PHYS 5024 Week 6 Notes Schmidt PDF
Document Details
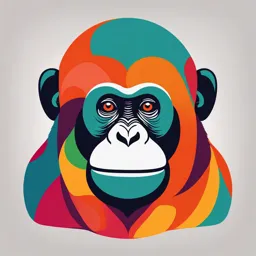
Uploaded by ResourcefulMimosa4070
Sonoran University of Health Sciences
Rodney A. Rhoades and David R. Bell
Tags
Summary
These notes cover various aspects of medical physiology, including spirometry, cardiac circulation, and related topics.
Full Transcript
PHYS 5024 Week 6 Notes Schmidt Medical Physiology: Principles for Clinical Medicine, by Rodney A. Rhoades and David R. Bell, Chapters 16, 18 & 19 Reminder: watch the narrated PowerPoint presentation on Spirometry before your week 6 spirometry lab session. Spirometry...
PHYS 5024 Week 6 Notes Schmidt Medical Physiology: Principles for Clinical Medicine, by Rodney A. Rhoades and David R. Bell, Chapters 16, 18 & 19 Reminder: watch the narrated PowerPoint presentation on Spirometry before your week 6 spirometry lab session. Spirometry -tidal volume (VT): volume of air leaving the lungs during a breath (typically about 500 ml) -total lung capacity: maximum air volume in lungs at maximal inhalation (about 6 L) -functional residual capacity (FRC): air remaining in lungs at the end of expiration during a normal breath; two parts..... *expiratory reserve volume (ERV): additional air that can be forced out of the lungs by forced expiration *residual volume (RV): additional that cannot be forced out by forced expiration -inspiratory reserve volume (IRV): maximum additional volume of air that can be inhaled beyond the tidal volume -vital capacity (VC): IRV + VT + ERV *a measure of VC is the forced vital capacity (FVC): the subject inspires maximally then rapidly exhales as completely as possible. *FEV1 (forced expiratory volume): the volume exhaled in the first second, typically about 80% of total FVC *FEF25-75: air flow rate (L/sec) when from 25% to 75% of the FVC volume is measured *These measures of lung capacity are reduced (with high residual volumes) by conditions such as: -bronchitis (inflammation, excessive mucus plugging small airways) -emphysema (loss of normal lung elasticity, airway collapse) -asthma (bronchial smooth muscle contractions, airway constriction) *chronic obstructive pulmonary diseases (COPD) can result in: -hypoxemia (low blood oxygen) -hypercapnia (high blood carbon dioxide) - hypoxia-induced increase in pulmonary vascular resistance (next week) -estimation of residual volume (RV): *test subject performs a forced expiration then *breaths 10% helium from a source with fixed volume *the helium will be diluted with the non-helium containing air in the residual volume *the decreased helium concentration reflects the size of the residual volume -restrictive lung disorders (interstitial lung disease) *total lung capacity reduced * FEV1/FEV can be increased Special Circulations cardiac circulation -coronary blood flow reserve: blood flow to the heart can increase about 4-5 fold during exercise *very little capacity for glycolysis; oxygen demand must be met *metabolic products such as adenosine act as local vasodilators *NO from endothelial cells also supports vasodilation -reduced coronary blood flow during systole *due to compression of small coronary blood vessels *complicates heart disease *coronary vascular disease -collateral vessels: allows some adaptation for artery blockage -relationship between cardiac output and oxygen use *stroke work: work done by the heart in moving blood can be estimated as SW = SV x MAP SV=stroke volume MAP=mean arterial pressure *stroke work is only 5-20% of the total energy needs of the heart -example: during isovolumetric contraction large amounts of energy is consumed but there is no work done moving blood *almost all heart energy comes from oxidative metabolism, so total energy demand closely corresponds to the rate of oxygen use *changes in energy demand and blood flow can be estimated as the product of the heart rate and the arterial pressure cerebral circulation -good autoregulation of blood flow at blood pressures from 55-155 mm Hg *CO2 and K+ trigger dilation of blood vessels *involves NO from neurons and endothelial cells small intestine circulation - during digestion, blood flow increases in proportion to metabolic demand - the vasodilators adenosine and NO are released during absorption of nutrients - sympathetic stimulation of vasoconstriction greatly reduces blood flow and nutrient absorption during exercise hepatic circulation - both an arterial blood supply and the hepatic portal vein *blood from both sources mixes in the capillaries *local control of arterial blood flow (reciprocal matching) in response to changes in portal blood flow -capillaries endothelial