atoms forming part of one of the many protein molecules in our bodies. Note that ribosomes are present inside mitochondria (as shown here), as well as in the cytoplasm. Details of molecular structure, as shown in the last two bottom panels, are beyond the power of the electron microscope. Alberts et. al. Essential Cell Biology, 5e,2018 9 Alberts et. al. Essential Cell Biology, 5e,2018 10 Alberts et. al. Essential Cell Biology, 5e,2018 11 Background and Scales Alberts et. al. Essential Cell Biology, 5e,2018 Background and Scales 12 Background and Scales Alberts et. al. Essential Cell Biology, 5e,2018 Background and Scales 13 Mole and Concentration Alberts et. al. Essential Cell Biology, 5e,2018 Background and Scales 14 15 The Eukaryotic cell bigger and more elaborate than bacteria and archaea By definition, all eukaryotic cells have a nucleus possession of a nucleus goes hand-in-hand with possession of a variety of other organelles, most of which are membrane-enclosed and common to all eukaryotic organisms In this section, we take a look at the main organelles found in eukaryotic cells from the point of view of their functions, and we consider how they came to serve the roles they have in the life of the eukaryotic cell. Alberts et. al. Essential Cell Biology, 5e,2018 16 Nucleus Figure 1–15 The nucleus contains most of the DNA in a eukaryotic cell. (A) This drawing of a typical animal cell shows its extensive system of membrane-enclosed organelles. The nucleus is colored brown, the nuclear envelope is green, and the cytoplasm (the interior of the cell outside the nucleus) is white. (B) An electron micrograph of the nucleus in a mammalian cell. Individual chromosomes are not visible because at this stage of the cell-division cycle the DNA molecules are dispersed as fine threads throughout the nucleus. Alberts et. al. Essential Cell Biology, 5e,2018 17 Mitochondria Figure 1–18 Mitochondria have a distinctive internal structure. (A) An electron micrograph of a cross section of a mitochondrion reveals the extensive infolding of the inner membrane. (B) This three-dimensional representation of the arrangement of the mitochondrial membranes shows the smooth outer membrane (gray) and the highly convoluted inner membrane (red). The inner membrane contains most of the proteins responsible for energy production in eukaryotic cells; it is highly folded to provide a large surface area for this activity. (C) In this schematic cell, the innermost compartment of the mitochondrion is colored orange. Alberts et. al. Essential Cell Biology, 5e,2018 18 Endoplasmic Reticulum (ER) Figure 1–22 The endoplasmic reticulum produces many of the components of a eukaryotic cell. (A) Schematic diagram of an animal cell shows the endoplasmic reticulum (ER) in green. (B) Electron micrograph of a thin section of a mammalian pancreatic cell shows a small part of the ER, of which there are vast amounts in this cell type, which is specialized for protein secretion. Note that the ER is continuous with the membranes of the nuclear envelope. The black particles studding the region of the ER (and nuclear envelope) shown here are ribosomes, structures that translate RNAs into proteins. Because of its appearance, ribosome-coated ER is often called “rough ER” to distinguish it from the “smooth ER,” which does not have ribosomes bound to it. Alberts et. al. Essential Cell Biology, 5e,2018 19 Golgi apparatus Figure 1–23 The Golgi apparatus is composed of a stack of flattened, membrane-enclosed discs. (A) Schematic diagram of an animal cell with the Golgi apparatus colored red. (B) More realistic drawing of the Golgi apparatus. Some of the vesicles seen nearby have pinched off from the Golgi stack; others are destined to fuse with it. Only one stack is shown here, but several can be present in a cell. (C) Electron micrograph that shows the Golgi apparatus from a typical animal cell. Alberts et. al. Essential Cell Biology, 5e,2018 20 Figure 1–24 Membrane-enclosed organelles are distributed throughout the eukaryotic cell cytoplasm. (A) The various types of membrane-enclosed organelles, shown in different colors, are each specialized to perform a different function. (B) The cytoplasm that fills the space outside of these organelles is called the cytosol (colored blue). Alberts et. al. Essential Cell Biology, 5e,2018 21 Cytosol Figure 1–26 The cytosol is extremely crowded. This atomically detailed model of the cytosol of E. coli is based on the sizes and concentrations of 50 of the most abundant large molecules present in the bacterium. RNAs, proteins, and ribosomes are shown in different colors Movie: https://util.wwnorton.com/jwplayer?type=video&msrc=/wwnorton.college.protected/bi ology/legacyanimations/ecb5_0102_cytoplasmic_dynamics.mp4&isrc=/wwnorton.colle ge.protected/biology/legacyanimations/ecb5_0102_cytoplasmic_dynamics.jpg&csrc=/ wwnorton.college.protected/biology/legacyanimations/ecb5_0102_cytoplasmic_dyna mics.vtt (From S.R. McGuffee and A.H. Elcock, PLoS Comput. Biol.6:e1000694, 2010.) Alberts et. al. Essential Cell Biology, 5e,2018 22 Cytoskeleton Figure 1–27 The cytoskeleton is a network of protein filaments that can be seen criss-crossing the cytoplasm of eukaryotic cells. The three major types of filaments can be detected using different fluorescent stains. Shown here are (A) actin filaments, (B) microtubules, and (C) intermediate filaments. Intermediate filaments are not found in the cytoplasm of cells with cell walls, such as plant cells. (A, Molecular Expressions at Florida State University; B, courtesy of Nancy Kedersha; C, courtesy of Clive Lloyd.) Alberts et. al. Essential Cell Biology, 5e,2018 23 Yeast Saccharomyces cerevisiae is a model eukaryote Figure 1–14 Yeasts are simple, free-living eukaryotes. The cells shown in this micrograph belong to the species of yeast, Saccharomyces cerevisiae, used to make dough rise and turn malted barley juice into beer. As can be seen in this image, the cells reproduce by growing a bud and then dividing asymmetrically into a large mother cell and a small daughter cell; for this reason, they are called budding yeast. Alberts et. al. Essential Cell Biology, 5e,2018 24 Fruit Fly Drosophila melanogaster Figure 1–34 Drosophila melanogaster is a favorite among developmental biologists and geneticists. Molecular genetic studies on this small fly have provided