Week 8 Cell Signaling Introduction PDF
Document Details
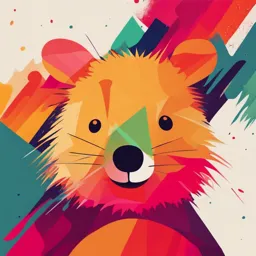
Uploaded by DashingActionPainting9551
Western University
Tags
Summary
This document provides an introduction to cell signaling. It covers the importance of receptors in cell signaling and various modes of intercellular signaling, including endocrine, paracrine, and synaptic signaling. Additionally, it describes different types of signaling molecules, such as hormones and cytokines.
Full Transcript
Week 8: Cell Signaling Introduction What is the Importance of Receptors in Cell Signaling? - Cells recognize and respond to environmental cues via receptors → cells communicate and coordinate by sending and receiving signals, and each cell expresses a variety of receptor proteins - wi...
Week 8: Cell Signaling Introduction What is the Importance of Receptors in Cell Signaling? - Cells recognize and respond to environmental cues via receptors → cells communicate and coordinate by sending and receiving signals, and each cell expresses a variety of receptor proteins - with most of them being on the plasma membrane facing the ECM. The receptors allow the cell to respond to particular signals, which can originate from (1) other cells, as well as (2) the environment: 1. Environmental Signals → environmental signals can occur at the level of the cell, as well as the senses: a. Cellular Environment → cells are able to detect nutrients, protons, nucleic acids, osmotic pressure, fluid shear stress, as well as xenobiotics. b. Sensory Signaling → sensory signaling involves the detection of external signals by sensory receptors, which can be designed for light (rhodopsin), smell (olfactory GPCRs), taste (GPCRs, ion channels, transporters), sound, balance, as well as touch (mechanoreceptors). 2. Endogenous Extracellular Signaling Molecules → signals that originate from other cells, and this is a means for cells to talk to each other. In this process, one cell will release a signal into its environment, for example, which will allow another cell to read the message. The different ways in which these signaling molecules work in the body are as follows: a. Secreted → signals can be secreted from the signaling cell into the extracellular space, and examples of secreted signals include hormones and neurotransmitters, which are stored in intracellular vesicles. b. Released → signals can be released by passive diffusion through channels in the PM, and examples of these signals include ATP and prostaglandins. Note that ATP can also be co-released with hormones and neurotransmitters, as most vesicles that store these signaling factors package them together with ATP. c. Liberation → liberation involves the releasing of PM-anchored ligands, which are covalently attached to the membrane, by matrix metalloproteinases, which cleave the ligand and release the active portion of the molecule. Examples of such signals include growth factors and cytokines. d. Exposed → exposed signals are exposed to the ECM, but remain tightly bound to the surface of the signaling cell. Hence, when another cell comes into physical contact with an exposed signaling molecule, then signaling can take place. An example of such a signal are ephrins. e. ECM Proteins → cells can have receptors for extracellular matrix proteins, and so they are also involved in signaling. An example of this is integrins. What are Modes of Intracellular Signaling? - There are different modes of intercellular signaling, which are as follows: 1. Endocrine Signaling → endocrine signaling occurs when hormones are released into the bloodstream or the lymphatic system, and these can travel through the body via these conduits to reach the target cells. This is a fairly long distance for diffusion of the signaling factors, which can act on target cells that express a receptor for the ligand. Due to the long distance, hormone signaling is relatively slow compared to the other modes. Examples of endocrine signaling involves the following: a. Insulin → insulin released from the pancreas promotes glucose uptake throughout the body. b. Parathyroid Hormone (PTH) → PTH is secreted from the parathyroid gland, and acts on PTH receptors in the bone, kidneys, as well as intestines. 2. Paracrine Signaling → paracrine signaling occurs when signaling factors are secreted, which act as local mediators that impact target cells in the immediate environment (para = near). Examples of paracrine signaling are as follows: a. Platelet-Derived Growth Factor (PDGF) → this is involved in wound healing. b. Parathyroid Hormone Related Peptide (PTHRP) → locally released PTHRP (not released by parathyroid, but the periphery of the body) activates PTH1 receptors in the bone and regulates mineralization. 