Week 3 Reading - Nutrition, Metabolism, and Body Temperature
Document Details
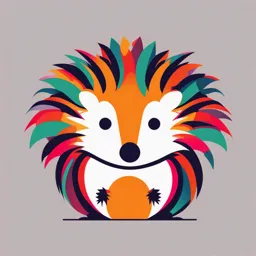
Uploaded by InvinciblePedalSteelGuitar
Tags
Summary
This document provides an overview of nutrition, metabolism, and body temperature regulation. It discusses essential nutrients, dietary sources, uses in the body, and recommended amounts of carbohydrates, lipids, and proteins, as well as vitamins and minerals. The text also explains the role of kilocalories in energy and discusses how different foods impact the body. It highlights the importance of a balanced diet and offers practical insights for maintaining a healthy lifestyle.
Full Transcript
17 C H A P T E R Nutrition, Metabolism, and body Temperature Regulation LEARN TO PREDICT Sadie and David loved it when their mothers planned a picnic at the park. They enjoyed running and playing on the jungle gym. Today, Sadie begged her mom to pack a snack of chocolate chip cookies and grape soda....
17 C H A P T E R Nutrition, Metabolism, and body Temperature Regulation LEARN TO PREDICT Sadie and David loved it when their mothers planned a picnic at the park. They enjoyed running and playing on the jungle gym. Today, Sadie begged her mom to pack a snack of chocolate chip cookies and grape soda. This snack was packed with calories that would give the children lots of energy, but otherwise, it had very little nutritional value. Sadie’s mom explained that a snack of fruits, whole-wheat crackers, and water would be much better for everyone. After reading this chapter, predict the outcome if Sadie’s mom allowed them to bring Sadie’s suggested snack, which was full of calories, rather than the snack her mother suggested they take to the park. 17.1 NUTRITION learning Outcomes After reading this section, you should be able to A. Define nutrition, essential nutrient, and kilocalorie. B. For carbohydrates, lipids, and proteins, describe their dietary sources, their uses in the body, and the daily recommended amounts of each in the diet. C. List the common vitamins and minerals, and give a function for each. When choosing from a menu or selecting food to prepare, we are often more concerned with the food’s taste than with its nutritional value. However, knowing about nutrition is important because the food we eat provides us with the energy and the building blocks necessary to synthesize new molecules. What happens if we don’t obtain enough vitamins, or if we eat too much sugar and fat? Health claims about foods and food supplements bombard us every day. Which ones are ridiculous, and which ones have merit? A basic understanding of nutrition can help us answer these and other questions so that we can develop a healthful diet. It can also allow us to know which questions currently do not have good answers. Nutrition (noo-trish′ ŭn; to nourish) is the process by which food is taken into and used by the body; it includes digestion, absorption, transport, and metabolism. Nutrition is also the study of food and drink requirements for normal body function. 476 Module 12 Digestive System Nutrients Nutrients are the chemicals taken into the body that provide energy and building blocks for new molecules. Some substances in food are not nutrients but provide bulk (fiber) in the diet. Nutrients can be divided into six major classes: carbohydrates, lipids, proteins, vitamins, minerals, and water. Carbohydrates, lipids, and proteins are the major organic nutrients and are broken down by enzymes into their individual subunits during digestion. Subsequently, many of these subunits are broken down further to supply energy, whereas other subunits are used as building blocks for making new carbohydrates, lipids, and proteins. Vitamins, minerals, and water are taken into the body without being broken down. They are essential participants in the chemical reactions necessary to maintain life. The body requires some nutrients in fairly substantial quantities and others, called trace elements, in only minute amounts. Nutrition, Metabolism, and Body Temperature Regulation Essential nutrients are nutrients that must be ingested because the body cannot manufacture them—or it cannot manufacture them in adequate amounts. The essential nutrients include certain amino acids, certain fatty acids, most vitamins, minerals, water, and some carbohydrates. The term essential does not mean that the body requires only the essential nutrients. Other nutrients are necessary, but if they are not ingested, they can be synthesized from the essential nutrients. Most of this synthesis takes place in the liver, which has a remarkable ability to transform and manufacture molecules. A balanced diet consists of enough nutrients in the correct proportions to support normal body functions. Every 5 years, the U.S. Department of Health and Human Services (HHS) and the U.S. Department of Agriculture (USDA) jointly make recommendations on what Americans should eat to be healthy. The latest recommendations, the Dietary Guidelines for Americans, 2010, were published in January 2011. In light of the increasing problem of obesity in the United States, the latest recommendations focus on two concepts: (1) balancing calorie intake to obtain and maintain a healthy weight, and (2) increasing consumption of healthy, nutrient-rich foods. In June 2011, the USDA also introduced MyPlate, a new food icon to replace the former food guide icon, called MyPyramid. MyPlate (figure 17.1) is a simple visual reminder of how to build a healthy meal. The MyPlate icon shows a plate and glass with portions representing foods from the fruits, vegetables, grains, proteins, and dairy food groups. To emphasize the importance of making healthy food choices, half the plate is fruits and vegetables. In addition to the MyPlate icon, the USDA also launched ChooseMyPlate.gov, a website that includes information on how to make healthy dietary choices. The importance of a healthy diet has been proposed as a key to a healthy life. Two studies completed in 2000 compared the eating habits of 51,529 men and 67,272 women to the government’s Healthy 477 Eating Index, a measure of how well a diet conforms to recommended dietary guidelines. Results from these studies demonstrate that eating a healthy diet does provide certain health benefits. People who ate the best, according to the index, were compared to those who ate the worst. Men who ate best had a 28% reduction in heart disease and an 11% decrease in chronic diseases compared to men who ate worst. Women who ate best had a 14% reduction in heart disease but no significant decrease in chronic diseases compared to women who ate worst. There was no significant difference in cancer rates between the men and women who ate best compared to those who ate worst. Kilocalories The energy the body uses is stored within the chemical bonds of certain nutrients. A calorie (kal′ ō-rē; heat) (cal) is the amount of energy (heat) necessary to raise the temperature of 1 gram (g) of water 1°C. A kilocalorie (kil′ ō-kal-ō-rē) (kcal) is 1000 cal and is used to express the larger amounts of energy supplied by foods and released through metabolism. For example, one slice of white bread contains about 75 kcal, 1 cup of whole milk contains 150 kcal, a banana contains 100 kcal, a hot dog contains 170 kcal (not counting the bun and dressings), a McDonald’s Big Mac has 540 kcal, and a soft drink adds another 145 kcal. For each gram of carbohydrate or protein metabolized by the body, about 4 kcal of energy are released. Fats contain more energy per unit of weight than carbohydrates and proteins, and they yield about 9 kcal/g. Table 17.1 lists the kilocalories supplied by some typical foods. A typical diet in the United States consists of 50–60% carbohydrates, 35–45% fats, and 10–15% proteins. Table 17.1 also lists the carbohydrate, fat, and protein composition of some foods. A kilocalorie is often called a Calorie (with a capital C). Unfortunately, this usage has resulted in confusion of the term calorie (with a lowercase c) with Calorie (with a capital C). It is common practice on food labels to use the term calorie when Calorie (kilocalorie) is the proper term. Carbohydrates Sources in the Diet Figure 17.1 MyPlate The MyPlate icon provides a visual reminder for making choices at mealtime, by selecting healthy foods from five food groups. Half the meal should be fruits and vegetables. Source: U.S. Department of Agriculture. Carbohydrates (kar-bō-hı̄′ drātz) include monosaccharides, disaccharides, and polysaccharides (see chapter 2). Although most of the carbohydrates we ingest are derived from plants, lactose is derived from animals. The most common monosaccharides in the diet are glucose and fructose. Plants capture energy from sunlight and use that energy to produce glucose, which can be found in vegetables, fruits, molasses, honey, and syrup. Fructose is most often derived from fruits and berries. The disaccharide sucrose (table sugar) is what most people think of when they use the term sugar. Sucrose consists of one glucose and one fructose molecule joined together, and its principal sources are sugarcane and sugar beets. Maltose (malt sugar), derived from germinating cereals, is a combination of two glucose molecules, and lactose (milk sugar) consists of one glucose molecule and one galactose molecule. Complex carbohydrates are large polysaccharides, which are composed of long chains of glucose (see figure 2.11). Examples are starch, glycogen, and cellulose, which differ from one another in the arrangement of the glucose molecules and the structure of the chemical bonds holding them together. 