Additive Manufacturing – principles and applications PDF
Document Details
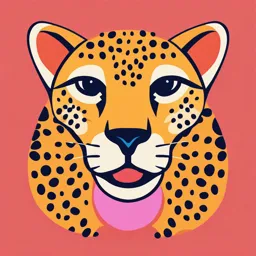
Uploaded by ReverentBowenite2086
The University of Sheffield
Candice Majewski
Tags
Summary
This document provides an overview of additive manufacturing principles and applications, focusing specifically on polymer laser sintering. The presentation covers factors affecting part properties, including pre-process, in-process, and post-process considerations. It also touches upon material requirements, structure of parts, and characterization methods. The document is intended for an undergraduate audience.
Full Transcript
Additive Manufacturing – principles and applications MEC454 Advanced understanding of polymer Laser Sintering Candice Majewski (she/her) [email protected] Content Re-cap of Laser Sintering The process Why are we interested? Factors...
Additive Manufacturing – principles and applications MEC454 Advanced understanding of polymer Laser Sintering Candice Majewski (she/her) [email protected] Content Re-cap of Laser Sintering The process Why are we interested? Factors affecting part properties Pre-process In-process Post-process Scientific understanding of the process Material requirements Structure of final parts Characterisation techniques 2 Context Over the course of these sessions we cannot cover everything… Literature searches on the topics mentioned will provide you with a much greater range and depth of understanding 3 Re-cap of Laser Sintering 4 The process Parts built by selectively scanning and sintering cross-sections of powdered material Hopkinson et al – Rapid Manufacturing – An industrial revolution for the digital age 5 Why are we interested? No support structures (polymers) Allows more complex designs Assemblies produced as one Less post-processing required Time-saving Better surface finish on down-facing surfaces Relatively high mechanical properties & stability of properties Build through build volume (not area) 6 Factors affecting part properties 7 What can we change? The process chain gives us a good idea of how we can affect our parts. Three main areas of influence: Pre-process In-process Post-process Most of what we do will be aimed at specific properties (mechanical, surface finish, accuracy, repeatability etc.) Main focus of this lecture is on mechanical properties (Nylon- 12 unless stated) 8 What can we change? Pre-process E.g. material Post-process properties, fillers etc. E.g. finishing In-process methods E.g. pre-heat temperature, energy input 9 Factors affecting part properties: pre-process 10 Pre-process Material choice (type and morphology) Fillers (type and level) Thermal history 11 Material Choice Choice of material will affect the end properties Traditionally Nylon-12 based Inclusion of fillers Other materials (e.g. elastomers, PEEK, polypropylene) The first step is selecting the correct material! What is your end application? Which are the critical properties? What level of these properties do you actually need? 12 Material Choice Bear in mind that you are unlikely to obtain the same properties as from Injection Moulding E.g. for Nylon-12, Strength and Modulus are comparable, whereas Elongation at Break is considerably lower Certain materials are more susceptible to in-process variations Perhaps a consideration if your properties are critical 13 Morphology Consider the LS process chain… Powder deposition is crucial… If we don’t have a smooth powder base to start with, what will be the effect on our parts? Low density Poor accuracy/surface finish Over-exposure of certain areas of parts (non-homogenous parts) What can we influence? Particle size Particle shape Powder agglomeration 14 Morphology Particle size & distribution This sounds like it should be simple, but in reality is a very complex issue… Size and distribution will have major effects on powder packing, and on resultant porosity of parts Average particle size (Nylon-12) is ~50 microns but with a range from ~10-90 microns 15 Morphology Particle size In general – larger particles will lead to poorer surface finish and lower density, but… Smaller particles can suffer more from static, leading to poor deposition Small particles susceptible to flow of nitrogen within chamber (this will also affect the laser window) 16 Morphology Particle size distribution Range of particles can assist in packing, leading