3. Synaptic Signaling → this is a specialized form of paracrine signaling, and is involved in regulating neuronal communication (forms a synapse). This is a raid form of signaling, and is a means to allow for adaptive changes required for behavioral responses or reflexes: a. As depicted above, neurons (nerve cells) extend long axons, which contact other neurons at synapses → these neurons can also form synapses with multiple cells, and so is a rapid mode of signaling. Neurotransmitters include acetylcholine, catecholamines (dopamine, adrenaline, noradrenaline), serotonin, endorphins, GABA, corticotropin releasing factor, etc. that are typically stored in intracellular vesicles and released into synapses. However, an exception to this is endocannabinoids, which are released from the postsynaptic neuron that travels back across the synapse and acts on the presynaptic neuron. 4. Juxtacrine Signaling → juxtacrine signaling, also known as contact-dependent signaling, requires physical contact between adjacent cells, or between the cell and the ECM. The ligand, in this scenario, is protruding from one cell and activates a receptor in the plasma membrane of neighboring cells. Examples of such receptors are ephrin receptors and adhesion GPCRs. Therefore, signaling molecules remain bound to the surface of the signaling cell, and activate target cells that come into contact with the signaling cell. This is an important form of signaling for developmental biology: 5. Autocrine Signaling → autocrine signaling involves cells releasing a signaling molecule that acts back upon receptors expressed on its own surface to control its activity. Examples of autocrine signaling molecules include cytokine interleukin-1 cells in immune cells, as well as vascular endothelial growth factor (VEGF) in cancer cells. Neurotransmitters, such as noradrenaline and 5HT, are also autocrine signaling molecules that bind to presynaptic autoreceptors: 6. Protease-Dependent Signaling → protease-dependent signaling involves the proteolytic activation of precursor proteins, an example of this is a class of GPCRs called proteinase-activated receptors. These receptors have an N-terminal and within this terminus is the activating ligand. However, the activity of this ligand is mashed by extra amino acids → what happens is that a protease, such as thrombin, comes along and cleaves a part of the N-terminus - thereby exposing the activating ligand that can then tuck back into the top of the receptor and activate it. Hence, in this case, the receptor is its own ligand, but remains inactive until the inactivating component of the ligand is proteolyzed. Note that this is a form of irreversible signaling. In addition, there are cases of membrane-anchored proteases, which can release tethered ligands from extracellular surfaces by metalloproteinase activity. Furthermore, the conversion of pro-hormone to active form by proteinase activity also involves protease-dependent signaling, which can occur either intracellularly (i.e. endorphins) a well as extracellularly (angiotensin II): a. As depicted above, angiotensin I is the inactive form of a hormone, and is converted by angiotensin-converting enzyme (ACE) to produce angiotensin II - the active form of the hormone. - Note that some endogenous ligands can play multiple signaling roles → for example, noradrenaline released from adrenal glands can act as a hormone, a neurotransmitter, or an autocrine factor. However, from the perspective of the receiving cell, there is no difference between hormonal, paracrine, and autocrine signaling. How do Cells Recognize and Respond to Environmental Signals? - The below diagram depicts 4 cells, in which the top cell sends out a chemical messenger that 3 other cells respond to → cell A has a receptor that is similar in shape to the chemical messenger, and so binds to the chemical receptor. Cells B and C, however, do not have appropriate receptors - disabling the messenger from binding to these cells. The important thing to note from this schematic is that a cell cannot respond to an extracellular cue unless it possesses a receptor that can recognize the cue: What is Signal Transduction? What are Key Concepts of Receptor-Ligand Binding? - Signal Transduction → cell surface receptors are able to transduce signals into cells, which essentially means to convert (something, such as energy or a message) into another form. This is the whole premise of signal transduction: - Receptor-Ligand Binding Concepts → there are several concepts associated with receptor-ligand binding, which are as follows: 1. Affinity → this is a term used to describe how tightly a drug binds to its receptors, and is characterized by the parameter KD = koff/kon (rate at which signaling molecule comes off the receptor divided by the rate at which the signaling molecule gets on the receptor). 