a key to the understanding of how all animals develop. Alberts et. al. Essential Cell Biology, 5e,2018 25 Model organisms Alberts et. al. Essential Cell Biology, 5e,2018 26 Essential Concepts of Chapter 1 Cells are the fundamental units of life. All present-day cells are believed to have evolved from an ancestral cell that existed more than 3 billion years ago. All cells are enclosed by a plasma membrane, which separates the inside of the cell from its environment. All cells contain DNA as a store of genetic information and use it to guide the synthesis of RNA molecules and proteins. This molecular relationship underlies cells’ ability to self-replicate. Cells in a multicellular organism, though they all contain the same DNA, can be very different because they turn on different sets of genes according to their developmental history and to signals they receive from their environment. Animal and plant cells are typically 5–20 μm in diameter and can be seen with a light microscope, which also reveals some of their internal components, including the larger organelles. The simplest of present-day living cells are prokaryotes—bacteria and archaea: although they contain DNA, they lack a nucleus and most other organelles and probably resemble most closely the original ancestral cell. Different species of prokaryotes are diverse in their chemical capabilities and inhabit an amazingly wide range of habitats. Eukaryotic cells possess a nucleus and other organelles not found in prokaryotes. They probably evolved in a series of stages, including the acquisition of mitochondria by engulfment of aerobic bacteria and (for cells that carry out photosynthesis) the acquisition of chloroplasts by engulfment of photosynthetic bacteria. The nucleus contains the main genetic information of the eukaryotic organism, stored in very long DNA molecules. Alberts et. al. Essential Cell Biology, 5e,2018 27 Essential Concepts of Chapter 1 The cytoplasm of eukaryotic cells includes all of the cell’s contents outside the nucleus and contains a variety of membrane-enclosed organelles with specialized functions: mitochondria carry out the final oxidation of food molecules and produce ATP; the endoplasmic reticulum and the Golgi apparatus synthesize complex molecules for export from the cell and for insertion in cell membranes; lysosomes digest large molecules; in plant cells and other photosynthetic eukaryotes, chloroplasts perform photosynthesis. Outside the membrane-enclosed organelles in the cytoplasm is the cytosol, a highly concentrated mixture of large and small molecules that carry out many essential biochemical processes. The cytoskeleton is composed of protein filaments that extend throughout the cytoplasm and are responsible for cell shape and movement and for the transport of organelles and large molecular complexes from one intracellular location to another. Free-living, single-celled eukaryotic microorganisms are complex cells that, in some cases, can swim, mate, hunt, and devour other microorganisms. Animals, plants, and some fungi are multicellular organisms that consist of diverse eukaryotic cell types, all derived from a single fertilized egg cell; the number of such cells cooperating to form a large, multicellular organism such as a human runs into thousands of billions. Biologists have chosen a small number of model organisms to study intensely, including the bacterium E. coli, brewer’s yeast, a nematode worm, a fly, a small plant, a fish, mice, and humans themselves. The human genome has about 19,000 protein-coding genes, which is about five times as many as E. coli and about 5000 more than the fly. Alberts et. al. Essential Cell Biology, 5e,2018 28 Schlüssel-Konzepte Zellen sind die grundlegenden Einheiten des Lebens. Es wird angenommen, dass sich alle heutigen Zellen aus einer Stammzelle entwickelt haben, die vor mehr als 3 Milliarden Jahren existierte. Alle Zellen sind von einer Plasmamembran umgeben, die das Innere der Zelle von ihrer Umgebung trennt. Alle Zellen enthalten DNA als Speicher für genetische Informationen und steuern damit die Synthese von RNA- Molekülen und -Proteinen. Diese molekulare Beziehung liegt der Fähigkeit der Zellen zugrunde, sich selbst zu replizieren. Zellen in einem mehrzelligen Organismus können, obwohl sie alle dieselbe DNA enthalten, sehr unterschiedlich sein, da sie je nach ihrer Entwicklungsgeschichte und den Signalen, die sie von ihrer Umgebung erhalten, unterschiedliche Sätze von Genen aktivieren. Tier- und Pflanzenzellen haben typischerweise einen Durchmesser von 5–20 μm und können mit einem Lichtmikroskop gesehen werden, das auch einige ihrer inneren Komponenten, einschließlich der größeren Organellen, sichtbar macht. Die einfachsten heutigen lebenden Zellen sind Prokaryoten - Bakterien und Archaeen: Obwohl sie DNA enthalten, fehlen ihnen ein Kern und die meisten anderen Organellen und sie ähneln wahrscheinlich am ehesten der ursprünglichen Stammzelle. Verschiedene Arten von Prokaryoten unterscheiden sich in ihren chemischen Fähigkeiten und bewohnen ein erstaunlich breites Spektrum an Lebensräumen. Eukaryontische Zellen besitzen einen Kern und andere Organellen, die in Prokaryoten nicht vorkommen. Sie haben sich wahrscheinlich in einer Reihe von Stadien entwickelt, einschließlich des Erwerbs von Mitochondrien durch Aufnahme von aeroben Bakterien und (für Zellen, die Photosynthese durchführen) des Erwerbs von Chloroplasten durch Aufnahme von photosynthetischen Bakterien. Der Kern enthält die wichtigsten genetischen Informationen des eukaryotischen Organismus, die in sehr langen DNA-Molekülen gespeichert sind. Alberts et. al. Essential Cell Biology, 5e,2018 29 Schlüssel-Konzepte Das Zytoplasma eukaryotischer Zellen umfasst den gesamten Zellinhalt außerhalb des Zellkerns und enthält eine Vielzahl von membranumschlossenen Organellen mit speziellen Funktionen: Mitochondrien führen die endgültige Oxidation von Lebensmittelmolekülen durch und produzieren ATP; das endoplasmatische Retikulum und der Golgi-Apparat synthetisieren komplexe Moleküle für den Export aus der Zelle und für die Insertion in Zellmembranen; Lysosomen verdauen große Moleküle; In Pflanzenzellen und anderen photosynthetischen Eukaryoten führen Chloroplasten eine Photosynthese durch. Außerhalb der membranumschlossenen Organellen im Zytoplasma befindet sich das Zytosol, eine