Wireless Power Transfer PDF
Document Details
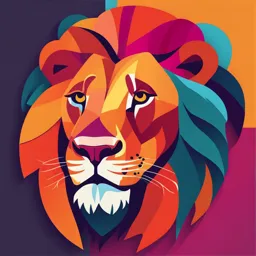
Uploaded by FortunateDystopia
Tags
Summary
This project details the design and implementation of a wireless charger for low power devices utilizing resonant inductive coupling. The document explores the fundamental principles of wireless power transfer, and potential applications.
Full Transcript
WIRELESS POWER TRANSFER INDEX Title Page no. 1. Abstract 01 2. Introduction 02 3. The basic concept of wireless power transf...
WIRELESS POWER TRANSFER INDEX Title Page no. 1. Abstract 01 2. Introduction 02 3. The basic concept of wireless power transfer 03 4. The inductance of Coil and Coil Design 05 5. Block diagram 07 6. Circuit diagram 08 7. Transmitter 10 8. Receiver 12 9. PCB layout 14 10. Output 15 11. Conclusion 16 12. Application and future work 17 13. Advantages and disadvantage 19 14. Biography 20 15. Appendix 21 INTRODUCTION We live in a world of technological advancement. New technologies emerge each and every day to make our life simpler. Despite all these, we still rely on the classical and conventional wire system to charge our everyday use low power devices such as mobile phones, digital camera etc. and even mid power devices such as laptops. The conventional wire system creates a mess when it comes to charging several devices simultaneously. It also takes up a lot of electric sockets and not to mention the fact that each device has its own design for the charging port. At this point a question might arise. ―What if a single device can be used to charge these devices simultaneously without the use of wires and not creating a mess in the process? We gave it a thought and came up with an idea. The solution to all these dilemma lies with inductive coupling, a simple and effective way of transferring power wirelessly. Wireless Power Transmission (WPT) is the efficient transmission of electric power from one point to another trough vacuum or an atmosphere without the use of wire or any other substance. This can be used for applications where either an instantaneous amount or a continuous delivery of energy is needed, but where conventional wires are unaffordable, inconvenient, expensive, hazardous, unwanted or impossible. The power can be transmitted using Inductive coupling for short range, Resonant Induction for mid-range and Electromagnetic wave power transfer for high range. WPT is a technology that can transport power to locations, which are otherwise not possible or impractical to reach. Charging low power devices and eventually mid power devices by means of inductive coupling could be the next big thing. The objective of this project is to design and construct a method to transmit wireless electrical power through space and charge a designated low power device. The system will work by using resonant coils to transmit power from an AC line to a resistive load. Investigation of various geometrical and physical form factors evaluated in order to increase coupling between transmitter and receiver. Success in doing so would eliminate the use of cables in the charging process thus making it simpler and easier to charge a low power device. It would also ensure the safety of the device since it would eliminate the risk of short circuit. The objective also includes the prospect of charging multiple low power devices simultaneously using a single source that would use a single power outlet. Basic concept of wireless power transfer 1) Inductive Coupling Inductive or Magnetic coupling works on the principle of electromagnetism. When a wire is proximity to a magnetic field, it generates a magnetic field in that wire. Transferring energy between wires through magnetic fields is inductive coupling. If a portion of the magnetic flux established by one circuit interlinks with the second circuit, then two circuits are coupled magnetically and the energy may be transferred from one circuit to the another circuit. This energy transfer is performed by the transfer of the magnetic field which is common to the both circuits. In electrical engineering, two conductors are referred to as mutual-inductively coupled or magnetically coupled when they are configured such that change in current flow through one wire induces a voltage across the end of the other wire through electromagnetic induction. The amount of inductive coupling between two conductors is measured by their mutual inductance. R1 R 2 φ12 I1 Φ E1 φ11 E2 φ22 Circuit-1 Circuit-2 Inductive Coupling with Four Component Fluxes Power transfer efficiency of inductive coupling can be increased by increasing the number of turns in the coil, the strength of the current, the area of cross-section of the coil and the strength of the radial magnetic field. Magnetic fields decay quickly, making inductive coupling effective at a very short range. 