2. Specificity → binding is selective, such that receptors bind preferentially to ligans that fit well into its binding state, as discussed. 3. Saturability → ligand-binding is limited by the number of receptors, which can become saturated at a certain concentration of ligand. After this point, adding more ligands does not result in more receptor-ligand complexes. 4. Reversibility → the ability to bind and dissociate from a receptor is referred to as reversiblit, and most ligands bind reversibly - with the exception of covalently-bound ligands. 5. Competition → a binding site can only accommodate one ligand at a time, and so if 2 or more ligands are present, they will compete with each other for the binding site. 6. Agonist → an endogenous ligand or drug that binds to a receptor and promotes its activation is referred to as an agonist. 7. Antagonist → a ligand that binds to a receptor but does not activate it is referred to as an antagonist. What are Cellular Determinants of Receptor Signaling? - There are varying ways in which different cells respond to a particular agonist, and the reason why they vary as follows: 1. Receptor Expression Level → the availability of receptors will determine cellular responses, as cells that lack receptors for a particular signal cannot respond to the signal. In contrast, cells with greater receptor density may respond at lower agonist concentrations and/or activate minor pathways. 2. Receptor Variants → ligands may bind to distinct receptor subtypes in different cells. 3. Intracellular Signaling Components → a receptor may activate different pathways in different cells, which depends on intracellular factors available to integrate and regulate receptor signals. Examples of this are the complement of kinases, ion channels, phosphatases, as well as substrates. How does Signal Turnoff Occur? - There are many ways in which signal turnoff can occur, as follow: 1. Metabolism → endogenous agonists may be deactivated metabolically, and examples of this include the hydrolysis of ACh by cholinesterases, as well as the breakdown of peptide and protein hormones by proteases. 2. Reabsorption → endogenous agonists may be reabsorbed from extracellular spaces via specialized uptake proteins (i.e. transporters for dopamine, adrenaline, noradrenaline, serotonin, and prostaglandins). 3. Excretion → drugs that mimic endogenous agonists can be metabolized by the liver enzymes and/or excreted via the urine. 4. Signal Limiting → receptor activation can also trigger intracellular processes that limit signaling, such as G-protein uncoupling and receptor internalization. What are Molecular Switches of Cellular Signaling? - There are many molecules switches for cellular signaling: 1. G-Proteins → heterotrimeric G-proteins and small Ras-like G-proteins belong to a superfamily of GTPases, where activation and deactivation correspond to GTP-binding and hydrolysis, respectively. For most G-proteins, these changes occur on a time scale of seconds to minutes - being slower relative to many biochemical processes. The regulation of G-protein activation state is performed by other proteins: a. GEFs → guanine nucleotide exchange factors promote GDP dissociation, and thus facilitates activation by GTP. b. GAPs → GTPase accelerating proteins promote the hydrolysis of GTP, thereby deactivating G-proteins. c. GDIs → guanine nucleotide dissociation inhibitors inhibit GDP dissociation, and thus impede activation. 2. Protein Phosphorylation → protein phosphorylation is a ubiquitous strategy to either turn on or off signaling. It involves the reversible covalent modification of the signal, with proteins dephosphorylated by phosphatases. Kinases undergo conformational changes in response to diverse inputs, which then regulates kinase catalytic function (phosphorylation). During phosphorylation, ATP is cleaved to ADP - with the phosphate that is released being covalently attached to a protein. Note, however, that phosphorylation does not always mean activation, as it can also mean inactivation - depending on the type of protein: What are General Characteristics of Cell Signaling? What are Signaling Cascades? - The below diagram depicts the general process of cell signaling → the intracellular signaling proteins alter the activity of target proteins, which results in a cell behavior change (response). The characteristics of cell signaling are as follows: 1. First Messengers → endogenous agonists or mimics are referred to as first messengers, and these are agonists that bind receptors and regulate intracellular signaling processes. Alternatively, receptors may be or control ion channels, leaving altered levels of intracellular ions. 2. Second Messengers → second messengers include inositol triphosphate (IP3), diacylglycerol (DAG), cyclic nucleotides (i.e. cAMP, cGMP), as well as calcium