478 Chapter 17 Table 17.1 Food Composition Food Quantity Food Energy (kcal) Carbohydrate (g) Fat (g) Protein (g) 8 Dairy Products Whole milk (3.3% fat) 1 cup 150 11 8 Low-fat milk (2% fat) 1 cup 120 12 5 8 Butter 1T 100 — 12 — Grain Bread, white enriched 1 slice 75 24 1 2 Bread, whole-wheat 1 slice 65 14 1 3 Apple 1 80 20 1 — Banana 1 100 25 — 1 Orange 1 65 16 — 1 4 Fruit Vegetables Corn, canned 1 cup 140 33 1 Peas, canned 1 cup 150 29 1 8 Lettuce 1 cup 5 2 — — Celery 1 cup 20 5 — 1 Potato, baked 1 large 145 33 — 4 23 Meat, Fish, and Poultry Lean ground beef (10% fat) 3 oz 185 — 10 Shrimp, french fried 3 oz 190 9 9 17 Tuna, canned 3 oz 170 — 7 24 Chicken breast, fried 3 oz 160 1 5 26 Bacon 2 slices 85 — 8 4 Hot dog 1 170 1 15 7 McDonald’s Egg McMuffin 1 300 30 12 18 McDonald’s Big Mac 1 540 45 29 25 Taco Bell Beef Burrito Supreme 1 420 53 15 17 Arby’s roast beef, regular 1 360 37 14 22 Pizza Hut Super Supreme 1 slice 245 29 17 14 Long John Silver’s fish 2 pieces 520 32 34 24 Dairy Queen Oreo Cookie Blizzard, medium 1 680 100 25 14 Fast Foods Desserts Chocolate chip cookie 1 50 7 2 1 Apple pie 1 piece 135 49 14 3 Soft ice cream 1 cup 377 38 23 7 — Beverage Cola soft drink 12 oz 145 37 — Beer 12 oz 144 13 — — Wine 321 oz 73 2 — — Hard liquor (86 proof) 121 oz 105 — — — Miscellaneous Egg 1 80 1 6 6 Mayonnaise 1T 100 — 11 — Sugar 1T 45 12 — — Nutrition, Metabolism, and Body Temperature Regulation Starch is an energy-storage molecule in plants and is found primarily in vegetables, fruits, and grains. Glycogen is an energystorage molecule in animals and is located primarily in muscle and in the liver. Cellulose forms plant cell walls. Uses of Carbohydrates in the Body During digestion, polysaccharides and disaccharides are split into monosaccharides, which are absorbed into the blood (see chapter 16). Humans have enzymes that can break the bonds between the glucose molecules of starch and glycogen, but they do not have the enzymes necessary to digest cellulose. Therefore, it is important to thoroughly cook or chew plant matter. Cooking and chewing break down the plant cell walls and expose the starches contained inside the cells to digestive enzymes. The undigested cellulose provides fiber, or “roughage,” which increases the bulk of feces, making it easier to defecate. Fructose and other monosaccharides absorbed into the blood are converted into glucose by the liver. Glucose, whether absorbed directly from the digestive tract or synthesized by the liver, is an energy source used to produce ATP. Because the brain relies almost entirely on glucose for its energy, the body carefully regulates blood glucose levels. If an excess amount of glucose is present in the diet, the glucose is used to make glycogen, which is stored in muscle and in the liver. Glycogen can be rapidly converted back to glucose when energy is needed. Because cells can store only a limited amount of glycogen, additional glucose that is ingested is converted into lipids for long-term storage in adipose tissue. In addition to being used as an energy source, sugars form part of deoxyribonucleic acid (DNA), ribonucleic acid (RNA), and ATP molecules. Sugars also combine with proteins to form glycoproteins, some of which function as receptor molecules on the outer surface of the cell membrane. Recommended Consumption of Carbohydrates According to the Dietary Guidelines Advisory Committee, the Acceptable Macronutrient Distribution Range (AMDR) for carbohydrates is 45–65% of total kilocalories. Although a minimum level of carbohydrates is not known, it is assumed that amounts of 100 g or less per day result in overuse of the body’s proteins and lipids for energy sources. Because muscles are primarily protein, the use of proteins for energy can result in the breakdown of muscle tissue. The extensive use of lipids as an energy source can result in acidosis (see chapter 18). Complex carbohydrates are recommended in the diet because many starchy foods contain other valuable nutrients, such as vitamins and minerals, and because the slower rate of digestion and absorption of complex carbohydrates does not result in large increases and decreases in blood glucose level, as the consumption of large amounts of simple sugars does. Eating whole grains also helps a person meet the recommended fiber intake (25 g/day for females and 38 g/day for males). Foods containing large amounts of simple sugars, such as soft drinks and candy, are rich in carbohydrates but have few other nutrients. For example, a typical soft drink is mostly sucrose, containing 9 teaspoons of sugar per 12-oz container. In excess, the consumption of these kinds of foods usually results in obesity and tooth decay. 479 Lipids Sources in the Diet Lipids (lip′ idz) include triglycerides, steroids, phospholipids, and fat-soluble vitamins. Triglycerides (trı̄-glis′ er-ı̄dz), also called triacylglycerols (tr ı̄-as′ il-glis′ er-olz), are the most common type of lipid in the diet, accounting for about 95% of the total lipid intake. Triglyceride molecules consist of three fatty acids bound to one glycerol molecule (see figure 2.12). Triglycerides are often referred to as fats. If the fat is a liquid at room temperature, it is referred to as an oil. Fats are saturated if their fatty acids have only single covalent bonds between carbon atoms and unsaturated if they have one or more double bonds (see figure 2.13). Monounsaturated fats have one double bond, and polyunsaturated fats have two or more double bonds. Saturated fats are found in meat, dairy products, eggs, nuts, coconut oil, and palm oil (table 17.1). Monounsaturated fats include olive and peanut oils; polyunsaturated fats are found in fish, safflower, sunflower, and corn oils. Solid fats, mainly shortening and margarine, work better than liquid oils in preparing some foods, such as pastries. Polyunsaturated vegetable oils can be changed from a liquid to a solid by making them more saturated—that is, by decreasing the number of double covalent bonds in their polyunsaturated fatty acids. To saturate an unsaturated oil, the oil can be hydrogenated, which means that hydrogen gas is bubbled through the oil, producing a change in molecular shape that solidifies the oil. The more saturated the product, the harder it becomes at room temperature. These processed fats are usually referred to as trans fats. Processed foods and oils account for most of the trans fats in the American diet, although some trans fats occur naturally in food from animal sources. Trans fatty acids raise the concentration of low-density lipoproteins and lower the concentration of highdensity lipoproteins in the blood (see chapter 16). These changes are associated with a greater risk of cardiovascular disease. In 2006, the Food and Drug Administration (FDA) required that food labels include a detailed list of the amounts of saturated and trans fats, allowing the consumer to make better dietary choices. The remaining 5% of ingested lipids include steroids and phospholipids. Cholesterol (kō-les′ter-ol) is a steroid (see chapter 2) found in high concentrations in the brain, the liver, and egg yolks, but it is also present in whole milk, cheese, butter, and meats. Cholesterol is not found in plants. Phospholipids, such as lecithin (les′i-thin; lekithos, egg yolk), are major components of cell membranes and are found in a variety of foods. A good source of lecithin is egg yolks. Uses of Lipids in the Body Triglycerides are an important source of energy that can be used to produce ATP. A gram of triglyceride delivers over twice as many calories as does a gram of carbohydrate or protein. Some cells, such as skeletal muscle cells, derive most of their energy from triglycerides. Ingested triglyceride molecules not immediately used are stored in adipose tissue or in the liver. When energy is required, the stored triglycerides are broken down, and the fatty acids are released into the blood. The fatty acids can be taken up and used by various tissues. In addition to storing energy, adipose tissue surrounds, pads, and protects organs. Adipose tissue located under the skin is an insulator, which helps reduce heat loss. 480 Chapter 17 CLINICAL IMPACT The essential fatty acids are used to synthesize molecules that affect blood clotting. Linoleic acid can be converted to arachidonic (ă-rak-i-don′ik; arakis) acid, which is used to produce prostaglandins that increase blood clotting. Alpha-linolenic acid can be converted to eicosapentaenoic (ı̄′kō-să-pen-tă-nō′ik) acid (ePa), which is used to produce pros- Fatty Acids and Blood Clotting taglandins that decrease blood clotting. Normally, most prostaglandins are synthesized from linoleic acid because it is more plentiful in the diet. However, individuals who consume foods rich in EPA, such as herring, salmon, tuna, and sardines, increase the synthesis of prostaglandins from EPA. People who eat these fish two or more times per week have a lower risk Cholesterol is an important molecule with many functions in the body. It is obtained in food, or it can be manufactured by the liver and most other tissues. Cholesterol is a component of the cell membrane, and it can be modified to form other useful molecules, such as bile salts and steroid hormones. Bile salts emulsify fats, which is important for fat digestion and absorption (see chapter 16). Steroid hormones include the sex hormones estrogen, progesterone, and testosterone, which regulate the reproductive system. Eicosanoids, which are derived from fatty acids, are involved in inflammation, tissue repair, smooth muscle contraction, and other functions. Phospholipids (see chapter 2) are part of the cell membrane and are used to construct myelin sheaths around the axons of nerve cells. Lecithin is found in bile and helps emulsify fats. Recommended Consumption of Lipids The AMDR for fats is 20–35% for adults, 25–35% for children and adolescents 4 to 18 years of age, and 30–35% for children 2 to 3 years of age. Saturated fats should be no more than 10% of total kilocalories, or as low as possible. Most dietary fat should come from sources of polyunsaturated and monounsaturated fats. Cholesterol should be limited to 300 mg (the amount in one egg yolk) or less per day, and trans fat consumption should be as low as possible. These guidelines reflect the belief that excess amounts of fats, especially saturated fats, trans fats, and cholesterol, contribute to cardiovascular disease. The typical American diet derives 35–45% of its kilocalories from fats, indicating that most Americans need to reduce their fat consumption. See table 17.1 for a sampling of the fat composition in foods. If a person does not consume