2) Inductive Charging Inductive charging uses the electromagnetic field to transfer energy between two objects. A charging station sends energy through inductive coupling to an electrical device, which stores the energy in the batteries. Because there is a small gap between the two coils, inductive charging is one kind of short-distance wireless energy transfer. Induction chargers typically use an induction coil to create an alternating electromagnetic field from within a charging base station, and a second induction coil in the portable device takes power from the electromagnetic field and converts it back into electrical current to charge the battery. The two induction coils in proximity combine to form an electrical transformer. Greater distances can be achieved when the inductive charging system uses resonant inductive coupling. The inductance of Coil and Coil Design Introduction An ideal inductor has inductance, but no resistance or capacitance, and does not dissipate or radiate energy. However, real inductors have resistance (due to the resistance of the wire and losses in core material), and parasitic capacitance (due to the electric field between the turns of wire which are at slightly different potentials). At high frequencies the capacitance begins to affect the inductor's behavior; at some frequency, real inductors behave as resonant circuits, becoming self-resonant. At frequencies above this the capacitive reactance becomes the dominant part of the impedance. Energy is dissipated by the resistance of the wire, and by any losses in the magnetic core due to hysteresis. At high currents, iron core inductors also show gradual departure from ideal behavior due to nonlinearity caused by magnetic saturation. At higher frequencies, resistance and resistive losses in inductors grow due to skin effect in the inductor's winding wires. Core losses also contribute to inductor losses at higher frequencies Single Layer Coil Block Diagram Circuit Diagram Components used in transmitter: Component’s Name Component’s Value or code Voltage Source, Vdc 15V Capacitor, C 10nF Resistor, R1 39 ohm, 5watt Resistor, R2 39 ohm, 5watt Resistor, R3 39 ohm, 5watt Resistor, R4 39 ohm, 5watt Resistor, R5 5.6k ohm Resistor, R6 5.6k ohm Diode, D1 1N4148 Diode, D2 1N4148 MOSFET,Q1 IRF540 MOSFET, Q2 IRF540 Radio Frequency Choke,L1 120 µH Radio Frequency Choke, L2 120 µH Transmitter coil, L 8 µH Components used in receiver: Component’s Name Component’s Value or code Diode, D1 OA79 Diode, D2 OA79 Diode, D3 OA79 Diode, D4 OA79 Capacitor, C1 10 Nf Capacitor, C2 100 µF Voltage Regulator IC IC LM 7812 Receiver coil, L 8 µH COMPONENTS SPECIFICATION 1) capacitor capacitor, device for storing electrical energy, consisting of two conductors in close proximity and insulated from each other. A simple example of such a storage device is the parallel-plate capacitor. If positive charges with total charge +Q are deposited on one of the conductors and an equal amount of negative charge −Q is deposited on the second conductor, the capacitor is said to have a charge Q. (See also electricity: Principle of the capacitor.) Capacitors have many important applications. They are used, for example, in digital circuits so that information stored in large computer memories is not lost during a momentary electric power failure; the electric energy stored in such capacitors maintains the information during the temporary loss of power. Capacitors play an even more important role as filters to divert spurious electric signals and thereby prevent damage to sensitive components and circuits caused by electric surges. There are two types of electrical charge, a positive charge in the form of Protons and a negative charge in the form of Electrons. When a DC voltage is placed across a capacitor, the positive (+ve) charge quickly accumulates on one plate while a corresponding and opposite negative (-ve) charge accumulates on the other plate. For every particle of +ve charge that arrives at one plate a charge of the same sign will depart from the -ve plate. Then the plates remain charge neutral and a potential difference due to this charge is established between the two plates. Once the capacitor reaches its steady state condition an electrical current is unable to flow through the capacitor itself and around the circuit due to the insulating properties of the dielectric used to separate the plates. The flow of electrons onto the plates is known as the capacitors Charging Current which continues to flow until the voltage across both plates (and hence the capacitor) is equal to the applied voltage Vc. At this point the capacitor is said to be “fully charged” with electrons. The strength or rate of this charging current is at its maximum value when the plates are fully discharged (initial condition) and slowly reduces in value to zero as the plates charge up to a potential difference across the capacitors plates equal to the source voltage. The amount of potential difference present across the capacitor depends upon how much charge was deposited onto the plates by the work being done by the source voltage and also by how much capacitance the capacitor has and this is illustrated below. The parallel plate capacitor is the simplest form of capacitor. It can be constructed using two metal or metallised foil plates at a distance parallel to each other, with its