to a denser part bed and (depending on other parameters too) higher mechanical properties Inclusion of very fine particles can sometimes aid flow 17 Morphology Particle shape Largely dependent on material and production method Different methods will provide different shapes of powder E.g. grinding may give us ‘cornflakes’ (see image) Generally accepted that smooth, spherical powder shapes are preferred 18 Morphology Powder agglomeration Powder can ‘clump’ together both before and during a build Static build-up (often caused by sieving) Additives can help flow, but have an effect on properties Moisture absorption Drying may be necessary in order to reduce excess moisture Material type will influence how much of a factor this is 19 Morphology Powder agglomeration Can lead to problems with deposition on the build area Can become worse as powder temperature increases (unless moisture is the cause, in which case the opposite can be true!) In gravity-fed systems, this can lead to ‘bridging’ Powder will not flow from chute Forced vibration of delivery chute may help 20 Fillers We know there are a range of filled materials available, but what are the issues with this? Compromise on properties – often we obtain a favourable effect on one property, at the detriment of another Filler ratios – for some materials an excess of filler will cause a decrease in properties Homogeneity of mixing: Mechanical mixing is simple, but difficult to maintain good distribution of filler both during mixing and storage More complex methods, e.g. co-extrusion followed by grinding can offer some improvements (but can affect particle shape…) 21 Fillers Inclusion of a filler will necessarily affect the resultant mechanical properties E.g Duraform GF (Nylon-12 filled with glass beads) Build parameters are almost identical for GF and standard PA – we are sintering Nylon 12 and not the glass Resultant parts are a composite of glass beads surrounded by Nylon-12 Majewski CE, Zarringhalam H, Toon D, Ajoku U, Hopkinson N & Caine MP (2009) The use of off-line part production to predict the tensile properties of parts produced by Selective Laser Sintering. J MATER PROCESS TECH, 209(6), 2855-2863 22 Fillers Young’s Modulus (stiffness) – 157 % increase between 0 and 50 % glass 8000 7000 6000 Young's Modulus (MPa) 5000 4000 3000 2000 1000 0 0 5 10 15 20 25 30 35 40 45 50 % glass 23 Fillers Elongation at Break (ductility) – 84 % decrease between 0 and 50 % glass 9 8 7 Elongation at Break (%) 6 5 4 3 2 1 0 0 5 10 15 20 25 30 35 40 45 50 % glass 24 Fillers Nano-materials are becoming increasingly relevant throughout many industries Think of all the fuss around graphene… Major considerations with respect to health and safety 25 Thermal history Don’t forget that LS is a thermal process Any un-sintered material has been sat in a heated bed temperature for a substantial amount of time This has effects on its molecular weight 26 Thermal history Molecular weight Decrease in Melt Flow Rate over successive builds Increase in molecular weight MFR's and Melt Pts 188 50 187.5 Melt Temperature (°C) 40 MFR (g/10 min) 187 30 186.5 20 10 186 0 I 185.5 virgin 1 2 3 4 5 6 7 MFR Melt Pt. Gornet TJ, Davis KR, Starr TL, Mulloy KM. Characterization of selective laser sintering materials to determine process stability. In: Solid freeform fabrication symposium proceedings; 2002. p. 546–53. 27 Thermal history Common in LS to use a mix of virgin and re-cycled material in order to reduce costs but maintain good properties Virgin gives greater repeatability, whilst recycled can give increased properties (notably Elongation at Break) Thermal conditioning can be used to improved EaB (bear in mind the cost and time associated with this) 28 Thermal history 12 10 Elongation at Break (%) 8 6 8.83 4 4.75 2 0 Virgin Recycled 29 Thermal history 30 Thermal history After a certain amount of re-use, surface finish is affected (orange peel effect) THE EFFECT OF EMPLOYING AN EFFECTIVE LASER SINTERING SCANNING STRATEGY AND ENERGY DENSITY VALUE ON ELIMINATING “ORANGE PEEL” ON A SELECTIVE LASER SINTERED PART W Yusoff and A Thomas, Iternational Association for Management of Technology, 2008 proceedings 31 Factors affecting part properties: in-process 32 In-process Build orientation Evidence of anisotropy for parts produced in different orientations To a lesser extent than for some other processes (e.g. FDM) Different degrees for different mechanical properties We can work around this for certain parts, or when conducting research, but how can we plan for this in general real-life terms? 