enough fats, the body can synthesize fats from carbohydrates and proteins. Linoleic (lin-ō-lē′ ik; linum, flax + oleum, oil) acid and alpha linolenic (lin-ō-len′ ik) acid are called essential fatty acids because the body cannot synthesize them, so they must be ingested. They are found in plant oils, such as canola or soybean oil. Proteins Sources in the Diet Proteins (prō′tēnz) are chains of amino acids (see figure 2.16). They are found in most of the plant and animal products we eat (see table 17.1). Proteins in the body are constructed of 20 different of heart attack than those who don’t, probably because of reduced blood clotting. Although EPA can be obtained using fish oil supplements, this is not currently recommended because fish oil supplements contain high amounts of cholesterol, vitamins A and D, and uncommon fatty acids, all of which can cause health problems when taken in large amounts. kinds of amino acids, which are divided into two groups: essential and nonessential amino acids. The body cannot synthesize essential amino acids, so they must be obtained in the diet. The nine essential amino acids are histidine, isoleucine, leucine, lysine, methionine, phenylalanine, threonine, tryptophan, and valine. Nonessential amino acids are necessary to construct our proteins but do not necessarily need to be ingested, since they can be synthesized from the essential amino acids. A complete protein food contains all nine essential amino acids in the needed proportions, whereas an incomplete protein food does not. Animal proteins tend to be complete proteins, whereas plant proteins tend to be incomplete. Examples of complete proteins are red meat, fish, poultry, milk, cheese, and eggs. Examples of incomplete proteins are leafy green vegetables, grains, and legumes (peas and beans). If two incomplete proteins, such as rice and beans, are ingested, each can provide amino acids lacking in the other. Thus, a vegetarian diet, if balanced correctly, provides all the essential amino acids. Uses of Proteins in the Body Proteins perform numerous functions in the human body, as the following examples illustrate. Collagen provides structural strength in connective tissue, as does keratin in the skin. The combination of actin and myosin makes muscle contraction possible. Enzymes are responsible for regulating the rate of chemical reactions in the body, and protein hormones regulate many physiological processes (see chapter 10). Proteins in the blood act as clotting factors, transport molecules, and buffers (which limit changes in pH). Proteins also function as ion channels, carrier molecules, and receptor molecules in the cell membrane. Antibodies, lymphokines, and complement are all proteins that function in the immune system. Proteins can also be used as a source of energy, yielding approximately the same amount of energy as that derived from carbohydrates. If excess proteins are ingested, the body can use the energy from the proteins to produce glycogen and lipid molecules, which can be stored. When protein intake is adequate in a healthy adult, the synthesis and breakdown of proteins occur at the same rate. Recommended Consumption of Proteins The AMDR for protein is 10–35% of total kilocalories. See table 17.1 for a sampling of the protein composition in foods. Nutrition, Metabolism, and Body Temperature Regulation CLINICAL IMPACT Free radicals are molecules, produced as part of normal metabolism, that are missing an electron. Free radicals can replace the missing electron by taking an electron from cell molecules, such as lipids, proteins, or DNA, resulting in damage to the cell. Damage from free radicals may contribute to aging and certain diseases, such as atherosclerosis and cancer. 481 Free Radicals and Antioxidants The loss of an electron from a molecule is called oxidation. antioxidants are substances that prevent oxidation of cell components by donating an electron to free radicals. Examples of antioxidants are beta carotene (provitamin A), vitamin C, and vitamin E. Many studies have attempted to determine whether taking large doses of antioxidants is beneficial. Among scientists Vitamins Vitamins (vı̄t′ ă-minz; life-giving chemicals) are organic molecules that exist in minute quantities in food and are essential to normal metabolism (table 17.2). Essential vitamins cannot be produced by the body and must be obtained through the diet. Because no single food item or nutrient class provides all the essential vitamins, it is necessary to maintain a balanced diet by eating a variety of foods. The absence of an essential vitamin in the diet can result in a specific deficiency disease. A few vitamins, such as vitamin K, are produced by intestinal bacteria, and a few others can be formed by the body from substances called provitamins. A provitamin is a part of a vitamin that the body can assemble or modify into a functional vitamin. Beta carotene is an example of a provitamin that can be modified by the body to form vitamin A. The other provitamins are 7-dehydrocholesterol (dē-hı̄′ drō-kō-les′ ter-ol), which can be converted to vitamin D, and tryptophan (trip′ tō-fan), which can be converted to niacin. Vitamins are not broken down by catabolism but are used by the body in their original or slightly modified forms. After the chemical structure of a vitamin is destroyed, its function is lost. The chemical structure of many vitamins is destroyed by heat, as when food is overcooked. Most vitamins function as coenzymes, which combine with enzymes to make the enzymes functional (see chapter 2). Vitamins B2 and B3, biotin (bı̄′ ō-tin), and pantothenic (pantō-then′ ik) acid are critical for some of the chemical reactions involved in the production of ATP. Folate (fō′ lāt) and vitamin B12 are involved in nucleic acid synthesis. Vitamins A, B1, B6, B12, C, and D are necessary for growth. Vitamin K is necessary for the synthesis of proteins involved in blood clotting (table 17.2). Predict 2 Predict what would happen if vitamins were broken down during the process of digestion rather than being absorbed intact into the circulation. Vitamins are either fat-soluble or water-soluble. Fat-soluble vitamins, such as vitamins A, D, E, and K, are absorbed from the intestine along with lipids. Some of them can be stored in the body for a long time. Because they can be stored, these vitamins can accumulate in the body to the point of toxicity. Water-soluble establishing the RDAs, the best evidence presently available does not support the claim that large doses of antioxidants can prevent chronic disease or otherwise improve health. On the other hand, the amount of antioxidants normally found in a balanced diet (including fruits and vegetables rich in antioxidants), combined with the complex mix of other chemicals found in food, can be beneficial. vitamins, such as the B vitamins and vitamin C, are absorbed with water from the intestinal tract and typically remain in the body only a short time before being excreted in the urine. Vitamins were first identified at the beginning of the twentieth century. They were found to be associated with certain foods that were known to protect people from diseases such as rickets and beriberi. In 1941, the first Food and Nutrition Board established the Recommended Dietary Allowances (RDAs), which are the nutrient intakes that are sufficient to meet the needs of nearly all people in certain age and gender groups. RDAs were established for different-aged males and females, starting with infants and continuing on to adults. RDAs were also set for pregnant and lactating women. The RDAs have been reevaluated every 4–5 years and updated, when necessary, on the basis of new information. The RDAs establish a minimum intake of vitamins and minerals that should protect almost everyone (97%) in a given group from diseases caused by vitamin or mineral deficiencies. Although personal requirements can vary, the RDAs are a good benchmark. The farther below the RDAs an individual’s dietary intake is, the more likely that person is to develop a nutritional deficiency. On the other hand, consuming too much of some nutrients can have harmful effects. For example, the long-term ingestion of 3–10 times the RDA for vitamin A can cause bone and muscle pain, skin disorders, hair loss, and an enlarged liver. Consuming 5–10 times the RDA of vitamin D over the long term can result in calcium deposits in the kidneys, heart, and blood vessels, and regularly consuming more than 2 g (which is more than 20 times the RDA) of vitamin C daily can cause stomach inflammation and diarrhea. Minerals Minerals (min′ er-ălz) are inorganic nutrients that are essential for normal metabolic functions. People ingest minerals alone or in combination with organic molecules. They constitute about 4–5% of total body weight and are involved in a number of important functions, such as establishing resting membrane potentials and generating action potentials; adding mechanical strength to bones and teeth; combining with organic molecules; and acting as coenzymes, buffers, or regulators of osmotic pressure. A balanced diet can provide all the necessary minerals, with a few possible 482 Chapter 17 Table 17.2 The Principal Vitamins Vitamin Fat- (F) or Water(W) Soluble A (retinol) Reference Daily Intake (RDI)sa Source Function Symptoms of Deficiency F From provitamin carotene found in yellow and green vegetables; preformed in liver, egg yolk, butter, and milk Necessary for rhodopsin synthesis, normal health of epithelial cells, and bone and tooth growth Rhodopsin deficiency, night blindness, retarded growth, skin disorders, and increased infection risk 900 REb B1 (thiamine) W Yeast, grains, and milk Involved in carbohydrate and amino acid metabolism; necessary for growth Beriberi—muscle weakness (including cardiac muscle), neuritis, and paralysis 1.2 mg B2 (riboflavin) W Green vegetables, liver, wheat germ, milk, and eggs Component of flavin adenine dinucleotide; involved in citric acid cycle Eye disorders and skin cracking, especially at corners of the mouth 1.3 mg B3 (niacin) W Fish, liver, red meat, yeast, grains, peas, beans, and