ions. - Signaling Cascades → as depicted below, signaling cascades are multistep biochemical reactions that occur in response to the activation of a receptor. At each step in a signaling cascade, the “product” of one step becomes the activator or substrate of the next: - Signal Amplification → amplification may occur, if for example a kinase phosphorylates multiple substrate molecules. Hence, the result is a bigger and bigger signal as the signal propagates through the cell: - Receptor Reverse → in some cases, there is redundancy built into the cell such that there are more receptors than required to produce the maximal cellular response, and these are referred to as spare receptors. - Signal Processing → there are other ways in which signals can be processed, and the strength of the receptor signal depends on various factors which are as follows: 1. Amplification → amplification of the signal occurs via the activities of downstream kinases, as well as other downstream enzymes. 2. Attenuation → signals are also subject to attenuation, which occur via phosphatases, counter-movement of ions, 2nd messenger breakdown, as well as GTPase activating proteins. 3. Scaffolding Proteins → the availability of scaffolding proteins also determine the strength of a receptor signal, as they bring components of signaling cascades into close proximity such that increased local concentrations of soluble factors result in an increased efficiency of signal propagation. In addition, scaffolding proteins can also bring in deactivating factors such as GTPase activating proteins → this allows for the temporal focusing via negative regulators, such that the signal can be turned off more rapidly. 4. Tachyphylaxis/Desensitization → in the long-term, the cell can also be made to undergo desensitization in a variety of ways such as changing ion channel conformations, receptor phosphorylation, substrate depletion, receptor internalization/degradation, as well as receptor mRNA downregulation. - Set of Reactions → signal transduction pathways involve a set of cascading reactions, which are often portrayed as a linear set of reactions. However, the pathways are not always linear due to their ability to converge, diverge, as well as cross-link: 1. Pleiotropy → pleiotropy refers to the ability of a single signal to cause multiple outcomes, which can be due to there being multiple different subtypes of the receptor such that different receptors trigger different intracellular pathways. An example of this is GPCRs, as their activation may trigger multiple signaling cascades (i.e. transcription, ion channel activity, cell proliferation, as well as cell survival): 2. Convergence → convergence involves multiple signals activating a common outcome, and the below diagram depicts an example of this: a. RTKs can activate PI3 kinase via p85, whereas GPCRs can activate PI3 kinase via p101 → due to PI3K being involved in the conversion of PIP2 to PIP3, both the RTK and GPCR pathways lead to the same outcome. However, p85 and p101 have different preferences for P13K subtypes. 3. Signal Integration → if a protein needs to be phosphorylated in 2 different places before it is activated, for example, the phosphorylation can occur via 2 different kinases. Hence, blocking either of the kinases can inhibit the activation of the protein: What Occurs when Cell Communication Fails? - There are numerous human diseases that are caused by malfunctions in signaling - with either too much or too little signaling. This is why a lot of drugs involve targeting receptors to induce activation or deactivation of receptors, thereby induce or inhibit signaling: 1. Type I Diabetes→ under normal circumstances, food enters the body and is broken down such that sugar molecules enter the bloodstream. The sugar then stimulates cells in the pancreas to release insulin, which travels through the blood - stimulating liver, muscle, and fat to take up sugar for later use. However, in type I diabetes, pancreatic cells that produce insulin are lost - resulting in the insulin signal also being lost. This results in sugar accumulating to toxic levels in the blood, such that without treatment, diabetes can lead to kidney failure, blindness, and heart disease in later life: 2. Type II Diabetes → in type II diabetes, instead of there being a loss in signal, the target cell ignores the signal. Hence, although insulin is produced in type II diabetics, the target cell loses its ability to respond - leading to hyperglycemia and abnormal insulin secretion. The end result, however, is the same → blood sugar levels become dangerously high. An available treatment for type II diabetes is glucagon-like peptide 1 receptor agonists, which lower blood glucose levels by increasing insulin release, decreasing glucagon release, and slowing gastric emptying: