Introduction to Endocrinology BMS200 PDF
Document Details
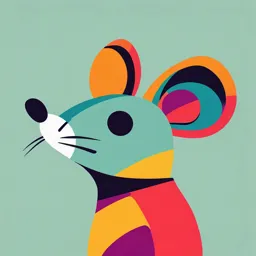
Uploaded by DivineRed
Canadian College of Naturopathic Medicine
2023
Dr. Lakshman, PhD
Tags
Summary
This document is a presentation on Introduction to Endocrinology, BMS200, given on September 16th, 2023. It includes learning outcomes and pre-assessments, as well as detailed anatomical information about the hypothalamus, pituitary, and other endocrine structures.
Full Transcript
Introduction to Endocrinology BMS200 Dr. Lakshman, September 16th, 2023 PhD Learning Outcomes ∙ Describe the functional anatomy of the vascular and non-vascular elements of the endocrine hypothalamus, pituitary gland, thyroid gland, and adrenal glands ∙ Explain the regulation of the hyp...
Introduction to Endocrinology BMS200 Dr. Lakshman, September 16th, 2023 PhD Learning Outcomes ∙ Describe the functional anatomy of the vascular and non-vascular elements of the endocrine hypothalamus, pituitary gland, thyroid gland, and adrenal glands ∙ Explain the regulation of the hypothalamic-pituitary-target gland axis, considering short and long feedback loops. ∙ Describe growth hormone synthesis, transport, function and regulation, including diurnal rhythms of secretion ∙ Analyze the regulation and biological actions of somatomedins in relation to growth hormone secretion. ∙ Predict complications associated with abnormal growth hormone production and function, such as acromegaly and gigantism. ∙ Describe the synthesis, regulation of secretion, and function of prolactin, including its role in cross- talk with other hypothalamic hormones. Pre-Assessment How does negative feedback work? What do you recall about the functionality of the growth hormone? Hypothalamus Anatomy The hypothalamus is composed of different types of neurons that are combined in various nuclei These receive input from various receptors of the body including information regarding thirst, blood pressure, appetite, light-dark, temperature, etc. Hypothalamic neurons send their output to centers in the brain, including pituitary gland Hypothalamic regulation The hypothalamus receives signals from the central nervous system and messengers that travel through the bloodstream: Situated in very close to the 3 ventricle rd there are regions within the 3rd ventricular that allow somewhat selective passage of signals from blood 🡪 ventricular fluid (known as circumventricular organs) Signals can be detected by neurons in the hypothalamic nuclei Can sense osmolarity, glucose, signal peptides (short loop feedback, appetite mediators)… many Extensive communication between the brainstem, limbic areas, and cortex (as already seen during our discussion of homeostatic pathways of appetite regulation) Magnocellular and Parvocellular Neurons Magnocellular neurons are located within the supraoptic and paraventricular nuclei Large, produce large quantities of neurohormones Neurohormones: oxytocin, vasopressin Output: posterior pituitary – release neurohormones into systemic circulation Parvocellular neurons are located within multiple different nuclei Small size Neurohormones: GnRH/LHRH, PRH CRH, TRH, GHRH, GHIH, DA, Output: median eminence (portal vein towards anterior pituitary), brainstem, spinal cord Hypothalamic-pituitary system (AP) The hypothalamus secretes releasing or inhibiting hormones into 1st set of capillaries These travel down to the anterior pituitary and modulate hormone secretion from those cells Anterior pituitary hormones control several other endocrine glands Thyroid, adrenal gland, gonads, liver Cranial anatomy – the hypophyseal fossa Note how the pituitary is almost completely surrounded by bone (sella Basic Function of Hypothalamic Neurohormones Neurohormone Function gnocellular Oxytocin (via posterior pituitary) Stimulate uterine contractions ron Vasopressin (ADH) (via posterior pituitary) Promote water reabsorption Stimulate thirst vocellular Corticotropin Releasing Hormone (CRH) Stimulate ACTH release; AP ron Thyrotropin Releasing Hormone (TRH) Stimulate TSH release; AP Gonadotropin Releasing Hormone (GnRH)/ Luteinizing Stimulate Gonadotropin release (LH Hormone Releasing Hormone (LHRH) and FSH); AP Growth Hormone Inhibiting Hormone (GHIH)/ Inhibit GH release from anterior somatostatin pituitary (AP) Growth Hormone Releasing Hormone (GHRH) Stimulate GH release from anterior pituitary (AP) Prolactin Releasing Hormone (PRH) Stimulate prolactin release; AP Prolactin Inhibiting Hormone (PRIH)/ Dopamine (DA) Inhibit prolactin release; AP Hypothalamus and Pituitary Median Posterior Pituitary eminence Composed of axon terminals Infundibul of magnocellular neurons and arteries forming inferior um hypophyseal artery Anterior Pituitary Composed of endocrine tissues (responsible for producing ACTH, GH, TSH, etc.) Hypothala Receives hypothalamic neurohormones via the secondary capillary plexus that receives mic- blood from portal vein (hypothalamic parvocellular neurons release hormones into the primary capillary plexus within median eminence) hypophyse Superior hypophyseal artery 🡪 primary capillary al tract plexus 🡪 portal vein 🡪 secondary capillary plexus Releases hormones into the hypophyseal veins (into systemic circulation via internal jugular vein) Hypothalamic Regulation Receives input from: CNS, intestines, heart, liver, stomach Contain specialized neurons that are able to detect different senses: glucose-sensing neurons, osmoreceptors Various hormones and signals from periphery can regulate hypothalamus via positive and negative feedback loops Negative loop: CRH stimulates ACTH release from anterior pituitary, ACTH inhibits hypothalamus from releasing more CRH Positive loop: Oxytocin stimulates uterine contractions, fetal head descends and stretches the cervix, triggering hypothalamus to release more oxytocin Hypothalamus is constantly integrating multiple different signals and adjusting its output accordingly Hypothalamic & Pituitary Regulation Most feedback is via negative feedback May be homeostatic or non-homeostatic mechanisms Oxytocin release during childbirth main example of positive feedback (more in BMS 250) – cervical thinning 🡪 oxytocin release 🡪 increased uterine contractions 🡪 increased cervical thinning Definitions: Long loop: target endocrine gland 🡪 hypothalamus or pituitary Short loop: pituitary 🡪 hypothalamus We won’t discuss ultra-short Review: Short and Long Feedback Loops Short loop Hypothalamus CRH GHRH TRH GnRH Pituitary ACTH GH TSH FSH, LH Long loop Target Gland Cortisol Insulin-like growth factor T3, T4 