hochkonzentrierte Mischung aus großen und kleinen Molekülen, die viele wesentliche biochemische Prozesse ausführen. Das Zytoskelett besteht aus Proteinfilamenten, die sich über das gesamte Zytoplasma erstrecken und für die Zellform und -bewegung sowie für den Transport von Organellen und großen Molekülkomplexen von einem intrazellulären Ort zum anderen verantwortlich sind. Frei lebende, einzellige eukaryotische Mikroorganismen sind komplexe Zellen, die in einigen Fällen schwimmen, sich paaren, jagen und andere Mikroorganismen verschlingen können. Tiere, Pflanzen und einige Pilze sind mehrzellige Organismen, die aus verschiedenen eukaryotischen Zelltypen bestehen, die alle aus einer einzigen befruchteten Eizelle stammen. Die Anzahl solcher Zellen, die zusammenarbeiten, um einen großen, vielzelligen Organismus wie einen Menschen zu bilden, beläuft sich auf Tausende von Milliarden. Biologen haben eine kleine Anzahl von Modellorganismen ausgewählt, die intensiv untersucht werden sollen, darunter das Bakterium E. coli, Bierhefe, ein Nematodenwurm, eine Fliege, eine kleine Pflanze, ein Fisch, Mäuse und Menschen selbst. Das menschliche Genom hat ungefähr 19.000 Protein-kodierende Gene, das ist ungefähr fünfmal so viel wie E. coli und ungefähr 5000 mehr als die Fliege. Alberts et. al. Essential Cell Biology, 5e,2018 30 “The structure and function of a living cell are dictated by the laws of chemistry, physics, and thermodynamics.” Alberts et. al. Essential Cell Biology, 5e,2018 31 Chapter 2 The Chemistry behind it all CHEMICAL BONDS ACIDS AND BASES 32 Periodic table of elements Alberts et. al. Essential Cell Biology, 5e,2018 33 Calculate with Mol atomic weight = The mass of an atom relative to the mass of a hydrogen atom; equal to the number of protons plus the number of neutrons that the atom contains molecular weight = Sum of the atomic weights of the atoms in a molecule; as a ratio of molecular masses, it is a number without units. 1 Mol = 6 x 1023 molecules of the substance Alberts et. al. Essential Cell Biology, 5e,2018 34 Chemical Bonds Matter is made of combinations of elements—substances such as The characteristics of substances hydrogen or carbon that cannot be other than pure elements—including broken down or interconverted by the materials from which living cells chemical means. The smallest are made—depend on which atoms particle of an element that still they contain and the way that these retains its distinctive chemical atoms are linked together in groups properties is an atom. to form molecules. To understand living organisms, therefore, it is crucial to know how the chemical bonds that hold atoms together in molecules are formed. Alberts et. al. Essential Cell Biology, 5e,2018 35 An atom consists of a nucleus surrounded by an electron cloud Figure 2–2 The number of protons in an atom determines its atomic number. Schematic representations of an atom of carbon and an atom of hydrogen are shown. The nucleus of every atom except hydrogen consists of both positively charged protons and electrically neutral neutrons; the atomic weight equals the number of protons plus neutrons. The number of electrons in an atom is equal to the number of protons, so that the atom has no net charge. The electrons are shown here as individual particles. The concentric black circles represent in a highly schematic form the “orbits” (that is, the different distributions) of the electrons. The neutrons, protons, and electrons are in reality minuscule in relation to the atom as a whole; their size is greatly exaggerated here. Alberts et. al. Essential Cell Biology, 5e,2018 36 Geometry Figure 2–9 Covalent bonds are characterized by particular geometries. (A) The spatial arrangement of the covalent bonds that can be formed by oxygen, nitrogen, and carbon. (B) Molecules formed from these atoms therefore have precise three- dimensional structures defined by the bond angles and bond lengths for each covalent linkage. A water molecule, for example, forms a “V” shape with an angle close to 109°. In these ball-and-stick models, the different colored balls represent different atoms, and the sticks represent the covalent bonds. The colors traditionally used to represent the different atoms—black (or dark gray) for carbon, white for hydrogen, blue for nitrogen, and red for oxygen—were established by the chemist August Wilhelm Hofmann in 1865, when he used a set of colored croquet balls to build molecular models for a public lecture on “the combining power of atoms.” Alberts et. al. Essential Cell Biology, 5e,2018 37 Covalent Bonds Form by Sharing of Electrons Figure 2–8 The hydrogen molecule is held together by a covalent bond. Each hydrogen atom in isolation has a single electron, which means that its first (and only) electron shell is incompletely filled. By coming together to form a hydrogen molecule (H2, or hydrogen gas), the two atoms are able to share their electrons, so that each obtains a completely filled first shell, with the shared electrons adopting modified orbits around the two nuclei. The covalent bond between the two atoms has a defined length—0.074 nm, which is the distance between the two nuclei. If the atoms were closer together, the positively charged nuclei would repel each other; if they were farther apart, they would not be able to share electrons as effectively. Alberts et. al. Essential Cell Biology, 5e,2018 38 Distribution of electrons Figure 2–11 In polar covalent bonds, the electrons are shared unequally. Comparison of electron distributions in the polar covalent bonds in a molecule of water (H2O) and the nonpolar covalent bonds in a molecule of oxygen (O2). In H2O, electrons are more strongly attracted to the oxygen nucleus than to the H nucleus, as indicated by the distributions of the partial negative (δ–) and partial positive (δ+) charges. Alberts et. al. Essential Cell Biology, 5e,2018 39 Ionic Bonds Figure 2–12 Sodium chloride is held together by ionic bonds. (A) An atom of sodium (Na) reacts with an atom of chlorine (Cl). Electrons of each atom are shown in their different shells; electrons in the chemically reactive (incompletely filled) outermost shells are shown in red. The reaction takes place with transfer of a single electron from sodium to chlorine, forming two electrically charged atoms, or ions, each with complete sets of electrons in