cells have gaps; very leaky -liver vasoconstriction following sympathetic stimulation can shift a significant volume of blood out of the liver skeletal muscle circulation -blood flow can increase about 20 fold in response to exercise -some vasodilation due to beta adrenergic receptors, but most is in response to local metabolic demand (autoregulation) -vasoconstriction is possible in response to blood loss and baroreceptor response to activate sympathetic stimulation dermal circulation -specialized for temperature regulation *in warm climates, increased blood flow allows heat to be lost from the body -arteriovenous anastomoses; hands, feet, parts of the head *in hands and feet, reduced adrenergic stimulation results in vasodilation *other regions of skin has cholinergic sympathetic nerves that mediate both sweating and an active vasodilation, "cutaneous active vasodilator system" *Rhoades says active vasodilation involves the vasodilator bradykinin, but this might not be the case "In vivo mechanisms of cutaneous vasodilation and vasoconstriction in humans during thermoregulatory challenges" http://jap.physiology.org/cgi/content/full/100/5/1709 *blood vessels also dilate when skin is warmed *in cold, adrenergic sympathetic nerves cause vasoconstriction that limits blood flow to the skin to minimize heat loss -if skin gets too cold, vessels eventually dilate and blood flow protects skin from damage Lung Ventilation Respiration: oxygen to tissues and carbon dioxide removed from tissues - gas exchange: dynamic range of about 20x * pulmonary capillaries: atmosphere - blood exchange * systemic capillaries: blood - tissue exchange - cellular respiration *metabolic reactions that produce carbon dioxide *oxygen use as electron acceptor for oxidative phosphorylation respiratory system structure and function -needed: a large surface area for gas exchange between air and blood -made possible by highly branching vascular and airway trees -conducting zone of airway tree *branching bronchi and bronchioles and terminal bronchioles *initial branches with cartilage support against collapse *smaller branches supported by elasticity of lung tissue *the bronchial circulation originates from the descending aorta -not involved in gas exchange while passing through the conducting zone *air is warmed and humidified *dust and bacteria removed -ciliated epithelium pushes mucus and debris to the pharynx - respiratory zone of airway tree * respiratory bronchioles with some attached alveoli * termini: alveolar ducts between dense collections of alveoli *ventilation function: movement of fresh air into and out of alveoli *alveolar surface area is about 75 m2 *alveoli are closely associated with the pulmonary circulation -70-80% of the alveolar surface is covered by capillaries *oxygen and carbon dioxide diffuse between the capillaries and the alveoli -distance is as small as 0.5 m *blood flow through pulmonary capillaries is relatively fast -typically several fold faster that in systemic capillaries Breathing: pressure changes and air flows -contraction of the diaphragm expands the volume of the thoracic cavity *normal breath: 1-2 cm movement of the diaphragm *deep breath (forced inspiration): movement as large as 10-12 cm -rib muscles can contribute to expanded volume of the thoracic cavity -abdominal wall expiratory muscles contract during forced expiration *this forces the relaxed diaphragm further into the thoracic cavity -relationship between thoracic cavity volume and lung volume *increased thoracic cavity volume reduces pressure in the pleural space - pleural space contains fluid between the lungs and the chest wall - pleural space pressure is slightly below atmospheric pressure except during forced expiration -negative pleural space pressure is generated by tension from the lungs and the chest wall both pulling away from the pleural space *the lungs expand against the reduced pressure in the pleural space -transpulmonary pressure: alveolar pressure minus the pleural space pressure *increased transpulmonary pressure when the lungs are inflating -transairway pressure: airway pressure minus the pleural space pressure * during forced expiration pleural space pressure becomes positive, airway pressure also goes higher limiting airway collapse Gas pressures *typical atmospheric pressure is about 760 mm Hg -also called barometric pressure *the total pressure of a mixed gas is the sum of the individual partial pressures *the partial pressure of oxygen in dry air is (760 x 0.21) = 160 mm Hg = PO2 - 21% of air is oxygen *in the humidified and warm air in lungs PH2O = 47 mm Hg *air pressure