Estrogen, progesterone, testosterone Growth Hormone (GH) Similar in structure to prolactin Produced by somatotrophs within the anterior pituitary Released in pulsatile bursts, major burst at night (nocturnal) during slow- wave sleep Transported with majority bound to growth-hormone binding protein to act as reservoir and to prolong half-life (protects against degradation) Half-life is 6-20 minutes Stimulates insulin-like growth factor 1 (IGF-1) release from liver Most important function: stimulation of postnatal longitudinal growth (anabolic and mitogenic effects) Production increases after 1-2 years, peaks in puberty, begins to decline in adulthood and continues with aging GH Regulation: Stimulation GH secretion is stimulated by: GHRH (hypothalamus) Hypoglycemia (promotes GHRH release) Arginine Catecholamines (also reduce GHIH/somatostatin) Dopamine Cortisol, thyroid hormones and androgens also influence GH by modifying the responsiveness to GHRH and GHIH Ghrelin (stomach, pancreas, kidney, liver, hypothalamus) GH Regulation: Inhibition GH secretion is inhibited by: Somatostatin / GHIH Hyperglycemia Increase in non-esterified fatty acids Insulin-like growth factor 1 (IGF-1) Directly inhibits somatotrophs Stimulates GHIH (which further inhibits somatotrophs) Somatostatin: Synthesized by many parts of the brain and organs (pancreas, stomach, others) Binds to (1) G - somatostatin receptor and promote tyrosine phosphatase activity (2) alpha i K channels resulting in hyperpolarized cell (stops release of GH) + GH Secretion Patterns In the adult, GH levels are reduced as a result of smaller pulse width and amplitude rather than a decrease in the number of pulses. GH Receptor: Class 1 Cytokine Receptor Family: Location: liver, bone, kidney, adipose tissue, muscle, brain, eye, heart and immune cells There are 2 bindings sites which allow the GH receptors to dimerize once GH binds Dimerization resulting in increased JAK activity which leads to phosphorylation of tyrosine residues These will allow the release of activators of transcription proteins, which will promote the expression of GH-regulated genes (genes that are influenced by growth hormone) GH Functions: Organ/ System Impact Bone Increase formation of new bone and cartilage resulting in longitudinal growth (peaks in puberty), in adults promotes bone turnover and bone formation to maintain healthy bones Adipose Tissue Lipolysis: release and oxidation of fatty acids by reducing lipoprotein lipase Skeletal Muscle Increases amino acid uptake, protein synthesis, cell proliferation Liver IGF-1 production and release, gluconeogenesis, reduce glucose uptake; promotes hepatic glucose output Immune System Influences B cells, antibody production, NK activity, macrophages and T cells CNS Influences mood and behavioural changes Metabolism Increase lipolysis, reduce skeletal muscle glucose utilization GH and IGF-1 IGF-1 (somatomedin) Regulated by GH, PTH and reproductive hormones (in bone) Function: stimulates bone formation, protein synthesis, glucose uptake into muscles, neuronal survival, myelin synthesis, bone turn over, collagen synthesis, linear growth, mitogen (DNA, RNA and protein synthesis) Low at birth, increases during childhood/puberty, begins to decline in 3rd decade IGF-2: function postnatally is unknown Too much GH – What would you expect? What symptoms or problems would you expect to see clinically given the functions of growth hormone, if it were to be produced in significant excess? Acromegaly Most often acromegaly is due to somatotrope adenoma resulting in over secretion of GH Bone: Acral bony overgrowth result in frontal bossing Increased hand and foot size Mandibular enlargement with prognathism Wide space between incisor teeth Soft tissue: Increased heel pad thickness, increased shoe size, coarse facial features, large fleshy nose Acromegaly – Neoplastic Complications GH – increase JAK/STAT pathway 🡪 proliferation BRCA1 – breast cancer Suppression of regulatory proteins 🡪 they typically stop inappropriate DNA and cell replication 🡪 colon and pituitary cancers Mitogen Acromegaly – Metabolic Complications Promote gluconeogenesis Reduction in insulin signaling pathway Insulin Resistance Acromegaly – Neurologic Complications If the somatotrope adenoma (aka AP tumour) grows large enough, it can: Impinge on the optic nerve (at the optic chiasm 🡪 bitemporal hemianopsia) left eye right eye Increase intracranial pressure (headaches, eventually impacting cortical function, selected cranial nerves) Acromegaly and Cardiovascular Impact Cardiomyopathy with arrhythmia’s Left ventricular hypertrophy Decreased diastolic function Hypertension Upper airway obstruction with sleep apnea (common) Central sleep dysfunction Soft tissue laryngeal airway obstruction Diabetes Gigantism If increased GH secretion occurs before epiphyseal long bone closure (in children or adolescents) this results in gigantism Gigantism has the same etiology and almost all the same clinical features/complicati ons as acromegaly However, GH secretion is elevated prior to Prolactin Synthesized by the lactotrophs (15-20% of anterior pituitary); amount of lactotrophs increases in response to estrogen (i.e. pregnancy) Secretion increases during sleep and reduces during wake hours Function: development of mammary glands and milk production Under tonic inhibition from the dopamine binding to D2 receptors on the lactotrophs; dopamine released from hypothalamus Somatostatin and GABA also exert inhibitory impact Stimulated by suckling and increased estrogen Suckling results in reduction of dopamine release from hypothalamus GnRH, serotonergic and opioidergic pathways also promote release as do Prolactin Releasing Factors (TRH, oxytocin, vasoactive intestinal peptide) Prolactin Function Prolactin Receptor: Location: mammary gland, ovary, brain Function: Develop mammary glands Milk synthesis Maintenance of milk synthesis Milk synthesis is prevented during pregnancy by high progesterone levels Inhibit GnRH What is the primary hormone responsible for stimulating both the synthesis and secretion of GH from somatotrophs? A. Somatostatin B. Insulin C. Ghrelin D. Growth hormone-releasing hormone (GHRH) What is the primary physiologic effect of growth hormone? A. Stimulation of brain function B. Suppression of immune response C. Promotion of adipocyte differentiation D. Stimulation of postnatal longitudinal growth Which family of receptors do growth hormone cell surface receptors belong to? A. G-protein couple receptors B. Class 1 cytokine receptors C. Tyrosine kinase receptors D. Steroid