capacitance value in Farads, being fixed by the surface area of the conductive plates and the distance of separation between them. Altering any two of these values alters the value of its capacitance and this forms the basis of operation of the variable capacitors. Also, because capacitors store the energy of the electrons in the form of an electrical charge on the plates the larger the plates and/or smaller their separation the greater will be the charge that the capacitor holds for any given voltage across its plates. In other words, larger plates, smaller distance, more capacitance. By applying a voltage to a capacitor and measuring the charge on the plates, the ratio of the charge Q to the voltage V will give the capacitance value of the capacitor and is therefore given as: C = Q/V this equation can also be rearranged to give the familiar formula for the quantity of charge on the plates as: Q = C x V Although we have said that the charge is stored on the plates of a capacitor, it is more exact to say that the energy within the charge is stored in an “electrostatic field” between the two plates. When an electric current flows into the capacitor, it charges up, so the electrostatic field becomes much stronger as it stores more energy between the plates. Likewise, as the current flowing out of the capacitor, discharging it, the potential difference between the two plates decreases and the electrostatic field decreases as the energy moves out of the plates. The property of a capacitor to store charge on its plates in the form of an electrostatic field is called the Capacitance of the capacitor. Not only that, but capacitance is also the property of a capacitor which resists the change of voltage across it. 2) Resistor There are many different Types of Resistor available for the electronics constructor to choose from, from very small surface mount chip resistors up to large wirewound power resistors. The principal job of a resistor within an electrical or electronic circuit is to “resist” (hence the name Resistor), regulate or to set the flow of electrons (current) through them by using the type of conductive material from which they are composed. Resistors can also be connected together in various series and parallel combinations to form resistor networks which can act as voltage droppers, voltage dividers or current limiters within a circuit. Resistors are what are called “Passive Devices”, that is they contain no source of power or amplification but only attenuate or reduce the voltage or current signal passing through them. This attenuation results in electrical energy being lost in the form of heat as the resistor resists the flow of electrons through it. Then a potential difference is required between the two terminals of a resistor for current to flow. This potential difference balances out the energy lost. When used in DC circuits the potential difference, also known as a resistors voltage drop, is measured across the terminals as the circuit current flows through the resistor. Most types of resistor are linear devices that produce a voltage drop across themselves when an electrical current flows through them because they obey Ohm’s Law, and different values of resistance produces different values of current or voltage. This can be very useful in Electronic circuits by controlling or reducing either the current flow or voltage produced across them we can produce a voltage-to-current and current-to-voltage converter. There are many thousands of different Types of Resistor and are produced in a variety of forms because their particular characteristics and accuracy suit certain areas of application, such as High Stability, High Voltage, High Current etc, or are used as general purpose resistors where their characteristics are less of a problem. Some of the common characteristics associated with the humble resistor are; Temperature Coefficient, Voltage Coefficient, Noise, Frequency Response, Power as well as a resistors Temperature Rating, Physical Size and Reliability. In all Electrical and Electronic circuit diagrams and schematics, the most commonly used symbol for a fixed value resistor is that of a “zig-zag” type line with the value of its resistance given in Ohms, Ω. Resistors have fixed resistance values from less than one ohm, ( 10MΩ ) in value. Fixed resistors have only one single value of resistance, for example 100Ω, but variable resistors (potentiometers) can provide an infinite number of resistance values between zero and their maximum value. The generic term “Film Resistor” consist of Metal Film, Carbon Film and Metal Oxide Film resistor types, which are generally made by depositing pure metals, such as nickel, or an oxide film, such as tin-oxide, onto an insulating ceramic rod or substrate. The resistive value of the resistor is controlled by increasing the desired thickness of the deposited film giving them the names of either “thick-film resistors” or “thin-film resistors”. Once deposited, a laser is used to cut a high precision spiral helix groove type pattern into this film. The cutting of the film has the effect of increasing the conductive or resistive path, a bit like taking a long length of straight wire and forming it into a coil. This method of manufacture allows for much closer tolerance resistors (1% or less) as compared to the simpler carbon composition types. The tolerance of a resistor is the difference between the preferred value (i.e, 100 ohms) and its actual manufactured value i.e, 103.6 ohms, and is expressed as a percentage, for example 5%, 10% etc, and in our example the actual tolerance is 3.6%. Film type resistors also achieve a much higher maximum ohmic value compared to other types and values in excess of 10MΩ (10 Million Ohms) are available. 