gradients that move air into and out of lungs are small *these pressures are often expressed as cm H2O - 1 mm Hg = 1.36 cm H2O *inspiration: alveolar pressure -2 cm H2O relative to atmospheric pressure *expiration: alveolar pressure +3 cm H2O relative to atmospheric pressure pneumothorax *lung or chest wall puncture allows air to enter the pleural space *pleural space attains atmospheric pressure *the lung will collapse *the mediastinal membrane restricts the collapse to one lung Pressure changes during the breathing cycle -inspiration *at start: -respiratory muscles are relaxed -alveolar pressure is zero (atmospheric pressure) -air flow is zero - pleural space pressure = -5 cm H2O - transpulmonary pressure = 5 (distending pressure keeping lungs inflated) *diaphragm contraction reduces pleural pressure towards -8 cm H2O -as transpulmonary pressure increases the lungs inflate -alveolar pressure becomes negative -air moves into the lungs according to the mouth-alveolar pressure gradient *at end alveolar pressure equals atmospheric pressure and air flow stops -expiration *the diaphragm relaxes and pleural pressure increases (less negative) *as transpulmonary pressure decreases the lungs deflate *alveolar volume decreases and pressure increases above atmospheric pressure *air flows out of the lungs until alveolar pressure equals atmospheric pressure *resistance to air flow is greater during expiration -alveolar pressure increase greater than the decrease during inspiration -expiration lasts slightly longer than inspiration Alveolar ventilation (VA) L/min - dead space volume (VD): *with a 500 ml inhaled breath about 150 ml is in the conducting airways -anatomic dead space volume *there can also be alveolar air that does not participate in gas exchange -for example, due to poor capillary supply to alveoli -alveolar dead space volume -physiological dead space volume = anatomic + alveolar dead space volumes - VA = (VT - VD) x f volume of fresh air reaching functioning alveoli per minute * f = breaths per minute - increased rate of breathing (f) is not very effective for increasing VA if VT decreases * dead volume becomes a larger fraction of VT - increased depth of breathing is more effective for increasing VA * dead volume becomes a smaller fraction of inspired air - a high rate of alveolar ventilation helps remove carbon dioxide from the body and deliver oxygen (next week, gas transfer) mechanical properties of the lungs -elasticity of the lungs is important for their easy of expansion when transpulmonary pressure increases and their return (recoil) to resting size when transpulmonary pressure decreases -distensibilty: the ease with which lungs inflate *distensibility and recoil are inversely related *if lungs are too easy to inflate then there will not be much elastic recoil *lung elasticity depends on matrix proteins like elastin -lung compliance *the change in lung volume (V) corresponding to a change in pleural pressure (P) * V / P = lung compliance * lung compliance is smaller at higher lung filling volumes * restrictive lung disease causes low compliance and low distensibility *more muscle work required (lower pleural pressure) to get normal lung inflation *in the obstructive disorder emphysema *elasticity is lost and there is high compliance (easy to inflate) *but little elastic recoil and there is usually a large residual air volume -chest wall elasticity *at about 70% of maximum lung capacity chest wall recoil is zero *below 70% chest wall recoil is directed outward -outward recoil is maximal at the lung residual volume *above 70% of maximum lung capacity chest wall recoil is directed inward -inward recoil is maximal at total lung capacity surface tension - surface tension of water is a force arising from the preference of water molecules for each other rather than for air, this force resists lung inflation - surface tension at the lung surface area acts as an elastic force in parallel with lung tissue -the elastic force due to surface tension is about two thirds of the total -surface tension makes the lung significantly less compliant (less distensible) -pulmonary surfactant *reduces the alveolar surface tension *produced by Type II alveolar cells *surfactant contains phospholipid that spreads over the air-water interface -dipalmitoylphosphatidylcholine (DPPC) *premature babies have too little surfactant, higher surface tension and difficulty keeping alveoli inflated (infant respiratory distress syndrome) airway resistance - due to either turbulent air flow during high rates of air movement in the large airways or laminar flow - air flow (V) is *proportional