receptors What is the primary physiologic role of prolactin in the mammary gland? A. Inhibition of mammary gland development B. Suppression of milk synthesis C. Stimulation of milk production D. Regulation of lactose metabolism References The Hypothalamus and Posterior Pituitary Gland. In: Molina PE. eds. Endocrine Physiology, 5e. McGraw Hill; 2018. Accessed July 25, 2023. https://accessmedicine-mhmedical-com.ccnm.idm.oclc.org/conte nt.aspx?bookid=2343§ionid=183488081 Anterior Pituitary Gland. In: Molina PE. eds. Endocrine Physiology, 5e. McGraw Hill; 2018. Accessed July 25, 2023. https://accessmedicine-mhmedical-com.ccnm.idm.oclc.org/conte nt.aspx?bookid=2343§ionid=183488163 Melmed S, Jameson J. Pituitary Tumor Syndromes. In: Loscalzo J, Fauci A, Kasper D, Hauser S, Longo D, Jameson J. eds. Harrison's Principles of Internal Medicine, 21e. McGraw Hill; 2022. Accessed August 08, 2023. https://accessmedicine-mhmedical-com.ccnm.idm.oclc.org/content.aspx? bookid=3095§ionid=265439651 Adrenal Pathologies PAT-3.04 BMS 200 Learning outcomes Describe the pathophysiology of Cushing's syndrome and Cushing's disease and relate them to their respective clinical features. Describe the pathophysiology of Addison's disease and compare it to adrenal insufficiency. Describe the pathophysiology of Conn's disease and relate it to its clinical features. Predict the pathological findings, clinical features, and complications associated with pheochromocytoma. Explain the pathophysiology of adrenal neoplasms and their correlation with clinical features. Describe the pathophysiology of congenital adrenal hyperplasia and its relationship to clinical features. Relate the pathophysiology and clinical features of relevant endocrinologic disorders to the following diagnostic tests: insulin tolerance test, CRH test, aldosterone: renin ratio, 17-OH progesterone, serum ACTH, cortisol testing, DXM suppression test; neurologic imaging (MRI). Terminology Within many endocrine pathologies, the location of dysfunction is denoted by: Tertiary refers to dysfunction at the hypothalamus Secondary refers to dysfunction at the level of the pituitary gland Primary – refers to dysfunction within the “final” endocrine organ Eg. Adrenal glands Pathologies of the Adrenal Glands - intro Can involve hyper- or hypofunction: Hyperfunction: secondary causes are quite common Can involve increased levels of cortisol or aldosterone Disorders that are associated with elevated levels of androgens usually also involve decreased levels of glucocorticoids and/or mineralocorticoids Hypofunction: We will focus on significant insufficiencies of the adrenal cortex Subtle alterations of glucocorticoid levels will not be discussed today Pathologies covered today Hyperfunction: Hypofunction: Cushing’s syndrome Hypercortisolism ▪ Adrenocortical Hyperaldosteronism insufficiency Pheochromocytoma ▪ Congenital adrenal hyperplasia Cushing Syndrome Disorder caused by any condition that produces an elevation in glucocorticoid levels 4 common sources of excess cortisol: Iatrogenic hypothalamic-pituitary diseases associated with hypersecretion of ACTH Hypersecretion of cortisol by an adrenal adenoma, carcinoma or nodular hyperplasia Secretion of ectopic ACTH by a nonendocrine neoplasm – aka paraneoplastic syndrome Hypersecretion of ACTH 70 to 80% of cases of endogenous hypercorticolism Affects women 5 times more often than men, occurs most frequently in twenties and thirties Vast majority of cases: pituitary gland contains an ACTH- producing microadenoma Referred to as Cushing Disease What do you think the adrenal glands would look like? Why? Ectopic ACTH production Paraneoplastic syndrome Main tumours are small cell lung cancer and a renal adenocarcinoma Represent about 10% of cases of endogenous Cushing syndromes Usually involve rapid increases in the levels of ACTH and evolution of symptoms/signs What would you expect the adrenals to look like? ACTH-independent Cushing Syndrome Primary Cushing syndrome 10 to 20 % of cases of endogenous hypercorticolism are due to an adenoma in the adrenal glands themselves Adenomas are usually well-differentiated, more common in women, and functional (i.e. secrete cortisol) Assume the adenoma is only found in one adrenal gland – what would the rest of the adrenal tissue look like? Why? Adrenal Carcinoma Adrenocortical carcinoma Uncommon cause of primary Cushing’s syndrome FYI – Cushing’s disease No suppression => ectopic ACTH-dependent Labs - Cushing Syndrome continued For Cushing syndrome: Once Cushing syndrome is established, how could serum ACTH levels help us distinguish between primary Cushing’s disease or ACTH-dependent forms for Cushing’s syndrome? Cortisol ACTH Dexamethasone suppression test Cushing’s Disease High High Suppression Adrenal Adenoma High Low - Normal No suppression Paraneoplastic syndrome High High No suppression Adrenocortical insufficiency Can be primary or secondary What do these terms mean again? Can also be an acute or a chronic disorder Those with chronic adrenal insufficiency may show signs similar to acute insufficiency if their need for steroids (stress, infection) suddenly increases Acute can be iatrogenic – people being weaned off of high doses of glucocorticoids too quickly Why would this lead to adrenal insufficiency? Adrenal Insufficiency Caused by primary adrenal disease or decreased stimulation of the adrenals due to a deficiency of ACTH Patterns of adrenal insufficiency can be considered under the following headings: – Primary acute adrenocortical insufficiency (Adrenal crisis) – Primary chronic adrenal insufficiency (Addison Disease) – Secondary adrenocortical insufficiency Primary Acute Adrenocortical Insufficiency Etiologies: Inin steroid patients with chronic adrenocortical insufficiency precipitated by any form of stress that requires an increased output from glands incapable of responding In patients on exogenous corticosteroids Rapid withdrawal of steroids or failure to increase steroid doses in response to acute stress may precipitate an adrenal crisis = inability of atrophic adrenals to produce glucocorticoid hormones Adrenal hemorrhage Next slide Acute adrenal insufficiency: adrenal hemorrhage Adrenal glands among the best-perfused tissues in body Hemorrhage can be caused by a number of different problems: Newborns after a prolonged & difficult delivery Anticoagulant therapy Post-surgical disseminated intravascular coagulation (aka DIC) Waterhouse-Friderichsen syndrome (rare) Acute adrenal insufficiency Life-threatening Emergency Clinical features: Hemodynamic instability inability to maintain blood pressure, shock, severe postural hypotension Abdominal pain, nausea, vomiting, fever Hypoglycemia and hyponatremia Decreased level of consciousness, stupor Often exacerbated by concomitant illness and physiologic stress (shock, infection, surgery, trauma) Can occur on a background of slowly progressive chronic insufficiency Addison Disease: Chronic primary Adrenal Insufficiency Addison Disease Uncommon disorder resulting from progressive destruction of adrenal cortex Clinical manifestations do not appear until 90% of adrenal cortex compromised 90% of all cases are attributable to one of four disorders Autoimmune destruction of the adrenal cortex (60-70% in developed countries) TB (less common now, but most common cause worldwide) AIDS Sarcoidosis or malignancy usually lung or breast metastases Addison disease - pathogenesis Autoimmune causes: Autoimmune polyendocrine syndrome (APS) type 1 Rare, autosomal recessive condition FYI - mutation in an autoimmune regulator gene (AIRE) that is thought to help the immune system “ignore” normal tissue Begins in older children & adolescents several endocrine organs can be attacked APS type 2 More common than type I begins in early adulthood (20-40s) does not have skin or dental findings often autoimmune attack of multiple endocrine organs Idiopathic Addison Disease: Clinical Course Begins insidiously - at least 90% of cortex of both glands destroyed Initial manifestations: progressive weakness and easy fatigability GI disturbances: anorexia, nausea, vomiting, weight loss, diarrhea Hyperpigmentation Loss of potassium and sodium: Hypotension, hypokalemia, hyponatremia, volume depletion Stress (infection, trauma, or surgery) can cause an acute adrenal crisis Death can occur rapidly unless corticosteroid therapy begins immediately Secondary Adrenocortical Insufficiency Any disorder of hypothalamus and pituitary that reduces the output of ACTH → syndrome of hypoadrenalism Possible etiologies include: metastatic cancer, infection, infarction, irradiation With secondary disease, hyperpigmentation of primary Addison is absent Deficient cortisol and androgen output but normal aldosterone levels (therefore no hyperkalemia, hyponatremia) Labs: In the case of suspected ACTH deficiency: Insulin tolerance test: after insulin administration cortisol & ACTH secretion should increase CRH administration should also show increased cortisol and Labs – Adrenal insufficiency For Adrenal insufficiency Often ACTH & cortisol is measured (as 24-hour free urine cortisol and/or serum morning cortisol) What do we expect urine or serum cortisol & ACTH to be with Adrenal insufficiency? To distinguish between primary and secondary adrenal insufficiency an ACTH stimulation test can be done Cortisol ACTH ACTH stim test Exogenous ACTH (cosyntropin) is given Addison’s disease Low High Nothing 2ndary adrenal Low Low Things go to normal insufficiency Primary hyperaldosteronism Generic term for small group of closely related, uncommon syndromes Characterized by chronic excess aldosterone secretion Causes sodium retention and potassium excretion Resulting in hypertension and hypokalemia Indicates an autonomous overproduction of aldosterone, with resultant suppression of the renin-angiotensin system and decreased plasma renin activity Primary Hyperaldosteronism Where is the level of dysfunction? Etiologies: Adrenocortical neoplasm: usually a solitary aldosterone secreting adenoma Conn Syndrome Bilateral hyperplasia of adrenals Secondary hyperaldosteronism Aldosterone release in response to activation of the renin- angiotensin system Characterized by increased levels of plasma renin Conditions: – Decreased renal perfusion – Arterial hypovolemia and edema: CHF, cirrhosis, nephrotic syndrome – Pregnancy: due to estrogen induced increases in plasma renin substrate Clinical course of Hyperaldosteronsim Hypertension: sodium retention increases total body sodium and expands the extracellular fluid Hypokalemia: due to renal potassium wasting and can cause variety of neuromuscular manifestations Labs: Serum aldosterone & renin can be measured for an aldosterone: renin ratio (ARR) What would you expect serum aldosterone to be? What about the ARR? Congenital Adrenal Hyperplasia (CAH) Autosomal recessive defect in enzyme in cortisol synthesis pathways Most common enzyme deficiency: 21- Hydroxylase Results in mild to complete lack of cortisol production How would this result in adrenal hyperplasia? What would accumulate? Congenital Adrenal Hyperplasia (CAH) continued Types: Classic: affects newborns Salt-wasting: complete inactivation of 21- hydroxylase Simple virilizing: significantly reduced function of 21-hydroxylase Non-classic Milder & later onset (late childhood/early adulthood) Partial deficiency in 21-hydroxylase Congenital Adrenal Hyperplasia (CAH) – classic forms Salt-wasting No synthesis of aldosterone causes hyponatremia, hyperkalemia, dehydration, hypotension, & increased renin secretion Can be fatal if not treated Virilization of female infants Simple virilizing Female infants often have ambiguous genitalia at birth Male infants show no abnormalities of sexual organs at birth Adult women typically experience infertility Adult men can be fertile or experience azoospermia Congenital Adrenal Hyperplasia (CAH) – non-classic Late-onset (aka non-classic) Most common form of CAH No abnormalities at birth, symptoms typically present at puberty In women, late-onset CAH can often mimics poly cystic ovary syndrome (PCOS) Hirsutism, acne, menstrual irregularities, infertility Men are often asymptomatic Labs 17 hydroxyprogesterone (17-OHP) levels as assessed, what would you expect to see? Pheochromocytoma Rare tumour of chromaffin cells Most of the time secretes elevated quantities of catecholamines Etiology: Most often sporadic, occasionally inherited alone or as part of hereditary syndrome Pathology: Most of the time tumours arises in the adrenal medulla FYI - (10% will arise elsewhere, i.e. carotid bodies) In about 10% of cases they can be malignant and will invade Pheochromocytoma continued Clinical features: Dominant picture is hypertension Many have a baseline elevated blood pressure 2/3 will experience paroxysmal hypertension enormous acute increases in blood pressure, often precipitated by stress or even posture changes Large changes in blood pressure can result in cerebrovascular accidents, heart failure, and eventual hypertrophy of the heart Treated with surgical excision – surgically curable high blood pressure Elevated levels of what