their outermost shells. The two ions have opposite charge and are held together by electrostatic attraction. (B) The product of the reaction between sodium and chlorine, crystalline sodium chloride, contains sodium and chloride ions packed closely together in a regular array in which the charges are exactly balanced. (C) Color photograph of crystals of sodium chloride. Alberts et. al. Essential Cell Biology, 5e,2018 40 Molecular Interaction Figure 2–14 A large molecule, such as a protein, can bind to another protein through noncovalent interactions on the surface of each molecule. In the aqueous environment of a cell, many individual weak interactions could cause the two proteins to recognize each other specifically and form a tight complex. Shown here is a set of electrostatic attractions between complementary positive and negative charges. Alberts et. al. Essential Cell Biology, 5e,2018 41 Hydrogen Bonds Hydrogen bonds have only about 1/20 the strength of a covalent bond Figure 2–13 Noncovalent hydrogen bonds form between water molecules and between many other polar molecules. (A) A hydrogen bond forms between two water molecules. The slight positive charge associated with the hydrogen atom is electrically attracted to the slight negative charge of the oxygen atom. (B) In cells, hydrogen bonds commonly form between molecules that contain an oxygen or nitrogen. The atom bearing the hydrogen is considered the H-bond donor and the atom that interacts with the hydrogen is the H-bond acceptor Alberts et. al. Essential Cell Biology, 5e,2018 42 Examples of Hydrogen bonds Alberts et. al. Essential Cell Biology, 5e,2018 43 Chemical properties of water How water influences the behavior of biological molecules Molecules of water join together transiently in a hydrogen-bonded lattice. The cohesive nature of water is responsible for many of its unusual properties, such as high surface tension, high specific heat capacity, and high heat of vaporization. Alberts et. al. Essential Cell Biology, 5e,2018 44 Hydrophobic molecules in water Substances that contain a preponderance of nonpolar bonds are usually insoluble in water and are termed hydrophobic. Water molecules are not attracted to such hydrophobic molecules and so have little tendency to surround them and bring them into solution. Hydrocarbons, which contain many C–H bonds, are especially hydrophobic Alberts et. al. Essential Cell Biology, 5e,2018 45 Hydrophilic Molecules in Water Substances that dissolve readily in water are termed hydrophilic. Ionic substances such as sodium chloride dissolve because water molecules are attracted to the positive (Na+) or negative (Cl–) charge of each ion. Polar substances such as urea dissolve because their molecules form hydrogen bonds with the surrounding water molecules. Alberts et. al. Essential Cell Biology, 5e,2018 46 Parameter of chemical bonds Alberts et. al. Essential Cell Biology, 5e,2018 47 Autoproteolysis of water Water can react as acid or as base in proteolysis Even multiply distilled water (= very pure water) has a specific conductivity, which would not be possible without the presence of the ions (H3O+ and OH-). Like any protolysis, the autoprotolysis of water is an equilibrium reaction, with the equilibrium being strongly left. 48 The law of mass action for the autoproteolysis of water. 49 The Ion product of water Although the equilibrium exists, the number of water particles, i.e. the concentration of water in this container, has not changed noticeably. Therefore, the concentration of water can be considered constant and is therefore shifted to the side of the constant K. This results in a new constant Kw, which is called the ion product of water. 50 Acids Substances that release hydrogen ions (protons) into solution are called acids. Alberts et. al. Essential Cell Biology, 5e,2018 51 The Law of Mass action How strong an acid is, depends on the dissociation equilibrium of the acidic aqueous solution on the product side. Strong acids dissociate completely to their ions. Applying the law of mass action to the protolysis equilibria leads us to a generally valid characterization of acid strengths. We consider the general case where an acid HA protolyses. 52 Acid constant Säurekonstante Ks As we always look at a diluted aqueous acid, it changes the water concentration by adjusting this equilibrium only so little that the concentration of water can be considered constant. Thus, [H2O] is drawn to the side of the constant K and combined to the acid constant Ks. Note: The constant Ks is a measure for the strength of an acid and is called the acid constant. The greater the Ks, the stronger the acid. 53 Weak Acids Many of the acids important in the cell are not completely dissociated, and they are therefore weak acids—for example, the carboxyl group (–COOH), which dissociates to give a hydrogen ion in solution. Note that this is a reversible reaction. Alberts et. al. Essential Cell Biology, 5e,2018 54 Hydrogen Ion Exchange Positively charged hydrogen ions (H+) can spontaneously move from one water molecule to another, thereby creating two ionic species. Pure water contains equal concentrations of hydronium ions and hydroxyl ions (both 10–7 M). Alberts et. al. Essential Cell Biology, 5e,2018 55 Figure 2–15 Protons move continuously from one molecule to another in aqueous solutions. (A) The reaction that takes place when a molecule of acetic acid dissolves in water. At pH 7, nearly all of the acetic acid molecules are present as acetate ions. (B) Water molecules are continually exchanging protons with each other to form hydronium and hydroxyl ions. These ions in turn rapidly recombine to form water molecules. Alberts et. al. Essential Cell Biology, 5e,2018 56 The pH The acidity of a solution is defined by the concentration (conc.) of hydronium ions (H3O+) it possesses, generally abbreviated as H+. For convenience, we use the pH scale. Alberts et. al. Essential Cell Biology, 5e,2018 57 Examples Figure 2–16 In aqueous solutions, the concentration of hydroxyl (OH–) ions increases as the concentration of H3O+ (or H+ ) ions decreases. The product of the two values, [OH–] x [H+], is always 10–14 (moles/liter)2. At neutral pH, [OH–] = [H+], and both ions are present at 10–7 M. Also shown are examples of common solutions along with their Alberts et. al. Essential Cell Biology, 5e,2018 