33 Build orientation All values in MPa Property X axis Y axis Z axis Tensile Strength 49 45 41 Flexural Strength 60 64 58 Compressive Strength 54 53 52 AJOKU, U.... et al, 2006. Investigating mechanical anisotropy and end-of-vector effect in laser-sintered nylon parts. Proceedings of the Institution of Mechanical Engineers, Part B : Journal of Engineering Manufacture, 220 (7), pp. 1077-1086 34 Part bed temperature Pre-heat build area prior to sintering, but… Laser sintering systems known to have somewhat uneven temperature distributions across the build area, and between systems Not uncommon for there to be several degrees difference between the edges and centre of the build area (often very cool at the extremities) Nowadays there are various system upgrades and enhancements available to improve this 35 Part bed temperature Laser sintering of polyamides and other polymers, Goodridge et. al,., Progress in Materials Science, Volume 57, Issue 2, February 2012, Pages 229–267 36 Part bed temperature Effect of bed temperature on density, for example… Part bed temperature of 182 deg C, full density obtained at a default energy density of 0.0284J/mm2 Part bed temperature of 178 deg C, same energy density, density of a sintered part decreases by ~4% Tontowi AE, Childs THC. Density prediction of crystalline polymer sintered parts at various powder bed temperatures. Rapid Prototyp J 2001;7:180–4. 37 Part bed temperature Effect of bed temperature on mechanical properties… Figure 12: Distribution of The % Elongation Values on Figure10: Distribution of The UTS Values on The LS the LS Build Platform Build Platform 43.2 41.6 36.7 44.9 43.3 44.2 46 45 12.3 9.9 44.7 39.1 46.1 11.7 9.9 7.6 10.1 46.2 42.8 39.4 11.5 11.2 45 44.1 45.9 40.9 38.5 14 11.5 10.4 8.5 40 43.6 9 36.8 42.5 45.5 43.7 9.9 35 44.9 12 10.6 11.6 44.45 10.3 9.4 8.5 30 44.7 44.3 9.9 10 11.5 10.3 11.3 25 UTS (MPa) 42.7 8 6.8 9 9.6 10.7 20 42 Elong (%) 6 10 10.6 15 10 4 5 8.4 E 32.8 2 0 E 0 D D 6.5 1 1 C 2 C 2 3 3 B B 4 4 A A 5 5 6 6 A B C D E A B C D E Research conducted at Loughborough University, Dr Naguib Saleh 38 Processing parameters Effect of main processing parameters on mechanical properties… Zarringhalam H. Investigation into crystallinity and degree of particle melt in selective laser sintering. PhD thesis, Loughborough University, UK; 2007. 39 Processing parameters Energy Density Caulfield et al define energy density as: Energy Density = Laser Power/(Scan Spacing × Laser Scan speed) Does not take into account part bed temperature or layer thickness, but highlights the effect of energy input 40 Processing parameters Energy Density E.g. higher ED = higher ductility (to a certain point) For some materials time is also a factor (i.e. changing scan speed has a different effect than laser power) Caulfield B, McHugh PE, Lohfeld S. Dependence of mechanical properties of polyamide components on build parameters, in the SLS process. J Mater Process Technol 2007;182:477–88. 41 Processing parameters Volumetric Energy Density Volumetric Energy Supplied by the Laser EMR Energy Require to Melt the Layer 𝐸𝑀𝑅 = (𝑃×𝑉𝐶/V𝑠 × 𝑉𝐵 × 𝑧)/ P=Laser power Tm = Melt temp. [𝐶𝑝(𝑇𝑚 −𝑇𝑏)+h𝑓]×(p𝑠) ×(p𝑑) SCNT = Scan count Tb= Part bed temp SCSP = Scan spacing hf = Heat of fusion bs= Beam speed ps= Density of solid Tl= Layer thickness pd = Packing density Cp= Specific heat See work by University of Louisville on Energy Melt Ratio, e.g. Starr et. al., The effect of process conditions on mechanical properties of laser-sintered nylon, Rapid Prototyping Journal, Volume 17, Issue 6 42 Processing parameters Volumetric Energy Density Just beyond the energy needed to fully melt a layer, we reach our highest possible properties Gornet and Starr, Mechanical and Fatigue Properties of Polymers for Direct Digital Manufacturing, SAMPE 43 Factors affecting part properties: post-process 44 Post-processing Even ‘standard’ techniques will have unwanted effects E.g. rounding of corners or discoloration when bead-blasting Infiltration May provide increases in mechanical properties Often specific to base material Don’t forget – this means added time, cost and complexity for our production process… 45 Post-processing End-of-build actions E.g. for Duraform EX we must leave heaters and nitrogen on after build is complete Part warpage Discoloration Part removal Parts must be allowed to cool before removal (below Tg, depending on how impatient you are…) 46 Other techniques Consider how these factors might vary for different processes, e.g.: What effect might energy input method, presence of ink etc. have when considering the High Speed Sintering process? How can you apply the same type of analysis to techniques in other AM categories? 