would you expect to find in the urine? Adrenals Labs Summary Increased levels of cortisol: Cushing disease, Cushing syndrome Stress (physiologic or psychologic) Hyperthyroidism (increased cortisol to meet needs of metabolism) Decreased levels of cortisol: Congenital adrenal hyperplasia Addison disease Hypopituitarism, hypothyroidism Adrenal Physiology PHY-3.02 BMS 200 Outcomes for today ∙ Contrast the histology, regulation, and secretions of the different zones of the adrenal glands. ∙ Explain the synthesis of steroid hormones, highlighting significant enzymes involved, as well as their transport and metabolism. ∙ Discuss the physiological roles of glucocorticoids, including their impact on carbohydrate, protein, and lipid metabolism, immune system modulation, and responsiveness to the autonomic nervous system and vasopressors. ∙ Explain the basic role of mineralocorticoids in sodium handling and fluid homeostasis. Outline General anatomy of the adrenal glands ▪ Microscopic & macroscopic anatomy ▪ Vasculature ▪ Embryology Glucocorticoid: ▪ Synthesis & regulation ▪ Effects Mineralocorticoid ▪ Synthesis & regulation ▪ Effects Catabolism of glucocorticoids & mineralocorticoids Catecholamines ▪ Synthesis & regulation ▪ Effects ▪ catabolism General Roles of the Adrenal Glands Key components of the endocrine system that are essential to life Four major sets of hormones: ▪ Glucocorticoids regulation of blood sugar and physiologic response to stress ▪ Mineralocorticoids maintain extracellular fluid (ECF) volume, sodium and potassium balance ▪ Weak androgens role in adults is yet to be fully elucidated ▪ Catecholamines: mostly epinephrine with some norepinephrine Adrenal Glands – General Anatomy ~ 3-5cm long, weighs 1.5 – 2.5g 2 components: ▪ cortex - Steroid Hormones Adrenal ▪ medulla - Catecholamines gland Major vasculature: ▪ Suprarenal artery and vein Supplied by abdominal aorta Extremely well-perfused, perhaps the most “vascular” organ in the body In adults, the kidneys and the adrenal glands are surrounded by a large fat pad Image adapted from: Lippincott® Atlas of Anatomy, Chapter 5, Adrenal Glands – General Anatomy The adrenal glands, located on top of each kidney, have a rich blood supply. Each gland gets blood from three main sources: 1. Superior suprarenal arteries - These come from the lower part of the diaphragm (the muscle that helps with breathing). 2. Middle suprarenal artery - This comes directly from the abdominal aorta (the main artery that supplies blood to the lower half of the body). 3. Inferior suprarenal arteries - These come from the renal arteries, which also supply the kidneys. Once blood flows into the adrenal gland, it moves through small vessels inside and then leaves through a single central vein. On the right adrenal gland, this vein drains into the inferior vena cava, while on the left side, it drains into the renal vein. Adrenal Glands – General Anatomy continued Image adapted from: Moore’s Clinically Oriented Anatomy, Chapter Adrenal Glands - Embryology Adrenal Medulla is Derived from Neural Crest Cells The adrenal medulla, the inner part of the adrenal gland, originates from neural crest cells during embryonic development. Neural crest cells are a group of cells in the embryo that give rise to various structures in the body, including the peripheral nervous system and parts of the adrenal gland. Neural crest cells differentiate into many types of cells, including those that become the chromaffin cells of the adrenal medulla. These chromaffin cells are responsible for producing and releasing catecholamines, such as adrenaline (epinephrine) and noradrenaline (norepinephrine). Because the medulla originates from the neural crest, its function is closely related to the sympathetic nervous system, which also arises from the neural crest. This connection explains why the adrenal medulla acts like a large sympathetic ganglion. Adrenal Glands - Embryology 2. The Medulla Functions as an “Overgrown Sympathetic Ganglion” The adrenal medulla is often described as an "overgrown sympathetic ganglion" because of its relationship with the sympathetic nervous system (SNS), which is responsible for the body's rapid response to stress. In the SNS, sympathetic ganglia are clusters of nerve cells that relay signals from the brain to various organs. The adrenal medulla functions in a similar way but on a much larger scale. Instead of sending signals through nerve fibres to organs, the adrenal medulla directly releases hormones (epinephrine and norepinephrine) into the bloodstream. When the brain detects stress, it activates the adrenal medulla through signals from sympathetic nerves, causing it to release these hormones quickly. This leads to a system-wide response, increasing heart rate, blood pressure, and energy availability, just like the sympathetic nervous system does, but through chemical signals instead of nerve impulses. Adrenal Glands - Embryology Adrenal Cortex is Derived from Mesoderm The outer layer of the adrenal gland, called the adrenal cortex, develops from the mesoderm, one of the three primary germ layers in the embryo. The mesoderm is responsible for forming many structures in the body, including muscles, bones, and the cardiovascular system. The adrenal cortex produces steroid hormones, which are essential for various physiological functions: The zona glomerulosa produces aldosterone, a hormone that regulates blood pressure by controlling sodium and water balance. The zona fasciculata produces cortisol, which helps regulate metabolism, immune responses, and the body's stress response. The zona reticularis produces androgens, which are precursor hormones for sex steroids like testosterone and estrogen. Adrenal Glands - Embryology Since the adrenal cortex arises from the mesoderm, it has a different origin and function compared to the adrenal medulla. The medulla is more closely tied to the nervous system, while the cortex deals with hormonal regulation of various body systems, especially in response to longer-term stress and metabolic demands. Adrenal Glands - Embryology Embryology: Adrenal medulla is derived from neural crest cells ▪ The medulla functions as an “overgrown sympathetic ganglion” Adrenal cortex is derived from mesoderm Adrenal Glands – Functional Histology & Anatomy Four major zones: Cortex: 1. Zona glomerulosa ▪ Smallest zone ▪ Mineralocorticoid secretion 2. Zona fasciculata ▪ Largest zone ▪ Glucocorticoid secretion 3. Zona reticularis ▪ Androgen secretion Adrenal Glands – Functional Histology & Anatomy Four major zones continued: 4. Medulla: Catecholamines ▪ Epinephrine secreted in large quantities (when secretion is stimulated) BMS 100 review of HPA The hypothalamic-pituitary axis (HPA) Hormones released by the hypothalamus regulate hormone release from the anterior pituitary Anterior pituitary hormones regulate the activity of a range of target endocrine glands BMS 100 review of HPA Hypothalamus Releases hormones (like CRH, TRH, GnRH). These hormones travel to the —-> Anterior Pituitary Stimulate the release of pituitary hormones (like ACTH, TSH, LH, FSH). Anterior pituitary hormones then act on target endocrine glands (adrenal cortex, thyroid, gonads) to regulate the production of further hormones (like cortisol, thyroid hormones, sex hormones). Feedback mechanisms ensure that hormone levels remain balanced to avoid over- or underproduction. This system is critical for managing responses to stress, regulating metabolism, controlling reproductive functions, and maintaining overall homeostasis. BMS 100 review of HPA Hormones Released by the Hypothalamus Corticotropin-releasing hormone (CRH): Stimulates the release of adrenocorticotropic hormone (ACTH) from the anterior pituitary. Thyrotropin-releasing hormone (TRH): Stimulates the release of thyroid- stimulating hormone (TSH). Gonadotropin-releasing hormone (GnRH): Stimulates the release of luteinizing hormone (LH) and follicle-stimulating hormone (FSH). Growth hormone-releasing hormone (GHRH): Stimulates the release of growth hormone (GH). Somatostatin (GHIH): Inhibits the release of GH and thyroid- stimulating hormone (TSH). Dopamine (prolactin-inhibiting factor): Inhibits the release of prolactin. BMS 100 review of HPA Hormones Released by the Anterior Pituitary Gland Adrenocorticotropic hormone (ACTH): Stimulates the adrenal cortex to produce cortisol, a key hormone in the stress response and metabolism regulation. Thyroid-stimulating hormone (TSH): Stimulates the thyroid gland to produce thyroid hormones (T3 and T4), which regulate metabolism and energy levels. Luteinizing hormone (LH) and follicle-stimulating hormone (FSH) : Target the gonads (testes in males and ovaries in females), regulating reproductive functions and the production of sex hormones (testosterone, estrogen, and progesterone). Growth hormone (GH): Stimulates growth, cell reproduction, and regeneration, targeting bones and muscles primarily. Prolactin: Promotes milk production in the mammary glands. Basic regulation of adrenal hormone secretion Adrenal gland hormone secretion depends on the zone: ▪ Cortisol release is regulated by ACTH from the anterior pituitary gland ▪ Mineralocorticoid release is regulated by: Secretion of angiotensin II Serum K+ levels ▪ Epinephrine release is regulated by the sympathetic nervous system It’s basically a huge sympathetic ganglion FYI – regulation of adrenal androgen secretion is still under investigation Basic regulation of adrenal hormone secretion continued In the case of steroid hormones, secretion is regulated through enzymatic activation alone ▪ The type of steroid hormone that is produced depends on the enzymes expressed by the cell ▪ We don’t need exocytosis for secretion of steroid hormones Why? Steroid hormones are hydrophobic and can cross the cell membrane via simple diffusion In the case of catecholamines, secretion is regulated both by: ▪ a) Enzymatic activation ▪ b) Exocytosis of vesicles containing epinephrine and norepinephrine What does the last slide actually mean? Steroid hormones (like cortisol, aldosterone, and sex hormones such as estrogen and testosterone) are derived from cholesterol. These hormones are not stored in the cells that produce them. Instead, they are made "on demand" when the body needs them. Steroid hormones are lipid-soluble, so they can easily pass through cell membranes, making storage in vesicles difficult. Enzymatic Activation for Hormone Production The production of steroid hormones relies on a series of enzymatic reactions that convert CHOLESTEROL into different hormones. The rate of hormone secretion is controlled by: how active these enzymes are When the body signals a need for more of a specific steroid hormone (e.g., cortisol during stress), the enzymes responsible for synthesizing that hormone become more active, increasing production. Once synthesized, the hormone diffuses out of the cell into the bloodstream, as it cannot be stored. Type of Steroid Hormone Depends on the Enzymes in the Cell Different types of cells express different enzymes, and these enzymes determine which steroid hormones the cell can produce. In the adrenal cortex the zona glomerulosa expresses enzymes that convert cholesterol into aldosterone(which regulates salt and water balance). In the zona fasciculata, other enzymes are expressed that lead to the production of cortisol (which helps regulate metabolism and stress responses). In the gonads (testes or ovaries), enzymes convert cholesterol into testosterone or estrogen (which regulate reproductive functions). Regulation of glucocorticoid secretion - overview Corticotrophin releasing hormone (CRH) is released from hypothalamus CRH stimulates ACTH release from anterior pituitary gland ACTH acts on the adrenal gland to increased synthesis of cortisol in the zona fasciculata. Let’s take a closer look at this step on the next slide Regulation of glucocorticoid secretion ACTH binds to a G-Protein coupled receptor on zona fasciculata cells When ACTH binds it activates a G- protein inside the cell. The G-protein consists of three subunits (α, β, γ), but the most important one here is the α-subunit (Gs) When activated, the α-subunit dissociates and activates an enzyme called adenylyl cyclase. Results in increased cAMP and Regulation of glucocorticoid secretion The increased levels of cAMP activate another enzyme called protein kinase A (PKA). PKA is an important regulatory enzyme that phosphorylates (adds a phosphate group to) various target proteins inside the cell. Phosphorylation changes the activity of these proteins, leading to specific cellular responses. Synthesis of adrenal steroids - 1 First off, we need a store of cholesterol: ▪ Most cholesterol used for steroid hormone synthesis comes from LDL uptake (exogenous pathway) rather than intracellular synthesis of cholesterol ▪ Exogenous pathway: cholesterol esters are taken up from LDL/IDL via upregulation of the LDL receptor ▪ Cholesteryl esters are stored in a lipid droplet if not needed Cholesteryl ester hydrolase (CEH) removes the fatty acid Cholesterol is released and can be used for steroid hormone synthesis Synthesis of adrenal steroids - 2 Cholesterol mobilization: PKA stimulates the release of cholesterol from intracellular stores (such as lipid droplets). Cholesterol is transported into the mitochondria, the site where the first steps of steroid hormone production take place. Steroidogenic acute regulatory protein (StAR): PKA enhances the activity of StAR, a crucial protein that helps transport cholesterol into the inner mitochondrial membrane. Synthesis of adrenal steroids - 3 All steroid hormone synthesis begins at the inner membrane of the mitochondria with cleavage of the cholesterol side chain and formation of pregnenolone Enzyme cascade: In the mitochondria, a series of enzymes (such as P450scc, 3β-HSD, 11β-hydroxylase, etc.) convert cholesterol into cortisol through several intermediate steps. The first step involves converting cholesterol into pregnenolone, and through subsequent reactions, it is finally transformed into cortisol. ▪ Enzyme: side-chain cleavage enzyme (SCC) This is the rate limiting step of the synthesis pathway Synthesis of glucocorticoids The remaining steps of glucocorticoid synthesis occur in the inner mitochondrial membrane and smooth endoplasmic reticulum Pregnenolone is eventually converted into cortisol or corticosterone ▪ Cortisol is the more potent glucocorticoid and its production is higher! Glucocorticoid synthesis regulation What were the Let’s consider how ACTH upregulates the synthesis of roles of the glucocorticoids in the zona fasciculata SCC enzymes ACTH upregulates the following steps: again? steroidogene ▪ Increased LDL receptor expression sis, which is ▪ Increased activity of CEH and StAR the process by ▪ Increased activity of the side chain cleavage which enzymes cholesterol is ACTH also is trophic for the adrenal gland – it makes it converted into grow steroid hormones like cortisol, aldosterone, Regulation of glucocorticoid synthesis FIGURE 9–6 Fluctuations in plasma ACTH and glucocorticoids (11- OHCS) throughout the day. Note the greater ACTH and glucocorticoid rises in the morning Regulation of glucocorticoid synthesis How are CRH and ACTH release regulated? ▪ Circadian rhythm – we have a “central clock” located in the suprachiasmatic nucleus of the hypothalamus (SCN) that is “calibrated” by melatonin secretion in response to darkness Contributes to regulation of CRH release ▪ Stress – CRH and ACTH are secreted in response to a wide range of (significant) stressors including: Surgery, hypoglycemia, inflammatory cytokines (physiologic stressors) Pain, unpleasant mood (psychologic stressors) ▪ Negative feedback Cortisol negatively feeds back to limit ACTH as well as CRH secretion Effects of glucocorticoids - intracellular Glucocorticoid receptors are found within the cytosol in almost all tissues in a wide range of cells ▪ Cortisol binds to the glucocorticoid receptor ▪ Stimulate transcription at the hormone responsive element (HRE) ▪ Review from BMS100: Binding of cortisol displaces the heat shock protein (HSP) —> Receptor & hormone then form a dimer and translocate the the nucleus —> stimulate transcription at a HRE General effects of glucocorticoids on metabolism and fighting stress Increase energy availability by: ▪ General inhibition of DNA/protein synthesis and acceleration of protein catabolism ▪ Increasing hepatic gluconeogenesis by: Stimulating gluconeogenesis enzymes (PEPCK and G-6-phosphatase) Increased hepatic responsiveness to glucagon ▪ Increasing hepatic & adipose lipolysis More to be available for glycerol for gluconeogenesis Increased free fatty acid release ▪ Can be broken down through which pathway for energy? A: free fatty acids can be broken down in Beta-oxidation ▪ Decreasing glucose uptake in muscle and adipose tissue Chronically this will contribute to increased insulin secretion ▪ Increasing appetite General effects of glucocorticoids on other tissues Fetus ▪ Combinations of glucocorticoids and a wide variety of other hormones are important in the fetal development of the lung and the liver Bone ▪ Physiologic levels of glucocorticoids do not impair bone, but excess glucocorticoids can inhibit bone formation through multiple different pathways: Osteoblast inhibition & overactivation of osteoclasts Potentiation of the effects of parathyroid hormone, reduction of calcium absorption Healing tissue ▪ Physiologic concentration of glucocorticoids can be helpful in healing tissue, but excess glucocorticoids inhibit fibroblasts and impair healing Preview – causing thinned skin and increased bruising General effects of glucocorticoids on other tissues continued Increase effectiveness of catecholamines at peripheral vessels and the heart, and “hypertension-inducing” effects at the kidney ▪ Increasing cardiac output, increasing blood pressure ▪ Also seem to increase salt and water retention at high doses, independently of mineralocorticoids ▪ May also increase the activity of angiotensin II Central nervous system ▪ Physiologic roles not fully understood ▪ Supraphysiologic levels result in euphoria initially and then later an array of mood disturbances: Irritability & emotional lability Depression Rarely psychosis ▪ Low levels invariably result in fatigue and depression General effects of glucocorticoids – the immune system Physiologic, short-term elevations in glucocorticoids increase neutrophil number and activity Physiologic, short-term elevations in glucocorticoids increase the diapedesis of: ▪ Monocytes, eosinophils ▪ Lymphocytes ▪ May also increase the function of innate immune cells, but this is difficult to prove Long-term elevations or supra-physiologic elevations of cortisol result in: ▪ Impaired macrophage activity and healing ▪ Impaired lymphocyte production and antibody production ▪ Decreased migration of cells to damaged sites ▪ Inhibit phospholipase A2 (this decreasing prostaglandin production) General effects of glucocorticoids on other tissues continued Massive number of distributed effects – see FYI table at this link (need to be logged into the library): ▪ https://accessmedicine-mhmedical-com.ccnm. idm.oclc.org/ViewLarge.aspx?figid=16624935 5&gbosContainerID=0&gbosid=0&groupID=0 §ionId=166249274 Synthesis of mineralocorticoids Initial steps are the same as for glucocorticoids ▪ Cholesterol is converted to pregnenolone Enzyme? ▪ Through various steps pregnenolone is converted to corticosterone Corticosterone is converted to aldosterone ▪ Production of aldosterone depends on the presence of aldosterone synthase located on the inner mitochondrial membrane only expressed by cells of the zona glomerulosa