58 Bases Substances that reduce the number of hydrogen ions in solution are called bases. Some bases, such as ammonia, combine directly with hydrogen ions. Alberts et. al. Essential Cell Biology, 5e,2018 59 Bases Other bases, such as sodium hydroxide, reduce the number of H+ ions indirectly, by producing OH– ions that then combine directly with H+ ions to make H2O. Alberts et. al. Essential Cell Biology, 5e,2018 60 Base constant Kb For the protolysis equilibrium of a base B, the base constant Kb is obtained in the same way: Note: The constant Kb is a measure for the strength of a base and is called base constant. The greater KB, the stronger the base. 61 pKb (base exponent) and pKs (acid exponent) All concentrations occurring in the equations of Ks and Kb are equilibrium concentrations. The unit of the constants is mol/L, thus the unit of a concentration. For many acids and bases the KS and KB values have been determined experimentally and tabulated. However, the Kb and Ks values are not written down there, but the pKb (base exponent) and pKs (acid exponent) values are found there. 62 Note Note: The constant Ks is a measure for the strength of an acid and is called acid constant. The higher the Ks or the lower the pKS, the stronger the acid. Note: The constant Kb is a measure for the strength of a base and is called base constant. The larger the KB or the smaller the pK, the stronger the base. 63 Weak bases Many bases found in cells are partially associated with H+ ions and are termed weak bases. This is true of compounds that contain an amino group (—NH2), which has a weak tendency to reversibly accept an H+ ion from water, thereby increasing the concentration of free OH— ions. The interior of a cell is kept close to neutral by the presence of buffers: mixtures of weak acids and bases that will adjust proton concentrations around pH 7 by releasing protons (acids) or taking them up (bases) whenever the pH changes. This give-and-take keeps the pH of the cell relatively constant under a variety of conditions. Alberts et. al. Essential Cell Biology, 5e,2018 64 Chapter 3 Chemical Components of Cells TYPES OF SUGAR FATTY ACIDS AMINO ACIDS NUCLEOTIDES Alberts et. al. Essential Cell Biology, 5e,2018 65 Cellular composition Figure 2–17 Sugars, fatty acids, amino acids, and nucleotides are the four main families of small organic molecules in cells. They form the monomeric building blocks, or subunits, for larger organic molecules, including most of the macromolecules and other molecular assemblies of the cell. Some, like the sugars and the fatty acids, are also energy sources. Alberts et. al. Essential Cell Biology, 5e,2018 66 Distribution by percentage Alberts et. al. Essential Cell Biology, 5e,2018 67 Outline of some of the types of sugar Monosaccharides: General formula (CH2O)n, where n is usually 3, 4, 5,or 6 Alberts et. al. Essential Cell Biology, 5e,2018 68 Alberts et. al. Essential Cell Biology, 5e,2018 69 Ring Formation In aqueous solution, the aldehyde or ketone group of a sugar molecule tends to react with a hydroxyl group of the same molecule, thereby closing the molecule into a ring. Alberts et. al. Essential Cell Biology, 5e,2018 70 Types of display Figure 2–18 The structure of glucose, a monosaccharide, can be represented in several ways. (A) A structural formula in which the atoms are shown as chemical symbols, linked together by solid lines representing the covalent bonds. The thickened lines are used to indicate the plane of the sugar ring and to show that the –H and –OH groups are not in the same plane as the ring. (B) Another kind of structural formula that shows the three- dimensional structure of glucose in a so-called “chair configuration.” (C) A ball-and-stick model in which the three-dimensional arrangement of the atoms in space is indicated. (D) A space-filling model, which, as well as depicting the three-dimensional arrangement of the atoms, also shows the relative sizes and surface contours of the molecule (Movie 2.1). The atoms in (C) and (D) are colored as in Figure 2–9: C, black; H, white; O, red. This is the conventional color-coding for these atoms and will be used throughout this book. Alberts et. al. Essential Cell Biology, 5e,2018 71 Disaccharides The carbon that carries the aldehyde or the ketone can react with any hydroxyl group on a second sugar molecule to form a disaccharide. Three common disaccharides are maltose (glucose + glucose) lactose (galactose + glucose) sucrose (glucose + fructose) Figure 2–19 Two monosaccharides can be linked by a covalent glycosidic bond to form a disaccharide. This reaction belongs to a general category of reactions termed condensation reactions, in which two molecules join together as a result of the loss of a water molecule. The reverse reaction (in which water is added) is termed hydrolysis. Alberts et. al. Essential Cell Biology, 5e,2018 72 Oligosaccharides und Polysaccharides Large linear and branched molecules can be made from simple repeating sugar subunits. Short chains are called oligosaccharides, and long chains are called polysaccharides. Glycogen, for example, is a polysaccharide made entirely of glucose subunits joined together. Alberts et. al. Essential Cell Biology, 5e,2018 73 Complex Oligosaccharides In many cases, a sugar sequence is nonrepetitive. Many different molecules are possible. Such complex oligosaccharides are usually linked to proteins or to lipids, as is this oligosaccharide, which is part of a cell-surface molecule that defines a particular blood group. Alberts et. al. Essential Cell Biology, 5e,2018 74 Fatty Acids Figure 2–21 Fatty acids have both hydrophobic and hydrophilic components. The hydrophobic hydrocarbon chain is attached to a hydrophilic carboxylic acid group. Different fatty acids have different hydrocarbon tails. Palmitic acid is shown here. (A) Structural formula, showing the carboxylic acid head group in its ionized form, as it exists in water at pH 7. (B) Ball-and-stick model. (C) Space-filling model Alberts et. al. Essential Cell Biology, 5e,2018 75 Phospholipids Figure 2–23 Phospholipids can aggregate to form cell membranes. Phospholipids contain two hydrophobic fatty acid tails and a hydrophilic head. (A) Phosphatidylcholine is the most common phospholipid in cell membranes. (B) Diagram showing how, in an aqueous environment, the hydrophobic