47 Scientific Understanding 48 At its most basic… ‘zap some powder with a laser and melt it’ But… in reality there is a lot more to it than that! 49 Content Material requirements Sintering behaviour Structure of parts Characterisation methods 50 Material requirements We know that the majority of materials for Laser Sintering are based on Nylon-12*, but why? Ideally suited to laser sintering due to large window between melt and crystallisation temperature Bed temperature keeps part in a molten state to allow uniform stress distribution during cooling (less shrinkage and distortion) Narrow melt range allows use of higher bed temperatures *semi-crystalline polymer – major focus here 51 Material requirements Crystallisation Bed Temperature 2.00 1.50 1.00 0.50 Heat flow (W/g) 0.00 120 130 140 150 160 170 180 190 200 210 220 230 -0.50 -1.00 -1.50 -2.00 -2.50 Temp (˚C) Melt Point 52 Material requirements Amorphous polymers Tend to have lower mechanical properties but greater dimensional stability (lack of shrinkage) Elastomers ‘Rubber-like’ materials (flexible, high ductility) Particularly relevant e.g. for the sports goods industry But… more difficult to sinter & may need infiltration 53 Material requirements 54 Material requirements Stable Sintering Region Beyond a certain temperature, degradation of material occurs The ‘best’ semi-crystalline materials will have a wide region between melt and degradation points Among other things, this helps account for lack of repeatability in and between systems 55 Material requirements Vasquez, M., Haworth, B. and Hopkinson, N. (2013), Methods for quantifying the stable sintering region in laser sintered polyamide-12. Polym Eng Sci, 53: 1230–1240. doi: 10.1002/pen.23386 56 Sintering behaviour Various models exist to predict sintering behaviour, and mechanical properties of sintered parts No such equations proved for polymer LS But, Frenkel model gives us a basic idea of key properties r 3gt 2 x r 2x 2x r 2 rh o x = Half neck thickness (mm) r = Particle radius (mm) Frenkel Viscous t = Time (s) Sintering Model g = Surface tension (Jm-2) ho = Viscosity (Nsm-2) 57 Sintering behaviour Viscosity We can see from the Frenkel equation that viscosity is important, but again a complex issue… Too high, and we cannot quickly melt the material – less density in the resultant parts Too low, and we lose accuracy Surface tension Another key property, but difficult to measure accurately for powders Temperature-dependency 58 Structure of parts Degree of Particle Melt LS parts are often comprised of regions with varying proportions of melting: Some particles fully melted and crystallised Other areas are partially melted, leaving an un-molten core Unmolten particle fused to edge Spherulite from fully melted & crystallised particle Unmolten particle core Spherulite from melted & crystallised region Zarringhalam, H., Hopkinson, N., Kamperman, N.F., de Vlieger, J.J., 2006, Effects of processing on microstructure and properties of SLS Nylon 12, Materials Science and Engineering A, Vol. 435-436, pp 172-180, ISSN 0921-5093 59 Structure of parts Partial melting occurs as a result of insufficient energy input to fully melt large particles. E.g. Duraform PA Average particle size 58mm Range from 25mm to 92mm (plus ‘fines’) For constant energy input, relative proportion of melted material decreases as particle size increases Melted material Un-melted material 60 Structure of parts Differential Scanning Calorimetry (DSC) for laser-sintered Nylon-12 parts show two distinct melt peaks, which relate to the melted and un-melted regions within the part* Fully melted material possesses single peak 0 -0.5 Heat flow (W/g) -1 -1.5 -2 -2.5 150 160 170 180 190 200 Temperature (deg C) 61 Structure of parts This suggests we may need to treat fully- and partially- melted SLS parts as separate materials One homogenous, single-phase material One quasi-composite material, comprised of both melted and un-melted material 62 Structure of parts Research has shown different trends, notably Tensile Strength, between fully and partially melted material Majewski C, Zarringhalam H & Hopkinson N (2008) Effect of the degree of particle melt on mechanical properties in selective laser-sintered Nylon-12 parts. 