Mineralocorticoid secretion regulation Mineralocorticoids are not primarily regulated by ACTH ▪ Although, ACTH can increase the secretion of aldosterone Major regulators of aldosterone secretion are: ▪ Angiotensin II within the renin-angiotensin-aldosterone system (RAAS) ▪ Elevations in serum K+ Signaling in the RAAS – the basics Renin angiotensin aldosterone system ▪ Decreased perfusion to the kidney stimulates renin release -- > renin catalyzes the conversion of Angiotensin to Angiotensin I More detail to come in BMS Signaling in the RAAS – the basics ▪ Angiotensin II has multiple effects: ▪ Vasoconstriction ▪ Stimulates secretion of aldosterone ▪ Stimulates secretion of ADH/AVP from posterior pituitary gland ▪ Increases reabsorption of Na+ and secretion of K+ in the kidneys More detail to come in BMS Aldosterone - effects The major effects of aldosterone are: ▪ Increased sodium reabsorption and increased potassium secretion from the kidney Sodium reabsorption causes increase water retention and overall increased ECF volume ▪ Decreased potassium reabsorption from the GI tract ▪ Increased activity of the sodium/potassium pump in many cells Helps to decrease K+ concentrations in the serum to avoid severe electrolyte imbalances (hyperkalemia) Much more limited than the effects of glucocorticoids ▪ Glucocorticoids can also bind to the aldosterone receptor (with low affinity) and also have some “aldosterone-like” effects How are these hydrophobic steroid hormones carried in the circulation? Cortisol and aldosterone will bind somewhat to albumin The majority of cortisol is carried by cortisol binding protein (CBG) Aldosterone circulates in a primarily unbound state, and its clearance is much more rapid than that of cortisol How are adrenal steroids metabolized? Both cortisol & aldosterone need to be glucuronidated by the liver before being excreted by the kidneys ▪ How does glucuronidation help with excretion? ▪ A: glucuronidation makes the hydrophobic steroid hormones more polar & therefore more easily excreted by the kidney ▪ Metabolites can be measured in the urine Eliminated forms of cortisol are known as 17-hydroxycorticosteroids Aldosterone as 18-glucuronide Synthesis of catecholamines Catecholamines are synthesized from the amino acids tyrosine. Adrenal medulla is the only tissue that produces epinephrine ▪ how does epinephrine differ from norepinephrine pharmacologically? ▪ Epi stimulates both alpha & Beta receptors. ▪ NE stimulates alpha 1 & 2, Beta 1 but has very little effect on B2 Regulation of catecholamine synthesis Sympathetic stimulation, ACTH, and cortisol all stimulate the synthesis of catecholamines Catecholamine synthesis Exocytosis of NE & Sympathet E ic stimulation ▪ Sympatheti c stimulation triggers exocytosis granules containing E & NE Catecholamine synthesis Stress Response and ACTH-Cortisol Pathway (Chronic Regulation): Sympathet Stress activates the hypothalamus, which ic releases corticotropin-releasing hormone (CRH). stimulation CRH stimulates the anterior pituitary to release adrenocorticotropic hormone (ACTH). ACTH targets the adrenal cortex to increase the production of cortisol. Cortisol, produced in the adrenal cortex, reaches the adrenal medulla via the intra- adrenal portal system. Cortisol induces the enzyme phenylethanolamine-N-methyltransferase (PNMT), which converts norepinephrine (NE) into epinephrine (Epi). Chronic regulation refers to how long-term stress or sustained ACTH and cortisol levels affect catecholamine production over time. Catecholamine synthesis Acute Regulation (Neuron Signaling): Sympathet Neurons stimulate the adrenal medulla ic through the release of acetylcholine. stimulation Acetylcholine binds to receptors on chromaffin cells (the cells that produce catecholamines), causing an influx of calcium (Ca²⁺) into the cell. Calcium promotes the exocytosis (release) of catecholamines stored in neurosecretory granules. This mechanism is acute regulation, as it responds to immediate stress or neuronal stimuli, rapidly increasing the release of norepinephrine and epinephrine into the bloodstream. Catecholamine synthesis Catecholamine Biosynthesis Pathway (Inside Chromaffin Cells): Sympathet The process begins with tyrosine, an ic amino acid that undergoes several enzyme-driven reactions to produce stimulation catecholamines: Tyrosine Hydroxylase (TH): Converts tyrosine to L-DOPA. DOPA Decarboxylase (DD): Converts L- DOPA to dopamine (DPN). Dopamine β-hydroxylase (DH): Converts dopamine into norepinephrine (NE). In the presence of cortisol, PNMT converts norepinephrine into epinephrine (Epi). Catecholamines are then stored in neurosecretory granules until they are released in response to neuronal signals. Actions of catecholamines - review Heart ▪ Increased contractile force ▪ Increased heart rate Vessels ▪ Vasoconstriction in skin, visceral tissue ▪ Limited vasoconstriction or vasodilation in skeletal muscle, cardiac muscle Energy metabolism ▪ ↑ blood glucose and “circulating” energy stores Gluconeogenesis, glycogenolysis, ketogenesis in the liver Lipolysis in adipose tissue Lungs ▪ Bronchodilation, decreased mucous production Catabolism of catecholamines The process by which the body breaks down catecholamines after they have performed their functions. Epinephrine and norepinephrine are excreted in the urine in the form of metanephrine and vanillylmandelic acid (VMA) Catabolism of catecholamines Key Steps in Catecholamine Breakdown: Enzymes Involved: Monoamine oxidase (MAO): An enzyme that breaks down catecholamines by removing an amine group. Catechol-O-methyltransferase (COMT): Another enzyme that helps by adding a methyl group to catecholamines, making them easier to break down. Main Pathway: Epinephrine and norepinephrine are first degraded by COMT, producing an intermediate called metanephrine (from epinephrine) or **normetanephrine** (from norepinephrine). Then, MAO breaks down these intermediates into vanillylmandelic acid (VMA), which is a final breakdown product. VMA is excreted in the urine. Catabolism of catecholamines Locations of Breakdown: Catecholamines are broken down primarily in the liver, kidneys, and nerve endings. This process ensures that catecholamines do not remain in the body too long after their effects are no longer needed (like after stress or a fight-or-flight response). Catecholamines like epinephrine and norepinephrine are broken down by the enzymes **MAO** and COMT into byproducts like VMA, which is then excreted in the urine. This prevents overstimulation and keeps hormone levels balanced.