tails of phospholipids pack together to form a lipid bilayer. In the lipid bilayer, the hydrophilic heads of the phospholipid molecules are on the outside, facing the aqueous environment, and the hydrophobic tails are on the inside, where water is excluded. Alberts et. al. Essential Cell Biology, 5e,2018 76 Families of Amino acids The common amino acids are grouped according to whether their side chains are acidic basic uncharged polar nonpolar These 20 amino acids are given both three-letter and one-letter abbreviations. Thus: alanine = Ala = A R is commonly one of the 20 different side chains , at pH= 7 both the amino and carboxyl group are ionized Alberts et. al. Essential Cell Biology, 5e,2018 77 Amino acids Subunits of Proteins Figure 2–24 All amino acids have an amino group, a carboxyl group, and a side chain (R) attached to their α-carbon atom. In the cell, where the pH is close to 7, free amino acids exist in their ionized form; but, when they are incorporated into a polypeptide chain, the charges on their amino and carboxyl groups are lost. (A) The amino acid shown is alanine, one of the simplest amino acids, which has a methyl group (CH3) as its side chain. Its amino group is highlighted in blue and its carboxyl group in red. (B) A ball-and-stick model and (C) a space-filling model of alanine. In (B) and (C), the N atom is blue and the O atom is red. Alberts et. al. Essential Cell Biology, 5e,2018 78 Peptides Figure 2–25 Amino acids in a protein are held together by peptide bonds. The four amino acids shown are linked together by three peptide bonds, one of which is highlighted in yellow. One of the amino acids, glutamic acid, is shaded in gray. The amino acid side chains are shown in red. The N-terminus of the polypeptide chain is capped by an amino group, and the C-terminus ends in a carboxyl group. The sequence of amino acids in a protein is abbreviated using either a three-letter or a one-letter code, and the sequence is always read starting from the N- terminus (see Panel 2–6, pp. 76–77). In the example given, the sequence is Phe-Ser-Glu-Lys (or FSEK). Alberts et. al. Essential Cell Biology, 5e,2018 79 80 81 https://de.wikipedia.org/wiki/Aminos%C3%A4uren 82 Alberts et. al. Essential Cell Biology, 83 5e,2018 Nucleotides Subunits of DNA and RNA NUCLEOBASES are nitrogen-containing ring compounds, either pyrimidines or purines. Alberts et. al. Essential Cell Biology, 5e,2018 84 Nucleotides A nucleotide consists of a nitrogen-containing base, a five-carbon sugar, and one or more phosphate groups. Nucleotides are the subunits of the nucleic acids Alberts et. al. Essential Cell Biology, 5e,2018 85 Nomenclature The names can be confusing, but the abbreviations are clear. Alberts et. al. Essential Cell Biology, 5e,2018 86 Nucleic acid Figure 5–3 The nucleotide subunits within a DNA strand are held together by phosphodiester bonds. These bonds connect one sugar to the next. The chemical differences in the ester linkages—between the 5ʹ carbon of one sugar and the 3ʹ carbon of the other—give rise to the polarity of the resulting DNA strand. For simplicity, only two nucleotides are shown here. Alberts et. al. Essential Cell Biology, 5e,2018 87 DNA Alberts et. al. Essential Cell Biology, 5e,2018 88 Basic Chemistry in all living Cells Figure 1–2 In all living cells, genetic information flows from DNA to RNA (transcription) and from RNA to protein (translation)—an arrangement known as the central dogma. The sequence of nucleotides in a particular segment of DNA (a gene) is transcribed into an RNA molecule, which can then be translated into the linear sequence of amino acids of a protein. Only a small part of the gene, RNA, and protein is shown. The discoveries of biochemists and molecular biologists have provided an elegant solution to this awkward situation. Although the cells of all living things are enormously varied when viewed from the outside, they are fundamentally similar inside. We now know that cells resemble one another to an astonishing degree in the details of their chemistry. They are composed of the same sorts of molecules, which participate in the same types of chemical reactions (discussed in Chapter 2). In all organisms, genetic information—in the form. Alberts et. al. Essential Cell Biology, 5e,2018 Unity and Diversity of Cell 89 Key terms Alberts et. al. Essential Cell Biology, 5e,2018 90 Essential concepts Living cells obey the same chemical and physical laws as nonliving things. Like all other forms of matter, they are made of atoms, which are the smallest unit of a chemical element that retains the distinctive chemical properties of that element. Cells are made up of a limited number of elements, four of which—C, H, N, O—make up about 96% of a cell’s mass. Each atom has a positively charged nucleus, which is surrounded by a cloud of negatively charged electrons. The chemical properties of an atom are determined by the number and arrangement of its electrons: it is most stable when its outer electron shell is completely filled. A covalent bond forms when a pair of outer-shell electrons is shared between two adjacent atoms; if two pairs of electrons are shared, a double bond is formed. A cluster of two or more atoms held together by covalent bonds is known as a molecule. When an electron jumps from one atom to another, two ions of opposite charge are generated; these ions are held together by mutual attraction, forming a noncovalent ionic bond. Cells are 70% water by weight; the chemistry of life therefore takes place in an aqueous environment. Alberts et. al. Essential Cell Biology, 5e,2018 91 Essential concepts Living organisms contain a distinctive and restricted set of small, carbon-based (organic) molecules, which are essentially the same for every living species. The main categories are sugars, fatty acids, amino acids, and nucleotides. Sugars are a primary source of chemical energy for cells and can also be joined together to form polysaccharides or shorter oligosaccharides. Fatty acids are an even richer energy source than sugars, but their most essential function is to form lipid molecules that assemble into sheet-like cell membranes. The vast majority of the dry mass of a cell consists of macromolecules—mainly polysaccharides, proteins, and nucleic acids (DNA and RNA); these macromolecules are formed as polymers of sugars, amino