nuts Component of nicotinamide adenine dinucleotide; involved in glycolysis and citric acid cycle Pellagra—diarrhea, dermatitis, and nervous system disorder 16 mg Pantothenic acid W Liver, yeast, green vegetables, grains, and intestinal bacteria Constituent of coenzyme-A; glucose production from lipids and amino acids; steroid hormone synthesis Neuromuscular dysfunction and fatigue 5 mg Biotin W Liver, yeast, eggs, and intestinal bacteria Fatty acid and nucleic acid synthesis; movement of pyruvic acid into citric acid cycle Mental and muscle dysfunction, fatigue, and nausea 30 μg B6 (pyridoxine) W Fish, liver, yeast, tomatoes, and intestinal bacteria Involved in amino acid metabolism Dermatitis, retarded growth, and nausea 1.7 mg Folate W Liver, green leafy vegetables, and intestinal bacteria Nucleic acid synthesis; hematopoiesis; prevents birth defects Macrocytic anemia (enlarged red blood cells) and spina bifida 0.4 mg B12 (cobalamins) W Liver, red meat, milk, and eggs Necessary for red blood cell production, some nucleic acid and amino acid metabolism Pernicious anemia and nervous system disorders 2.4 μg C (ascorbic acid) W Citrus fruit, tomatoes, and green vegetables Collagen synthesis; general protein metabolism Scurvy—defective bone formation and poor wound healing 90 mg D (cholecalciferol, ergosterol) F Fish liver oil, enriched milk, and eggs; provitamin D converted by sunlight to cholecalciferol in the skin Promotes calcium and phosphorus use; normal growth and bone and tooth formation Rickets—poorly developed, weak bones, osteomalacia; bone reabsorption 10 μgc E (tocopherol, tocotrienols) F Wheat germ; cottonseed, palm, and rice oils; grain; liver; and lettuce Prevents oxidation of cell membranes and DNA Hemolysis of red blood cells 15 mg K (phylloquinone) F Alfalfa, liver, spinach, vegetable oils, cabbage, and intestinal bacteria Required for synthesis of a number of clotting factors Excessive bleeding due to retarded blood clotting 120 μg a b c RDIs for people over 4 years of age; IU = international units. Retinol equivalents (RE). 1 retinol equivalent = 1 μg retinol or 6 μg β-carotene. As cholecalciferol. 1 μg cholecalciferol = 40 IU (international units) vitamin D. Nutrition, Metabolism, and Body Temperature Regulation Table 17.3 Important Minerals Mineral Function Symptoms of Deficiency Calcium Bone and teeth formation; blood clotting; muscle activity; and nerve function Spontaneous action potential generation in neurons and tetany 1300 mg Chlorine Blood acid-base balance; hydrochloric acid production in stomach Acid-base imbalance 2.3 gb Chromium Associated with enzymes in glucose metabolism Unknown 35 μg Reference Daily Intake (RDI)sa Cobalt Component of vitamin B12; red blood cell production Anemia Unknown Copper Hemoglobin and melanin production; electrontransport system Anemia and loss of energy 0.9 mg Fluorine Provides extra strength in teeth; prevents dental caries No real pathology 4 mg Iodine Thyroid hormone production; maintenance of normal metabolic rate Goiter and decrease in normal metabolism 150 μg Iron Component of hemoglobin; ATP production in electron-transport system Anemia, decreased oxygen transport, and energy loss 18 mg Magnesium Coenzyme constituent; bone formation; muscle and nerve function Increased nervous system irritability, vasodilation, and arrhythmias 420 mg Manganese Hemoglobin synthesis; growth; activation of several enzymes Tremors and convulsions 2.3 mg Molybdenum Enzyme component Unknown 45 μg Phosphorus Bone and teeth formation; important in energy transfer (ATP); component of nucleic acids Loss of energy and cellular function 1250 mg Potassium Muscle and nerve function Muscle weakness, abnormal electrocardiogram, and alkaline urine 4.7 g Selenium Component of many enzymes Unknown 55 μg Sodium Osmotic pressure regulation; nerve and muscle function Nausea, vomiting, exhaustion, and dizziness 1.5 gb Sulfur Component of hormones, several vitamins, and proteins Unknown Unknown Zinc Component of several enzymes; carbon dioxide transport and metabolism; necessary for protein metabolism Deficient carbon dioxide transport and deficient protein metabolism 11 mg a b 483 RDIs for people over 4 years of age, except for sodium. 3.8 g sodium chloride (table salt) exceptions. For example, individuals suffering from chronic bleeding or women who experience excessive menstrual bleeding may need an iron supplement. Table 17.3 lists some minerals and their functions. Daily Values Daily Values appear on food labels to help consumers plan a healthful diet and to minimize confusion. However, not all possible Daily Values are required to be listed on food labels. Daily Values are based on two other sets of reference values—Reference Daily Intakes and Daily Reference Values: Reference Daily Intakes (RDIs) are based on the 1968 RDAs for certain vitamins and minerals. RDIs have been set for four categories of people: infants, toddlers, people over 4 years of age, and pregnant or lactating women. Generally, the RDIs are set to the highest 1968 RDA value of an age category. For example, the highest RDA for iron in males over 4 years of age is 10 mg/day and for females over 4 years of age is 18 mg/day. Thus, the RDI for iron is set at 18 mg/day. Daily Reference Values (DRVs) are set for total fat, saturated fat, cholesterol, total carbohydrate, dietary fiber, sodium, potassium, and protein. The Daily Values appearing on food labels are based on a 2000-kcal reference diet, which approximates the weight maintenance requirements of postmenopausal women, women who exercise moderately, teenage girls, and sedentary men (figure 17.2). On large food labels, additional information is listed based on a daily intake of 2500 kcal, which is adequate for young men. The Daily Values for energy-producing nutrients are determined as a percentage of daily kilocaloric intake: 60% for carbohydrates, 30% for total fats, 10% for saturated fats, and 10% for proteins. The Daily Value for fiber is 14 g for each 1000 kcal of intake. The Daily Values for a nutrient in a 2000 kcal/day diet can be calculated on the basis of the recommended daily percentage of the nutrient and the kilocalories in a gram of the nutrient. For example, carbohydrates should constitute 60% of a 2000 kcal/day diet, or 1200 kcal/day (0.60 × 2000). Because there are 4 kilocalories in a gram of carbohydrate, the Daily Value for carbohydrate is 300 g/day (1200/4). 484 Chapter 17 their weight on a 2000 kcal/day diet, the total of the % Daily Values for each of these nutrients should be no more than 100%. However, for individuals consuming more or fewer than 2000 kcal per day, the total of the % Daily Values can be more or fewer than 100%. For example, for a person consuming 2200 kcal/day, the total of the % Daily Values for each of these nutrients should be no more than 110% because 2200/2000 = 1.10, or 110%. Predict 4 Suppose a person consumes 1800 kcal/day. What total % Daily Values for energy-producing nutrients is recommended? When using the % Daily Values of a food to determine how the amounts of certain nutrients in the food fit into the overall diet, the number of servings in a container or package needs to be considered. For example, suppose a small (2.25-oz) bag of corn chips has a % Daily Value of 16% for total fat. A person might suppose that eating the bag of chips accounts for 16% of total fat for the day. The bag, however, contains 2.5 servings. All the chips in the bag account for 40% (16% × 2.5) of the maximum recommended total fat. (a) Figure 17.2 Food Label (a) Current food label. (b) Revised food label proposed in early 2014. (b) A Case in Point Source: U.S. Food and Drug Administration. Vegetarian Diet The Daily Values for some nutrients are the uppermost limit considered desirable because of the link between these nutrients and certain diseases. Thus, the Daily Values for total fats are less than 65 g; saturated fats, less than 20 g; and cholesterol, less than 300 mg because of their association with increased risk of heart disease. The Daily Value for sodium is less than 2400 mg because of its association with high blood pressure in some people. For a particular food, the Daily Values are used to calculate the Percent Daily Value (% Daily Value) for some of the nutrients in one serving of the food (figure 17.2). For example, if a serving of food has 3 g of fat and the Daily Value for total fat is 65 g, then the % Daily Value is 5% (3/65 = 0.05, or 5%). The Food and Drug Administration (FDA) requires % Daily Values to be on food labels, so that the public has useful and accurate dietary information. In early 2014, the FDA proposed a major revision of food nutrition labels. The newly proposed nutrition labels will have calorie counts displayed more prominently, representing more reasonable serving sizes (Figure 17.2b). Manufacturers will also be required to list the amount of added sugar on the nutrition label, to assist the consumer in making better choices about total sugar intake, which has been linked to the current increase in obesity in the United States. Saturated fat and trans fat information will also be included, but the “Calories from Fat” on the old label will no longer be included. This reflects the modern view of nutritionists that the types of fats we ingest are more important than the amount. Once the new food label has been approved, manufacturers will have to include the revisions on most packaged foods within two years. Predict 3 One serving of a food has 30 g of carbohydrate. What % Daily Value for carbohydrate is on the food label for this food? The % Daily Values for nutrients related to energy consumption are based on a 2000 kcal/day diet. For people who maintain Hazel Nutt decides to switch from a typical American diet to a vegetarian diet because she believes a vegetarian diet is better for her health, and she no longer wants to eat animals. A strict vegetarian (or vegan) diet includes only plant foods. Plants alone can provide all the protein required for good health. But to get adequate amounts of the essential amino acids, a person following a vegan diet should consume a variety of protein sources, such as grains and legumes. In addition, the Dietary Guidelines for Americans recommends that vegan diets be supplemented with vitamin B12, vitamin D, calcium, iron, and zinc. This is especially important for children and pregnant and lactating women. Plant sources do not supply vitamin B12 or sufficient amounts of vitamin D, although the body can produce vitamin D with adequate exposure to sunlight (see chapter 5). Calcium is found in green leafy vegetables and nuts. Iron and zinc are in whole grains, nuts, and legumes. However, these minerals are either present in low amounts or not easily absorbed. 