3) Diode diode, an electrical component that allows the flow of current in only one direction. In circuit diagrams, a diode is represented by a triangle with a line across one vertex. The most common type of diode uses a p-n junction. In this type of diode, one material (n) in which electrons are charge carriers abuts a second material (p) in which holes (places depleted of electrons that act as positively charged particles) act as charge carriers. At their interface, a depletion region is formed across which electrons diffuse to fill holes in the p-side. This stops the further flow of electrons. When this junction is forward biased (that is, a positive voltage is applied to the p-side), electrons can easily move across the junction to fill the holes, and a current flows through the diode. When the junction is reverse biased (that is, a negative voltage is applied to the p-side), the depletion region widens and electrons cannot easily move across. The current remains very small until a certain voltage (the breakdown voltage) is reached and the current suddenly increases. Early diodes were vacuum tubes, an evacuated glass or metal electron tube containing two electrodes—a negatively charged cathode and a positively charged anode. These were used as rectifiers and as detectors in electronic circuits such as radio and television receivers. When a positive voltage is applied to the anode (or plate), electrons emitted from the heated cathode flow to the plate and return to the cathode through an external power supply. If a negative voltage is applied to the plate, electrons cannot escape from the cathode, and no plate current flows. Thus, a diode permits electrons to flow from cathode to plate but not from plate to cathode. If an alternating voltage is applied to the plate, current flows only during the time when the plate is positive. The alternating voltage is said to be rectified, or converted to direct current. The diode is said to be “forward biased” when conducting current in this direction. When connected within a circuit in the reverse direction (i.e. the “high resistance” direction), the diode is said to be “reverse biased”. A diode only blocks current in the reverse direction (i.e. when it is reverse biased) while the reverse voltage is within a specified range. Above this range, the reverse barrier breaks. The voltage at which this breakdown occurs is called the “reverse breakdown voltage”. When the voltage of the circuit is higher than the reverse breakdown voltage, the diode is able to conduct electricity in the reverse direction (i.e. the “high resistance” direction). This is why in practice we say diodes have a high resistance in the reverse direction – not an infinite resistance. A PN junction is the simplest form of the semiconductor diode. In ideal conditions, this PN junction behaves as a short circuit when it is forward biased, and as an open circuit when it is reverse biased. The name diode is derived from “di–ode” which means a device that has two electrodes. Diodes are commonly used in many electronics projects and are included in many of the best Arduino starter kits. 4) Mosfet FETs have a few disadvantages like high drain resistance, moderate input impedance and slower operation. To overcome these disadvantages, the MOSFET which is an advanced FET is invented. MOSFET stands for Metal Oxide Silicon Field Effect Transistor or Metal Oxide Semiconductor Field Effect Transistor. This is also called as IGFET meaning Insulated Gate Field Effect Transistor. The FET is operated in both depletion and enhancement modes of operation. The following figure shows how a practical MOSFET looks like. The construction of a MOSFET is a bit similar to the FET. An oxide layer is deposited on the substrate to which the gate terminal is connected. This oxide layer acts as an insulator (sio2 insulates from the substrate), and hence the MOSFET has another name as IGFET. In the construction of MOSFET, a lightly doped substrate, is diffused with a heavily doped region. Depending upon the substrate used, they are called as P-type and N-type MOSFETs. The following figure shows the construction of a MOSFET. The voltage at gate controls the operation of the MOSFET. In this case, both positive and negative voltages can be applied on the gate as it is insulated from the channel. With negative gate bias voltage, it acts as depletion MOSFET while with positive gate bias voltage it acts as an Enhancement MOSFET. 