acids, or nucleotides, respectively. The most diverse and versatile class of macromolecules are proteins, which are formed from 20 types of amino acids that are covalently linked by peptide bonds into long polypeptide chains. Proteins constitute half of the dry mass of a cell. Nucleotides play a central part in energy-transfer reactions within cells; they are also joined together to form information- containing RNA and DNA molecules, each of which is composed of only four types of nucleotides. Protein, RNA, and DNA molecules are synthesized from subunits by repetitive condensation reactions, and it is the specific sequence of subunits that determines their unique functions. Four types of weak noncovalent bonds—hydrogen bonds, electrostatic attractions, van der Waals attractions, and the hydrophobic force—enable macromolecules to bind specifically to other macromolecules or to selected small molecules. Noncovalent bonds between different regions of a polypeptide or RNA chain allow these chains to fold into unique shapes (conformations). Alberts et. al. Essential Cell Biology, 5e,2018 92 Schlüssel-Konzepte Lebende Zellen gehorchen den gleichen chemischen und physikalischen Gesetzen wie nicht lebende Dinge. Wie alle anderen Formen der Materie bestehen sie aus Atomen, die die kleinste Einheit eines chemischen Elements darstellen, das die charakteristischen chemischen Eigenschaften dieses Elements beibehält. Zellen setzen sich aus einer begrenzten Anzahl von Elementen zusammen, von denen vier - C, H, N, O - etwa 96% der Masse einer Zelle ausmachen. Jedes Atom hat einen positiv geladenen Kern, der von einer Wolke negativ geladener Elektronen umgeben ist. Die chemischen Eigenschaften eines Atoms werden durch die Anzahl und Anordnung seiner Elektronen bestimmt: Es ist am stabilsten, wenn seine äußere Elektronenhülle vollständig gefüllt ist. Eine kovalente Bindung bildet sich, wenn sich zwei benachbarte Atome ein Paar Außenhüllen-Elektronen teilen; werden zwei Elektronenpaare geteilt, bildet sich eine Doppelbindung. Ein Cluster aus zwei oder mehr Atomen, die durch kovalente Bindungen zusammengehalten werden, wird als Molekül bezeichnet. Wenn ein Elektron von einem Atom zu einem anderen springt, werden zwei Ionen mit entgegengesetzter Ladung erzeugt; diese Ionen werden durch gegenseitige Anziehung zusammengehalten und bilden eine nichtkovalente Ionenbindung. Zellen bestehen zu 70 Gew.-% aus Wasser; die Chemie des Lebens findet also in einer wässrigen Umgebung statt. Alberts et. al. Essential Cell Biology, 5e,2018 93 Schlüssel-Konzepte Lebende Organismen enthalten eine charakteristische und begrenzte Menge kleiner, auf Kohlenstoff basierender (organischer) Moleküle, die im Wesentlichen für alle lebenden Arten gleich sind. Die Hauptkategorien sind Zucker, Fettsäuren, Aminosäuren und Nukleotide. Zucker sind eine primäre chemische Energiequelle für Zellen und können auch zu Polysacchariden oder kürzeren Oligosacchariden zusammengefügt werden. Fettsäuren sind eine noch reichhaltigere Energiequelle als Zucker, aber ihre wesentlichste Funktion besteht in der Bildung von Lipidmolekülen, die sich zu flächigen Zellmembranen zusammenfügen. Der überwiegende Teil der Trockenmasse einer Zelle besteht aus Makromolekülen - hauptsächlich Polysaccharide, Proteine und Nukleinsäuren (DNA und RNA); diese Makromoleküle werden als Polymere von Zuckern, Aminosäuren bzw. Nukleotiden gebildet. Die vielfältigste und vielseitigste Klasse von Makromolekülen sind die Proteine, die aus 20 Arten von Aminosäuren gebildet werden, die kovalent durch Peptidbindungen zu langen Polypeptidketten verbunden sind. Proteine machen die Hälfte der Trockenmasse einer Zelle aus. Nukleotide spielen eine zentrale Rolle bei Energieübertragungsreaktionen innerhalb von Zellen; sie werden auch zu informationshaltigen RNA- und DNA-Molekülen zusammengefügt, die jeweils nur aus vier Arten von Nukleotiden bestehen. Protein-, RNA- und DNA-Moleküle werden aus Untereinheiten durch sich wiederholende Kondensationsreaktionen synthetisiert, und es ist die spezifische Sequenz der Untereinheiten, die ihre einzigartigen Funktionen bestimmt. Vier Arten schwacher nichtkovalenter Bindungen - Wasserstoffbrückenbindungen, elektrostatische Anziehungskräfte, Van-der-Waals-Anziehungskräfte und die hydrophobe Kraft - ermöglichen es Makromolekülen, spezifisch an andere Makromoleküle oder an ausgewählte kleine Moleküle zu binden. Nichtkovalente Bindungen zwischen verschiedenen Bereichen einer Polypeptid- oder RNA-Kette ermöglichen es diesen Ketten, sich in einzigartige Formen (Konformationen) zu falten. Alberts et. al. Essential Cell Biology, 5e,2018 94 CHAPTER 3 Energy, Catalysis and Biosynthesis THE USE OF ENERGY BY FREE ENERGY AND BIOSYNTHESIS CELL CATALYSIS 95 Figure 3–3 Biological structures are highly ordered. Well-defined, ornate, and beautiful spatial patterns can be found at every level of organization in living organisms. Shown are: (A) protein molecules in the coat of a virus (a parasite that, although not technically alive, contains the same types of molecules as those found in living cells); (B) the regular array of microtubules seen in a cross section of a sperm tail; (C) surface contours of a pollen grain; (D) cross section of a fern stem, showing the patterned arrangement of cells; and (E) a spiral array of leaves, each made of millions of cells. 96 Figure 1–4 Life is an autocatalytic process. DNA and RNA provide the sequence information (green arrows) that is used to produce proteins and to copy themselves. Proteins, in turn, provide the catalytic activity (red arrows) needed to synthesize DNA, RNA, and themselves. Together, these feedback loops create the self-replicating system that endows living cells with their ability to reproduce. Alberts et. al. Essential Cell Biology, 5e,2018 Unity and Diversity of Cell 97 The Use of Energy by Cells Left to themselves, nonliving things eventually become disordered: buildings crumble and dead organisms decay. Living cells, by contrast, not only maintain but actually generate order at every level, from the large-scale structure of a butterfly or a flower down to the organization of the molecules that make up such organisms (Figure 3–3). This property of life is made possible by elaborate molecular mechanisms that extract energy from the environment and convert it into the energy stored