63 Characterisation methods A wide range of techniques that we can use to asses our materials, e.g.: Differential Scanning Calorimetry (DSC) Melt Flow Indexing (MFI) Hot Stage Microscopy (HSM) Particle Size Analysis (PSA) Thermo-gravimetric Analysis (TGA) 64 Characterisation methods Differential Scanning Calorimetry: Thermal analysis technique Measure difference in heat flow between a sample and a known reference, with respect to time and temperature For example, if we heat this reference sample at a certain rate, how much energy do we need to put into our test sample to match this? Use for measurement of key thermal properties, e.g. Temperatures Range 2.00 1.50 Energy requirement 1.00 0.50 Heat flow (W/g) Part properties (DPM) 0.00 -0.50 120 130 140 150 160 170 180 190 200 210 220 230 -1.00 -1.50 -2.00 -2.50 Temp (˚C) 65 Characterisation methods Melt flow indexing: Measurement of material viscosity Measure mass of material flowing through a specific size of orifice, under a certain load, over a given time This gives an indication of molecular weight: High melt flow rate = low viscosity = low molecular weight Only a comparative technique – not an exact science http://www.chenesah-intl.eu 66 Characterisation methods Hot Stage Microscopy: As you might guess from the name… Standard microscopy but on a heated base Use to investigate particle flow behaviour, in particular: Temperature at which necking begins Time taken for complete particle agglomeration http://www.rsc.org/ej/CE/2013/c2ce26575c/c2ce26575c-f11.gif 67 Characterisation methods Particle Size Analysis: Analysis of size, range of sizes, and sometimes shape of our particles Variety of methods, e.g.: Sieving – range of sieve sizes (slow, inaccurate) Optical microscopy (slow) Laser Diffraction (accurate but expensive) http://www.azom.com 68 Characterisation methods Thermo-gravimetric Analysis: Thermal analysis technique Measure mass of sample at different temperatures This gives indication of physical or chemical changes (e.g. oxidation, vaporisation etc.) Particular use in LS to investigate degradation temperature: We tend to take the degradation temperature as the point at which we have ‘lost’ 1% of our mass 69 Characterisation methods A wide range of techniques that we can use to asses our parts, e.g.: Tensile Testing Compression testing Surface profilometry Hardness testing 70 Characterisation methods Tensile testing: Measurement of properties of parts when subject to tension Most common properties to be quoted for AM http://www.matweb.com/refer ence/tensilestrength.aspx 71 Characterisation methods Tensile testing: Common to quote Ultimate Tensile Strength, Young’s Modulus (stiffness), Elongation at Break (ductility) http://www.instron.us/wa/applications/test_types/tension 72 Characterisation methods Compression testing: Measurement of properties of parts when subject to compression Basically ‘put it in the machine, squash it, see how it behaves’ Of particular interest with e.g. elastomers (sportswear /equipment?) http://www.matweb.com/reference/compressivestrength.aspx 73 Characterisation methods Surface profilometry: Measurement of surface roughness properties of a part Stylus is dragged along the surface, and change in height is recorded Newer methods involve laser measurement or 3D surface capture Range of parameters which can be measured, but most common is Ra Assessment of surface profile data acquired by a stylus profilometer, Dong-Hyeok Lee and Nahm-Gyoo Cho 2012 Meas. Sci. Technol. 23, 10 74 Characterisation methods The most crucial thing is to fully specify exactly what you have tested, and how… Always refer to British/ISO/ASTM standards for procedures, and state these Development of AM-specific standards is well underway Until we have a full set of these standards, think very carefully about whether, and how, you may need to modify existing standards to account for differences between AM and more traditional manufacturing methods 75 Do you have questions? 76