to the pressure difference driving flow (mouth to alveoli, Pmouth – PA) *inversely proportional to the resistance (airway resistance, Raw) * V = (Pmouth – PA)/Raw * Raw = (Pmouth – PA)/ V - airway resistance is fairly constant during normal breathing (at rest) *not constant during vigorous exercise and forced expiration *when lung volume is reduced (in association with forced expiration) resistance is high due to compression of airways *during forced expiration pleural pressure can be 30 cm H2O -the high pleural pressure can cause airway constriction -regulation of airway resistance *bronchial constriction -parasympathetic stimulation (mAChR, release of intracellular Ca++) -local factors such as histamine (from mast cells during allergy) *bronchial dilation -circulating epinephrine acting on 2 receptors -high airway carbon dioxide work of breathing - at rest the energy for breathing might be 5% of total energy demand - during exercise breathing might account for 20% of total energy demand - in restrictive lung disease, greater work is required to produce a normal tidal volume *to minimize this work, breaths tend to be rapid and shallow - in obstructive lung disease, patients tend to take deeper breaths than normal *this is a way of reducing lung resistance * greater energy is expended due to the higher resistance Gas exchange between alveolar air and pulmonary capillaries -Differences in oxygen concentrations between the atmosphere and alveoli: -in the humidified and warm air in lungs PH2O = 47 mm Hg (saturation at body temperature) *increased water vapor of inspired air dilutes the oxygen from 160 to 150 mm Hg -mixing inspired air with air in the functional reserve capacity * the functional reserve capacity is about 2.3 liters * less than 0.5 L of fresh air reaches the alveoli with each breath (at rest) *the fresh air is diluted with existing old air in the lungs, giving a typical alveolar partial pressure of oxygen of about 102 mm Hg -net diffusion of oxygen and carbon dioxide *depends on concentration differences, net diffusion from high to low concentration *concentrations of gases are proportional to their partial pressures *diffusion of oxygen from air in alveoli to blood -typical partial pressure of oxygen in alveoli: 102 mm Hg -typical partial pressure of oxygen in pulmonary artery blood: 40 mm Hg -at rest, net oxygen diffusion into blood is complete before blood exits the capillaries *a greater amount of oxygen transfer to blood is possible if capillary blood flow increases *during exercise capillary blood flow increases, but oxygen diffusion is still fast enough so that the alveolar and blood partial pressures of oxygen have time to equilibrate *diffusion of carbon dioxide from blood to air in alveoli -typical partial pressure of carbon dioxide in alveoli: 40 mm Hg -typical partial pressure of carbon dioxide in pulmonary artery blood: 46 mm Hg venous admixture -the partial pressure of oxygen in arterial blood of the systemic circulation is lower than the partial pressure of oxygen leaving the pulmonary capillaries -some deoxygenated venous blood from the bronchial circulation is mixed with oxygenated venous blood from the pulmonary circulation -diffusing capacity *the rate of gas diffusion in fluid depends on: -the solubility of the gas in the fluid -the surface area available for diffusion *carbon dioxide is about 20-fold more soluble in water than is oxygen -to achieve the same diffusion rates, larger partial pressure gradients of oxygen are needed than of carbon dioxide * diffusing capacities at rest -oxygen: about 20 ml/min/mm Hg -carbon dioxide: about 400 ml/min/mm Hg * diffusing capacities during exercise -oxygen: maximum of about 65 ml/min/mm Hg -carbon dioxide: about 1250 ml/min/mm Hg -basis of increased diffusing capacity: *all pulmonary capillaries open *dilation of capillaries (increased surface area for diffusion) *better ventilation-perfusion ratio (below) gas exchange in systemic capillaries *interstitial fluid partial pressure of oxygen averages about 40 mm Hg *the partial pressure of oxygen in blood arriving at systemic capillaries averages about 95 mm Hg *if tissue oxygen consumption increases, a greater rate of blood flow can maintain the oxygen level *cells need a partial pressure of oxygen of about 1-3 mm Hg; probably average >20 mm Hg *the partial pressure of oxygen in blood leaving systemic capillaries averages about 40 mm Hg *tissue partial pressure of carbon dioxide is about 45 mm Hg *arterial blood arriving at systemic capillaries: PCO2 is about 40 mm Hg *venous blood leaving systemic capillaries: PCO2 is about 45 mm Hg