5) Radio frequency choke Inductors and RF chokes are basically the same type of electrical components. The difference in design is related to the function the device will perform in a circuit. Most engineers are more familiar with inductors – some think both devices can be used interchangeably – that are prevalent in frequency selective systems, such as a tuner for radio receivers or filters. We can think of RF chokes as applications of inductors. They are designed as fixed inductors with the purpose of choking off or suppressing high-frequency alternating current (AC) signals, including signals from radio frequency (RF) devices, and allowing the passage of low-frequency and DC signals. Strictly speaking, ideally an RF choke is an inductor that rejects all frequencies and passes only DC. To achieve this, the choke (or the inductor) must have a high impedance over the range of frequencies it is designed to suppress, as we can see by inspecting the formula for the value of the impedance, XL: XL = 6.283*f*L Where f is the frequency of the signal and L is the inductance. We see that the higher the frequency, the higher the impedance, so a signal with high frequency will encounter an equivalent resistance (impedance) that will block its passage through the choke. Low-frequency and DC signals will pass through with little power loss. Chokes are normally built with a coil of insulated wires wounded on a magnetic core or a circular-shaped “bead” of ferrite material strung on a wire. They are often wound in complex patterns in order to reduce their self-capacitance. Typically, RF chokes can be seen on computer cables. They are known as ferrite beads and are used to eliminate digital RF noise. As shown in Figure 2, ferrite beads are cylindrical or torus-shaped and normally slipped over a wire. Transmitter Working of transmitter circuit: The transmitter module of our project is made up of a D.C. power source, an oscillator circuit (commonly known as an inverter) and a transmitter coil. The D.C. power source provides a constant D.C. voltage to the input of the oscillator circuit. There, this D.C. power is converted to a high frequency A.C. power and is supplied to the transmitter coil. The transmitter coil, energized by the high frequency A.C. current, produces an alternating magnetic field. DC supply: The D.C. Power Source consists of a simple step down transformer and a rectifier circuit. The transformer steps down the voltage to a desired level and the rectifier circuit convert the A.C. voltage to D.C. Oscillator circuit: The prototype oscillator Circuit designed for the project is a modified Royer oscillator. This oscillator circuit is incredibly simple yet a very powerful design. Very high oscillating current can be achieved with this circuit depending on the semiconductor used. Here high current is necessary to increase the strength of the magnetic field. Working of oscillator circuit: The circuit consists of with two chokes labeled L1 and L2, two semiconductors (Here N-channel Enhancement power-MOSFETS) labeled Q1 and Q2, a resonating capacitor labeled C2 and an inductor (here the transmitter coil) labeled L3. Cross-coupled feedback is provided via the diodes D1 and D2. R1, R3 and R2, R4 are the biasing network for MOSFETS. When power is applied, DC current flows through the two sides of the coil and to the transistors’ drain. At the same time the voltage appears on both gates and starts to turn the transistors on. One transistor is invariably a little faster than the other and will turn on more. The current would continue to increase until the coil (transformer) saturates. The resonating capacitor C causes the voltage across the primary to first rise and then fall in a standard sine wave pattern. Assuming that Q1 turned on first, the voltage at the drain of Q1’s will be clamped to near ground while the voltage at Q2’s drain rises to a peak and then falls as the tank formed by the capacitor and the coil primary oscillator through one half cycle. After that, D1 will be forward bias by more voltage than D2 and hence it will turn on Q2 and cycle repeats. The oscillator runs at the frequency determined by the inductance of the coil, the capacitor value and to a lesser extent, the load applied to the secondary (Source coil). The operating frequency is the familiar formula for resonance, F= 1/2 × π × √(LC) Transmitter coil: For this project the transmitter coil was constructed with 92 mm diameter, 17 swg copper wire and 7 turns. From the equation of inductance of a single layer air core coil, we get inductance L = 8.1 uH. Receiver Working of Receiver: The receiver module of our project is made up of a receiver coil, a rectifier circuit and a voltage regulator IC. And additional buck converter to get more current by decreasing output voltage to 5 volt. An A.C. voltage is induced in the receiver coil. The rectifier circuit converts it to D.C. and the voltage regulator IC helps to maintain a constant limited voltage at the load. The following block diagram gives a general idea of the receiver module: Block Diagram of the