17.2 Metabolism Learning Outcomes A. B. C. D. E. F. G. H. I. After reading this section, you should be able to Define metabolism, anabolism, and catabolism. List three ways in which enzyme activity is controlled. Describe glycolysis, and name its products. Describe the citric acid cycle and its products. Describe the electron-transport chain and how ATP is produced in the process. Explain how the breakdown of glucose yields 2 ATP molecules in anaerobic respiration and 38 ATP molecules in aerobic respiration. Describe the basic steps involved in using lipids and amino acids as energy sources. Differentiate between the absorptive and postabsorptive metabolic states. Define metabolic rate. Nutrition, Metabolism, and Body Temperature Regulation 485 1 ATP Production The energy released during catabolism can be used to synthesize ATP. P P Catabolism P Anabolism ATP Catabolism is the energyreleasing process by which larger molecules are broken down to smaller ones. Ingested food is the source of molecules used in catabolic reactions. Energy Energy P P Anabolism is the energyrequiring process by which smaller molecules join to form larger ones. Anabolic reactions result in the synthesis of the molecules necessary for life. P ADP + Pi 2 ATP Breakdown The energy released from the breakdown of ATP can be used during anabolism to synthesize other molecules and to provide energy for cellular processes, such as active transport and muscle contraction. PROCESS Figure 17.3 ATP Derived from Catabolic Reactions Drives Anabolic Reactions Metabolism (mĕ-tab′ō-lizm; change) is the total of all the chemical reactions that occur in the body. It consists of catabolism (kătab′ō-lizm), the energy-releasing process by which large molecules are broken down into smaller ones, and anabolism (ă-nab′ō-lizm), the energy-requiring process by which small molecules are joined to form larger ones. Catabolism begins during the process of digestion and is concluded within individual cells. Anabolism occurs in all cells of the body as they divide to form new cells, maintain their own intracellular structure, and produce molecules such as hormones, neurotransmitters, or extracellular matrix molecules for export. The energy derived from catabolism is used to drive anabolic reactions. Metabolism can be divided into the chemical reactions that occur during digestion and the chemical reactions that occur after the products of digestion are taken up by cells. The chemical reactions that occur within cells are often referred to as cellular metabolism. The digestive products of carbohydrates, proteins, and lipids can be further broken down inside cells. The energy released during this breakdown can be used to combine ADP and an inorganic phosphate group (Pi) to form ATP (figure 17.3). ATP is often called the energy currency of the cell. When ATP is broken down to ADP, cells can use the released energy for active transport, muscle contraction, and molecule synthesis. Because the body has high energy demands, it uses ATP rapidly. Regulation of Metabolism The products of digestion, such as glucose, fatty acids, and amino acids, are molecules containing energy within their chemical bonds. A series of chemical reactions, called a biochemical pathway, controls the energy release from these molecules. At some of the steps, small amounts of energy are released; some of this energy is used to synthesize ATP (figure 17.4). About 40% of the energy in foods is incorporated into ATP; the rest is lost as heat. There are several different biochemical pathways inside cells. Which pathways function and how much each pathway is used is determined by enzymes because each step in the pathway requires a specific enzyme (see chapter 2). In turn, enzymes are regulated in several ways: 1. Enzyme synthesis. Enzymes are proteins, and their synthesis depends on DNA (see chapter 3). Thus, the types and amounts of enzymes present in cells are under genetic control. 2. Receptor-mediated enzyme activity. The combination of a chemical signal, such as a neurotransmitter or hormone, with a membrane-bound or intracellular receptor can activate or inhibit enzyme activity (see chapter 10). 3. Product control of enzyme activity. The end product of a biochemical pathway can inhibit the enzyme responsible for the first reaction in the pathway. This negative-feedback regulation prevents accumulation of the intermediate products and the end product of the pathway (figure 17.4). Carbohydrate Metabolism Monosaccharides are the breakdown products of carbohydrate digestion. Of these, glucose is the most important in terms of cellular metabolism. Glucose is transported in the circulation to all tissues of the body, where it is used as a source of energy. Any excess glucose in the blood following a meal can be used to form 486 Chapter 17 CLINICAL IMPACT Many metabolic disorders result from missing or dysfunctional enzymes. For example, in Tay-Sachs disease, the breakdown of lipids within lysosomes is impaired. Thus, the intermediate products of lipid metabolism accumulate abnormally, resulting in the destruction Enzymes and Disease of neurons and death by age 3–4 years. Phenylketonuria (fen′ il-kē′ tō-noo′ rē-a) (PKU) results from the inability to convert the amino acid phenylalanine to tyrosine. Therefore, phenylalanine accumulates in nerve cells and causes brain damage. Unlike Tay-Sachs disease, the extent of damage resulting from PKU can be limited by controlling the diet. Restricting the intake of phenylalanine is an effective treatment for PKU because it reduces the accumulation of phenylalanine in the body. This subsequently reduces the amount of damage to the brain. Negative-feedback inhibition Substance A Enzyme 1 Substance B Enzyme 2 Substance C Enzyme 3 Substance D Energy released is used to produce ATP or is lost as heat. Figure 17.4 Biochemical Pathway Each step in the pathway is regulated by a specific enzyme. Substance D can inhibit enzyme 1, thus regulating its own production. Some of the energy released by reactions in the pathway can be used to synthesize ATP. glycogen (gl ı̄′ kō-jen; glyks, sweet), or it can be partially broken down and the components used to form lipids. Glycogen is a short-term energy-storage molecule that the body can store only in limited amounts, whereas lipids are long-term energy-storage molecules that the body can store in large amounts. Most of the body’s glycogen is in skeletal muscle and in the liver. Glycolysis Glycolysis (glı̄-kol′ i-sis) is a series of chemical reactions that occurs in the fluid part of cytoplasm surrounding the organelles. It results in the breakdown of glucose to two pyruvic (pı̄-roo′ vik) acid molecules (figure 17.5). When glucose is converted to pyruvic acid, two ATP molecules are used and four ATP molecules are produced, for a net gain of two ATP molecules. Glucose consists of 6 carbon atoms, 12 hydrogen atoms, and 6 oxygen atoms covalently bonded together. During the breakdown of glucose, a hydrogen ion (H+) and two electrons (e−) are released and can attach to a carrier molecule, which moves the H+ and electrons to other parts of the cell. A very common carrier molecule in cells is nicotinamide adenine dinucleotide (nik-ōtin′ a-mı̄d ad′ ĕ-nēn d ı̄-noo′ klē-ō-t ı̄d) (NADH): NAD+ + 2 e− + H+ NADH The H and high-energy electrons in the NADH molecules can be used in other chemical reactions or in the production of ATP molecules in the electron-transport chain (described later in this section). + The pyruvic acid and NADH produced in glycolysis can be used in two different biochemical pathways, depending on the availability of O2. When the amounts of O2 are limited, anaerobic respiration can take place. Anaerobic respiration does not require O2 and can quickly produce a few ATP molecules for a short time. If the cell has adequate amounts of O2, the pyruvic acid and NADH produced in glycolysis are used in aerobic respiration to produce many more ATP. Anaerobic Respiration Lactic acid fermentation, a form of anaerobic respiration, is the breakdown of glucose in the absence of O2 to produce two molecules of lactic (lak′ tik) acid and two molecules of ATP (figure 17.5). The ATP thus produced is a source of energy during activities, such as intense exercise, when insufficient O2 is delivered to tissues. Lactic acid fermentation can be divided into two phases: 1. Glycolysis. Glucose undergoes several reactions to produce two pyruvic acid molecules, two ATP, and two NADH. 2. Lactic acid formation. Pyruvic acid is converted to lactic acid, a reaction that requires the input of energy from the NADH produced in phase 1 of lactic acid fermentation. Lactic acid is released from the cells that produce it, and blood transports it to the liver. When O2 becomes available, the lactic acid in the liver can be converted through a series of chemical reactions into glucose. The glucose then can be released from the Nutrition, Metabolism, and Body Temperature Regulation 1 Glycolysis converts glucose to two pyruvic acid molecules. There is a net gain of two ATP and two NADH from glycolysis. 2 Anaerobic respiration, which does not require O2, includes glycolysis and converts the two pyruvic acid molecules produced by glycolysis to two lactic acid molecules. This conversion requires energy, which is derived from the NADH generated in glycolysis. 487 Glucose 2 ATP Glycolysis 1 2 NADH Anaerobic respiration 2 pyruvic acid 2 2 lactic acid PROCESS Figure 17.5 Glycolysis and Anaerobic Respiration liver and transported in the blood to cells that use glucose as an energy source. Some of the reactions that convert lactic acid to glucose require the input of energy derived from ATP that is produced by aerobic respiration. The O2 necessary to make enough ATP for the synthesis of glucose from lactic acid contributes to the oxygen deficit (see chapter 7). Aerobic Respiration Aerobic (ār-ō′ bik) respiration is the breakdown of glucose in the presence of O2 to produce CO2, water, and 38 molecules of ATP (figure 17.6). Aerobic respiration can be divided into four phases: 1. Glycolysis. The six-carbon glucose molecule is broken down to form two molecules of pyruvic acid, each consisting of three carbon atoms. Two ATP and two NADH molecules are also produced. 2. Acetyl-CoA formation. Each pyruvic acid moves from the cytoplasm into a mitochondrion, where enzymes remove a carbon atom from the three-carbon pyruvic acid molecule to form CO2 and a two-carbon acetyl (as′ e-til, a-set′ il) group. Hydrogen ions and electrons are released in the reaction and can be used to produce NADH. Each acetyl group combines with coenzyme A (CoA), derived from vitamin B2, to form acetyl-CoA. Because two pyruvic acid molecules are produced in phase 1, phase 2 results in two acetyl-CoAs, two CO2 molecules, and two NADH molecules for each glucose molecule. 3. Citric acid cycle. Each acetyl-CoA combines with a fourcarbon molecule to form a six-carbon citric acid molecule, which enters the citric acid cycle. The citric (sit′ rik) acid cycle is also called the tricarboxylic (tr ı̄-kar-bok′ sil-ik) acid (TCA) cycle (citric acid has three carboxylic acid groups) or the Krebs cycle, after its discoverer, British biochemist Sir Hans Krebs (1900–1981). The citric acid cycle is a series of reactions wherein the six-carbon citric acid molecule is converted, in a number of steps, into a four-carbon molecule (figure 17.6). The four-carbon molecule can then combine with another acetyl-CoA molecule to form another citric acid molecule and reinitiate the cycle. During the cycle, two carbon atoms are used to form CO2, and energy, H+, and electrons are released. Some of the energy can be used to produce ATP. Most of the energy, H+, and electrons are used to form NADH molecules and another carrier molecule, called flavin (flā′ vin) adenine dinucleotide (FADH2). These molecules are used in the electrontransport chain to generate additional ATP. Carbon dioxide diffuses out of the cell and into the blood. It is transported by the circulatory system to the lungs, where it is expired. Thus, the carbon atoms that constitute food molecules, such as glucose, are eventually eliminated from the body as CO2. We literally breathe out part of the food we eat! 4. Electron-transport chain. The electron-transport chain is a series of electron-transport molecules attached to the inner mitochondrial membrane (figure 17.7). This membrane 488 Chapter 17 1 Glycolysis in the cytoplasm converts glucose to two pyruvic acid molecules and produces two ATP and two NADH. The NADH can go to the electron-transport chain in the inner mitochondrial membrane. Glucose (six-carbon molecule) 1 Glycolysis Cytoplasm 2 ATP 2 NADH 2 The two pyruvic acid molecules produced in glycolysis are converted to two acetyl-CoA molecules, producing two CO2 and two NADH. The NADH can go to the electron-transport chain. 2 pyruvic acid (three-carbon molecules) Outer mitochondrial membrane 4 Electron-transport chain Inner mitochondrial membrane 3 The two acetyl-CoA molecules enter the citric acid cycle, which produces four CO2, six NADH, two FADH2, and two ATP. The NADH and FADH2 can go to the electron-transport chain. 6 O2 6 H2O 2 CO2 34 ATP 2 NADH 2 4 The electron-transport chain uses NADH and FADH2 to produce 34 ATP. This process requires O2, which combines with H+ to form H2O. 2 acetyl-CoA (two-carbon molecules) 2 citric acid (six-carbon molecules) 2 four-carbon molecules 3 4 CO2 2 ATP 6 NADH 2 FADH2 PROCESS Figure 17.6 Aerobic Respiration Aerobic respiration involves four phases: (1) glycolysis, (2) acetyl-CoA formation, (3) the citric acid cycle, and (4) the electron-transport chain. The number of carbon atoms in a molecule is indicated after the molecule’s name. As glucose is broken down, the carbon atoms from glucose are incorporated into carbon dioxide. divides the interior of the mitochondrion into an inner compartment and an outer compartment. Electrons are transferred from NADH and FADH2 to the electron-transport carriers, and H+ is released into the inner mitochondrial compartment. Some of the electron-transport carriers are also H+ pumps, which use some of the energy from the transported electrons to pump H+ from the inner to the outer mitochondrial compartment. Because of an increased H+ concentration in the outer compartment, the H+ passes by diffusion back into the inner compartment. The H+ passes through channels in the inner mitochondrial membrane that couple the movement of the H+ to ATP production. In the last step of the electron-transport chain, two H+ and two electrons combine with an O2 atom to form H2O: 2 H+ + 2 e− + 12 O2 H 2O Without O2 to accept the H+ and electrons, the citric acid cycle and the electron-transport chain cannot function. Note that the O2 we breathe in is eventually bound to two hydrogen atoms to become water, which has many uses in the body (see chapter 2). Predict 5 Many poisons function by blocking certain steps in the metabolic pathways. For example, cyanide blocks the last step in the electron-transport chain. Explain why this blockage causes death. Summary of Anaerobic Respiration and Aerobic Respiration In anaerobic respiration, each glucose molecule (C6H12O6) yields 2 ATP and 2 lactic acid molecules (C3H6O3) through glycolysis: C6H12O6 + 2 ADP + Pi 2 C3H6O3 + 2 ATP Nutrition, Metabolism, and Body Temperature Regulation 489 Mitochondrion Cytosol Outer membrane H+ Outer compartment H+ Inner membrane H+ H+ 2 e– I H+ 2 H+ H+ H+ 2 e– III H+ H+ H+ II Inner compartment H+ H+ Carrier H+ protein H+ H+ ATP synthase ADP IV 2 e– NADH Pi + ADP 2 e– 2 e– H+ 1 H+ FADH2 H+ ATP 2 e– NAD+ 1 NADH or FADH 2 transfer their electrons to the electron-transport chain. PROCESS Figure 17.7 Pi ATP H2O 2 As the electrons move through the electron-transport chain, some of their energy is used to pump H+ into the outer compartment, resulting in a higher concentration of H+ in the outer than in the inner compartment. 2 H+ 1 2 O2 H+ 3 3 The H+ diffuse back into the inner compartment through special channels (ATP synthase) that couple the H+ movement with the production of ATP. The electrons, H+, and O2 combine to form H2O. 4 4 ATP is transported out of the inner compartment by a carrier protein that exchanges ATP for ADP. A different carrier protein moves phosphate into the inner compartment. Electron-Transport Chain The electron-transport chain in the inner membrane consists of four protein complexes (purple; numbered I to IV) with carrier molecules. In contrast, in aerobic respiration, each glucose molecule yields 38 ATP: C6H12O6 + 6 O2 + 38 ADP + 38 Pi 6 CO2 + 6 H2O + 38 ATP Of the 38 ATP molecules, 2 result from glycolysis, 2 result from the citric acid cycle, and 34 form through the electron-transport chain. Thus, aerobic respiration is much more efficient at producing ATP than is anaerobic respiration. In addition, many of the chemical reactions of aerobic respiration can be used to produce energy from other food molecules, such as lipids and proteins (see “Lipid Metabolism” and “Protein Metabolism” next). The number of ATP molecules produced during aerobic respiration can also be reported as 36. The 2 NADH molecules produced by glycolysis cannot cross the inner mitochondrial membrane; thus, their electrons are donated to a shuttle molecule that carries the electrons to the electron-transport chain. Depending on the shuttle molecule, each glycolytic NADH molecule can produce 2 or 3 ATP molecules. In skeletal muscle and in the brain, only 2 ATP molecules are produced for each NADH mol- ecule formed during glycolysis, resulting in a total number of 36 ATP molecules; however, in the liver, kidneys, and heart, 3 ATP molecules are produced for each NADH molecule, and the total number of ATP molecules formed is 38. Lipid Metabolism Triglycerides, or fat, are the body’s main energy-storage molecules. In a normal person, fat is responsible for about 99% of the body’s energy storage, and glycogen accounts for the remaining 1%. Between meals, triglycerides in adipose tissue are broken down into fatty acids and glycerol. Some of the fatty acids produced are released into the blood. Other tissues, especially skeletal muscle and the liver, use the fatty acids as a source of energy. The metabolism of fatty acids takes place in the mitochondria. It occurs by a series of reactions wherein two carbon atoms are removed from the end of a fatty acid chain to form acetyl-CoA (figure 17.8). As the process continues, carbon atoms are removed two at a time until the entire fatty acid chain is converted into acetyl-CoA. Acetyl-CoA can enter the citric acid cycle and be used 490 Chapter 17 Protein Metabolism to generate ATP. In the liver, two acetyl-CoA molecules can also combine to form ketones (kē′ tōnz). The ketones are released into the blood and travel to other tissues, especially skeletal muscle. In these tissues, the ketones are converted back to acetyl-CoA, which can enter the citric acid cycle to produce ATP. The presence of small amounts of ketones in the blood is normal and beneficial, but excessive production of ketones is called ketosis (ke-to′ sis). If the increased number of ketones exceeds the capacity of the body’s buffering systems, acidosis, a decrease in blood pH, can occur (see “Regulation of Acid-Base Balance,” chapter 18). Conditions that increase lipid metabolism can increase the rate of ketone formation. Examples are starvation (see the Clinical Impact “Starvation and Obesity” later in this chapter), diets consisting of proteins and fats with few carbohydrates, and untreated diabetes mellitus (see chapter 10). Because ketones are excreted by the kidneys and lungs, characteristics of untreated diabetes mellitus include ketones in the urine and “acetone breath.” Amino acids are the products of protein digestion. Once amino acids are absorbed into the body, they are quickly taken up by cells, especially in the liver. Amino acids are used primarily to synthesize needed proteins and only secondarily as a source of energy. If serving as a source of energy, amino acids can be used in two ways (figure 17.8): (1) The amino acids can be converted into the molecules of carbohydrate metabolism, such as pyruvic acid and acetyl-CoA. These molecules can be metabolized to yield ATP. (2) The amine group (−NH2) can be removed from the amino acid, leaving ammonia and an α-keto acid. This process produces NADH, which can enter the electron-transport chain to produce ATP. Ammonia is toxic to cells, so the liver converts it to urea, which the blood carries to the kidneys, where it is eliminated. The α-keto acid can enter the citric acid cycle or can be converted into pyruvic acid, acetyl-CoA, or glucose. Although proteins can serve as an energy source, they are not considered major storage molecules. Food Protein Carbohydrate Lipid Monosaccharides (e.g., glucose) Fatty acids Glycerol Glycolysis Ketones Pyruvic acid Acetyl -CoA Amino acids Citric acid cycle ATP O2 α-keto acid NADH CO2 NADH FADH2 Electrontransport chain H2O ATP Figure 17.8 Overall Pathways for the Metabolism of Food Carbohydrates, lipids, and proteins enter biochemical pathways to produce energy in the cell. Ammonia Urea 491 Nutrition, Metabolism, and Body Temperature Regulation Metabolic States The body experiences two major metabolic states. The first is the absorptive state, the period immediately after a meal, when nutrients are being absorbed through the intestinal wall into the circulatory and lymphatic systems (figure 17.9). The absorptive state usually lasts about 4 hours after each meal, and most of the glucose that enters the circulation is used by cells for the energy they require. The remainder of the glucose is converted into glycogen or lipids. Most of the absorbed fats are deposited in adipose tissue. Many of the absorbed amino acids are used by cells in gene expression, and some are used for energy; still others enter the liver and are converted to lipids or carbohydrates. The second state, the postabsorptive state, occurs late in the morning, late in the afternoon, or during the night after each absorptive state is concluded (figure 17.10). Normal blood glucose levels range between 70 and 110 mg/dL, and it is vital to the body’s homeostasis that this range be maintained. During the postabsorptive state, blood glucose levels are maintained by the conversion of other molecules to glucose. The first source of blood glucose during the postabsorptive state is the glycogen stored in the liver. However, this glycogen supply can provide glucose for only about four hours. The glycogen stored in skeletal muscles can also be used during vigorous exercise. As the glycogen stores are depleted, the body uses lipids as an energy source. The glycerol from triglycerides Nutrients Absorbed Nutrients are absorbed from the digestive tract and carried by the blood to the liver. Metabolic Rate Metabolic rate is the total amount of energy produced and used by the body per unit of time. Metabolic rate is usually estimated by measuring the amount of oxygen used per minute. One liter of oxygen consumed by the body is estimated to produce 4.825 kcal of energy. Metabolic energy can be used in three ways: for basal metabolism, for muscle contraction, and for the assimilation of food, which involves processes such as the production of digestive enzymes and the active transport of digested molecules. The basal metabolic rate (BMR) is the energy needed to keep the resting body functional. It is the metabolic rate calculated in expended kilocalories per square meter of body surface area per hour. BMR is measured when a person is awake but restful and has not eaten for 12 hours. A typical BMR for a 70-kg (154-lb) male is 38 kcal/m2/hour. Amino acids Nutrients Processed The liver converts nutrients into energy-storage molecules, such as glycogen, fatty acids, and triglycerides. Amino acids are also used to synthesize proteins, such as plasma proteins. Fatty acids and triglycerides produced by the liver are released into the blood. Nutrients not processed by the liver are also carried by the blood to tissues. Nutrients Stored and Used Nutrients are stored in adipose tissue as triglycerides and in muscle as glycogen. Nutrients also are a source of energy for tissues. Amino acids are used to synthesize proteins. can be converted to glucose. The fatty acids can be converted to acetyl-CoA, moved into the citric acid cycle, and used as a source of energy to produce ATP. In the liver, acetyl-CoA can be used to produce ketone bodies that other tissues can use for energy. The use of fatty acids as an energy source can partly eliminate the need to use glucose for energy, so that less glucose is removed from the blood and homeostasis is maintained. The amino acids of proteins can be converted to glucose or can be used to produce energy, again sparing blood glucose. Proteins Triglycerides Nonessential amino acids Glucose Glycogen Glycerol _-keto acids Ammonia Acetyl-CoA Urea Fatty acids Energy Amino acids Glucose Proteins Glycogen Glucose Fatty acids Glucose Fatty acids Glucose Glycerol Muscle Energy Triglycerides Adipose tissues Most tissues (including muscle and adipose) Energy Nervous tissue Figure 17.9 Events of the Absorptive State Absorbed molecules, especially glucose, are used as sources of energy. Molecules not immediately needed for energy are stored: Glucose is converted to glycogen or triglycerides, triglycerides are deposited in adipose tissue, and amino acids are converted to triglycerides or carbohydrates. 492 Chapter 17 Stored Nutrients Used Stored energy molecules are used as sources of energy: Glycogen is converted to glucose, and triglycerides are converted to fatty acids. Molecules released from tissues are carried by the blood to the liver. Muscle Proteins Glycogen Amino acids Glucose Adipose tissue Triglycerides Glycerol Energy Nutrients Processed The liver processes molecules to produce additional energy sources: Glycogen and amino acids are converted to glucose and fatty acids to ketones. Glucose and ketones are released into the blood and are transported to tissues. Lactic acid Fatty acids Most tissues (including muscle) Nervous tissue Energy Energy Fatty acids Ketone bodies Glucose Energy Glycerol Fatty acids Glycogen Acetyl-CoA Ketone bodies Energy Glucose _-keto acid Amino acids Ammonia Urea Energy Figure 17.10 Events of the Postabsorptive State Stored energy molecules are used as sources of energy: Glycogen is converted to glucose; triglycerides are broken down to fatty acids, some of which are converted to ketones; and proteins are converted to glucose. Basal metabolism supports active transport mechanisms, muscle tone, maintenance of body temperature, beating of the heart, and other activities. A number of factors can affect the BMR. Males have a higher BMR than females, younger people have a higher BMR than older people, and fever can increase BMR. Greatly reduced kilocaloric input, as occurs during dieting or fasting, depresses the BMR. The daily input of energy should equal the energy demands of metabolism; otherwise, a person will gain or lose weight. For a 23-year-old, 70-kg (154-lb) male to maintain his weight, the input should be 2700 kcal/day; for a 58-kg (128-lb) female of the same age, 2000 kcal/day are necessary. A pound of body fat contains about 3500 kcal. Reducing kilocaloric intake by 500 kcal/day can result in the loss of 1 lb of fat per week. Clearly, adjusting kilocaloric input is an important way to control body weight. The other way to control weight is through energy expenditure. Physical activity through skeletal muscle movement (exercise) greatly increases the metabolic rate. In the average person, basal metabolism accounts for about 60% of energy expenditure, muscular activity 30%, and assimilation of food about 10%. Of these amounts, energy loss through muscular activity is the only component that a person can reasonably control. Comparing the number of kilocalories gained from food and the number of kilocalories burned during exercise reveals why losing weight is difficult. For example, if brisk walking uses 225 kcal/h, it takes 20 minutes of brisk walking to burn off the 75 kcal in one slice of bread (75/225 = 0.33 h). Research suggests that a combination of appropriate physical activity and appropriate kilocaloric intake is the best approach to maintaining a healthy body composition and weight. A Case in Point Gastric Bypass Surgery Les Moore is a 35-year-old male who weighs 420 pounds. Although at one time he weighed less as a result of dieting, he has not only regained the lost weight but also gained additional weight. Les has type 2 diabetes, high blood cholesterol, and high blood pressure. Because of his extreme obesity and related health problems, Les elects to have a Roux-en-Y gastric bypass. In this procedure, the digestive tract is surgically rearranged to form a Y-shaped structure. One arm of the Y is formed by separating the superior part of the stomach to form a small pouch that is connected to the jejunum. The small size of the stomach pouch dramatically reduces the amount of food a person can eat. The other arm of the Y consists of the remainder of the stomach, the duodenum, and part of the jejunum. The body of the Y is the distal jejunum. Food from the esophagus enters the small stomach pouch and bypasses the rest of the stomach and the duodenum by entering the jejunum. Bile and pancreatic juices enter the duodenum and pass into the jejunum. Roux-en-Y is the most commonly performed gastric bypass surgery in the United States. It results in the permanent loss of over 50% of presurgery body weight for over 90% of patients. It also cures most cases of type 2 diabetes, lowers blood pressure, decreases cholesterol levels, reduces the risk of premature death, and improves the quality of life in