in chemical bonds. Biological structures are therefore able to maintain their form, even though the materials that form them are continually being broken down, replaced, and recycled. Your body has the same basic structure it had 10 years ago, even though you now contain atoms that, for the most part, were not part of your body then. 98 The Concept of Free Energy in chemical Reactions 99 Reactions ΔG (“DELTA G”) Changes in free energy occurring in a reaction are denoted by ΔG, where “Δ” indicates a difference. Thus, for the reaction ΔG measures the amount of disorder caused by a reaction: the change in order inside the cell, plus the change in order of the surroundings caused by the heat released. ΔG is useful because it measures how far away from equilibrium a reaction is. 100 Chemical Equilibrium This reversible Reaction will proceed until the ratio of concentrations [C][D]/[A][B] is equal to K (note: square brackets [ ] indicate concentration). At this point, the free energy of the system will have its lowest value. 101 Reaction Rates free-energy change (ΔG) will not necessarily occur rapidly by itself. Consider, for example, the combustion of glucose in oxygen: Even this highly favorable reaction may not occur for centuries unless enzymes are present to speed up the process. Enzymes are able to catalyze reactions and speed up their rate, but they cannot change the ΔG° of a reaction. 102 Free Energy and Catalysis Life depends on the highly specific chemical reactions that take place inside cells. The vast majority of these reactions are catalyzed by proteins called enzymes. To understand how enzymes promote the acceleration of the specific chemical reactions needed to sustain life, we first need to examine the energetics involved. In this section, we consider how the free energy of molecules contributes to their chemistry, and we see how free-energy changes This type of enzyme-assisted catalysis is crucial for cells: without it, life could not exist. 103 Enzymes Reduce the Energy Needed to Initiate Spontaneous Reactions Figure 3–12 Even energetically favorable reactions require activation energy to get them started. (A) Compound Y (a reactant) is in a relatively stable state; thus energy is required to convert it to compound X (a product), even though X is at a lower overall energy level than Y. This conversion will not take place, therefore, unless compound Y can acquire enough activation energy (energy a minus energy b) from its surroundings to undergo the reaction that converts it into compound X. This energy may be provided by means of an unusually energetic collision with other molecules. For the reverse reaction, X → Y, the activation energy required will be much larger (energy a minus energy c); this reaction will therefore occur much more rarely. The total energy change for the energetically favorable reaction Y → X is energy c minus energy b, a negative number, which corresponds to a loss of free energy. (B) Energy barriers for specific reactions can be lowered by catalysts, as indicated by the line marked d. Enzymes are particularly effective catalysts because they greatly reduce the activation energy for the reactions they catalyze. Note that activation energies are always positive. 104 The Equilibrium Constant Also Indicates the Strength of Noncovalent Binding Interactions The concept of free-energy change does not apply only to chemical reactions where covalent bonds are being broken and formed. It is also used to quantitate the strength of interactions in which one molecule binds to another by means of noncovalent interactions Two molecules will bind to each other if the free-energy change for the interaction is negative; that is, the free energy of the resulting complex is lower than the sum of the free energies of the two partners when unbound. Noncovalent interactions are immensely important to cells. They include the binding of substrates to enzymes, the binding of transcription regulators to DNA, and the binding of one protein to another to make the many different structural and functional protein complexes that operate in a living cell. 105 106 Essential Concepts Living organisms are able to exist because of a continual input of energy. Part of this energy is used to carry out essential reactions that support cell metabolism, growth, movement, and reproduction; the remainder is lost in the form of heat. The ultimate source of energy for most living organisms is the sun. Plants, algae, and photosynthetic bacteria use solar energy to produce organic molecules from carbon dioxide. Animals obtain food by eating plants or by eating animals that feed on plants. Each of the many hundreds of chemical reactions that occur in a cell is specifically catalyzed by an enzyme. Large numbers of different enzymes work in sequence to form chains of reactions, called metabolic pathways, each performing a different function in the cell. Enzymes catalyze reactions by binding to particular substrate molecules in a way that lowers the activation energy required for making and breaking specific covalent bonds. The rate at which an enzyme catalyzes a reaction depends on how rapidly it finds its substrates and how quickly the product forms and then diffuses away. These rates vary widely from one enzyme to another. The ΔG for a chemical reaction depends on the concentrations of the reacting molecules, and it may be calculated from these concentrations if the equilibrium constant (K) of the reaction (or the standard free- energy change, ΔG°, for the reactants) is known. Equilibrium constants govern all of the associations (and dissociations) that occur between macromolecules and small molecules in the cell. The larger the binding energy between two molecules, the larger the equilibrium constant and the more likely that these molecules will be found bound to each other. By creating a reaction pathway that couples an energetically favorable reaction to an energetically unfavorable one, enzymes can make otherwise impossible chemical transformations occur. Large numbers of such coupled reactions make life possible. 107 Deine Möglichkeiten an der Universität Salzburg: https://www.uni-salzburg.at/index.php?id=208814 Informiere dich über die verschiedenen Forschungsgruppen am Fachbereich Biowissenschaften und lass dich inspirieren. Auf ein baldiges Wiedersehen! 108