Receiver Module Receiver coil: Receiver coil for our project is designed same as transmitter coil with same value. Rectifier: A diode bridge is an arrangement of four (or more) diodes in a bridge circuit configuration that provides the same polarity of output for either polarity of input. When used in its most common application, for conversion of an alternating current (AC) input into direct current a (DC) output, it is known as a bridge rectifier. The essential feature of a diode bridge is that the polarity of the output is the same regardless of the polarity at the input. Operation of bridge rectifier: During the Positive half cycle of the input AC waveform, diodes D1 and D3 are forward biased and D2 and D4 are reverse biased. When the voltage, more than the threshold level of the diodes D1 and D3, starts conducting – the load current starts flowing through it. During the negative half cycle of the input AC waveform, the diodes D2 and D4 are forward biased, and D1 and D3 are reverse biased. Load current starts flowing through the D2 and D4 diodes. Further we can use a capacitor filter to remove ripples present in the output of the bridge rectifier. After the capacitor filter, smooth DC voltage is present at the input of the voltage regulator. Voltage regulator IC: A voltage regulator is an electrical regulator designed to automatically maintain a constant voltage level. It may use an electromechanical mechanism, or electronic components. Depending on the design, it may be used to regulate one or more AC or DC voltages. In this project, LM 7812 voltage regulator IC is used since it allows no more than 12v to the output. Buck converter: It is a totally optional part of the receiver circuit. It is used here to increase current at output. Buck converter is a DC to DC converter which steps down the voltage and, according to it, increases output current. Efficiency of the converter is high (near about 98%) and hence there is a very small amount of power loss in this module. Conclusion The goal of this project was to design and implement a wireless charger for low power devices via resonant inductive coupling. After analyzing the whole system step by step for optimization, a circuit was designed and implemented. Experimental results showed that significant improvements in terms of power-transfer efficiency have been achieved. It was described and demonstrated that resonant inductive coupling can be used to deliver power wirelessly from a source coil to a load coil and charge a low power device. As it was mentioned earlier, wireless charging could be the next big thing. Possible Applications and future work Applications: 1) Smart Phones, Portable Media Players, Digital Cameras and Tablets. 2) Public Access Charging Terminal. 3) Computer Systems 4) Miscellaneous: Wireless chargers are finding its way into anything with a battery inside it. This includes game and TV remotes, cordless power tools, cordless vacuum cleaners, soap dispensers, hearing aids and even cardiac pacemakers. Wireless chargers are also capable of charging super capacitors (super caps), or any device that is traditionally powered by a low-voltage power cable. Future work: To transmit the power to a greater distance, a high power radio frequency amplifier connected with an oscillator is needed. But the construction of the bulky RF power amplifier requires much time and patience. High power vacuum tube transistor amplifier with high current will make the system more efficient. A crystal oscillator circuit might be a better option for the transmitter circuit since it can produce a very high frequency A.C. current. Further effort on this same project can yield some real solutions that can solve the problems of this project. The knowledge of this project will help those who want to design a wireless charging system. 17 Advantages and disadvantage Wireless power transfer will be next biggest move in this integrated technologies world because it has numerous advantages and applications. Advantages: we don’t have need to stick with wires while using any electric device like mobile, laptop, camera etc. complete removal of wires is possible by WPT so system becomes very user friendly and complexity can be reduced. At public places like Malls and stations, complexity of power system can be reduced by WPT. Disadvantages: Major disadvantage of wireless power transfer is high power loss for longer distance. So we can transfer power wirelessly from one point to another very efficiently if distance is too small but loss rapidly increases with distance. 1) Jacob Millman and Christos C. Halkias, ―Integrated Electronics: Analog and Digital Circuits and Systems 2) Muhammad H. Rashid, ―Power Electronics: Circuits, Devices, and Applications 3) Robert L. Boylestad and Louis Nashelsky, Electronic Devices and Circuit Theory 4) William H.Hayt,Jr. and John A.Buck, Engineering Electromagnetics 5) https://en.wikipedia.org/wiki/Wireless_power 6) http://www.engineersgarage.com/articles/wireless-power-transmission Appendix 1) Datasheet of MOSFET IRF540. 2) Datasheet of OA79. 3) Datasheet of LM7812.