most patients. But despite the benefits, gastric bypass has some negatives. It is major surgery— complications and even death are possible. Also, the normal digestive functions of the stomach and duodenum are bypassed as food moves quickly through the stomach pouch into the jejunum. As a result, vitamin and mineral deficiencies can develop—particularly, deficiencies of vitamin B12, calcium, and iron. Nutrition, Metabolism, and Body Temperature Regulation CLINICAL IMPACT Starvation Starvation is the inadequate intake of nutrients or the inability to metabolize or absorb nutrients. Starvation can be caused by a number of factors, such as prolonged fasting, anorexia, deprivation, or disease. No matter what the cause, starvation takes approximately the same course and consists of three phases. The events of the first two phases occur even during relatively short periods of fasting or dieting. The third phase occurs in prolonged starvation and ends in death. During the first phase of starvation, blood glucose levels are maintained through the production of glucose from glycogen, proteins, and fats. At first, glycogen is broken down into glucose. However, enough glycogen is stored in the liver to last only a few hours. Thereafter, blood glucose levels are maintained by the breakdown of proteins and fats. Fats are decomposed into fatty acids and glycerol. Fatty acids can be used as a source of energy, especially by skeletal muscle, thus decreasing the use of glucose by tissues other than the brain. The brain cannot use fatty acids as an energy source, so the conservation of glucose is critical to normal brain function. Glycerol can be used to make a small amount of glucose, but most of the glucose is formed from the amino acids of proteins. In addition, some amino acids can be used directly for energy. In the second stage, which can last for several weeks, fats are the primary energy source. The liver metabolizes fatty acids into ketone bodies that can serve as a source of energy. After about a week of fasting, the brain begins to use ketone bodies, as well as glucose, for energy. This usage decreases the demand for glucose, and the rate of protein breakdown diminishes but does not stop. In addition, the use of proteins is selective; that is, proteins not essential for survival are used first. The third stage of starvation begins when the fat reserves are nearly depleted and the body switches to proteins as the major energy source. Muscles, the largest source of protein in the body, are rapidly depleted. At the end of this stage, proteins essential for cellular functions are broken down, and cell function degenerates. Death can occur very rapidly. 493 Starvation and Obesity Symptoms of starvation, in addition to weight loss, include apathy, listlessness, withdrawal, and increased susceptibility to infectious diseases. Few people die directly from starvation because they usually die of an infectious disease first. Other signs of starvation include changes in hair color, flaky skin, and massive edema in the abdomen and lower limbs, causing the abdomen to appear bloated. During starvation, the body’s ability to consume normal volumes of food also decreases. Foods high in bulk but low in protein content often cannot reverse the process of starvation. Intervention involves feeding the starving person low-bulk foods containing ample protein, calories, vitamins, and minerals. Starvation also results in dehydration; thus, rehydration is an important part of intervention. Even with intervention, a victim may be so affected by disease or weakness that he or she cannot recover. Obesity Obesity is the storage of excess fat, and it results from the ingestion of more food than is necessary for the body’s energy needs. Obesity can be defined on the basis of body weight, body mass index, or body fat. “Desirable body weight” is listed in a table produced by the Metropolitan Life Insurance Company and indicates, for any height, the weight associated with a maximum lifespan. Overweight is defined as 10% more than the desirable weight, and obesity is 20% more than the desirable weight. body mass index (bMI) can be calculated by dividing a person’s weight (Wt) in kilograms by the square of his or her height (Ht) in meters: BMI = Wt/Ht2 A BMI greater than 25–27 is overweight, and a value greater than 30 is defined as obese. About 26% of Americans have a BMI of 30 or greater. In terms of the percent of total body weight contributed by fat, 15% body fat in men and 25% body fat in women is associated with reduced health risks. Obesity is defined as more than 25% body fat in men and 30–35% in women. The distribution of fat in obese individuals can vary. Fat can accumulate mainly in the upper body, such as in the abdominal region, or it can be associated with the hips and buttocks. These distribution differ- ences can be clinically significant because upper body obesity is associated with an increased likelihood of diabetes mellitus, cardiovascular disease, stroke, and death. In some cases, obesity is caused by a medical condition. For example, a tumor in the hypothalamus can stimulate overeating. In most cases, however, no specific cause can be recognized. In fact, obesity can occur for many reasons and can have more than one cause in the same individual. There seems to be a genetic component for obesity; if one or both parents are obese, their children are more likely to be obese. Environmental factors, such as eating habits, can also play an important role. For example, adopted children can exhibit obesity similar to that of their adoptive parents. In addition, psychological factors, such as overeating as a way to deal with stress, can contribute to obesity. In hypertrophic (hı̄-per-trof′ik; hyper-, above normal + trophē, nourishment) obesity, the number of adipose cells is usually normal, but the amount of fat contained in each adipose cell is increased. This type of obesity is characteristic of adult-onset obesity. People who were thin or of average weight and quite active when young become less active as they age. Although they no longer use as many kilocalories, they still consume the same amount of food as when they were younger. The excess kilocalories (see “Metabolic Rate” earlier in this section) are used to synthesize fat. In this type of obesity, the amount of fat in each adipose cell increases, and if the amount of stored fat continues to increase, the total number of adipose cells may also increase. It is estimated that the average U.S. resident gains 1.25–1.5 lb of fat per year after age 25 and, at the same time, loses 0.25–0.5 lb of lean body weight (muscle mass) per year. In hyperplastic (hı̄-per-plas′tik; hyper+ plasis, a molding) obesity, which is characteristic of juvenile-onset obesity, the number of adipose cells is increased. This condition may also be accompanied by an increase in cell size (hypertrophic obesity). Hyperplastic obesity has a very strong hereditary component, but family eating habits can also have a great influence. People with hyperplastic obesity are obese as children and become more obese with age. This type of obesity is a major health problem in school-age children. 494 Chapter 17 Predict 6 Radiation from sun and water If watching TV uses 95 kcal/h, how long does it take to burn off the kilocalories in one cola or beer (see table 17.1)? If jogging at a pace of 6 mph uses 580 kcal/h, how long does it take to use the kilocalories in one cola or beer? 17.3 Body Temperature Regulation Learning Outcome Evaporation After reading this section, you should be able to A. Describe heat production and regulation in the body. Humans can maintain a relatively constant internal body temperature despite changes in the temperature of the surrounding environment. A constant body temperature is very important to homeostasis. For example, environmental temperatures are too low for normal enzyme function. The heat produced by metabolism and muscle contraction helps maintain the body temperature at a steady, elevated level that is high enough for normal enzyme function. Excessively high temperatures can alter enzyme structure, resulting in loss of the enzyme’s function. Free energy is the total amount of energy that can be liberated by the complete catabolism of food. About 40% of the total energy released by catabolism is used to accomplish biological work, such as anabolism, muscular contraction, and other cellular activities. The remaining energy is lost as heat. Predict 7 Explain why we become warm during exercise. Why is shivering useful when it is cold? Normal body temperature is regulated in the same way as other homeostatic conditions in the body. The average normal temperature is usually considered to be 37°C (98.6°F) when measured orally and 37.6°C (99.7°F) when measured rectally. Rectal temperature comes closer to the true core body temperature, but an oral temperature is more easily obtained in older children and adults and therefore is the preferred measure. The normal oral temperature may vary from person to person, with a range of approximately 36.1–37.2°C (97–99°F). Body temperature is maintained by balancing heat input with heat loss. The body and the environment exchange heat in a number of ways (figure 17.11). Radiation is the gain or loss of heat as infrared energy between two objects that are not in physical contact with each other. For example, heat can be gained by radiation from the sun, a hot coal, or the hot sand of a beach. On the other hand, heat can be lost as radiation to cool vegetation, water in the ocean, or snow on the ground. Conduction is the exchange of heat between objects that are in direct contact with each other, such as the bottom of the feet and the ground. Convection is a transfer of heat between the body and the air or water. A cool breeze moves air over the body and causes loss of heat from the body. Evaporation is the conversion of water from a liquid to a gaseous form. As water evaporates from body surfaces, heat is lost. The amount of heat exchanged between the environment and the body is determined by the difference in temperature between the two. The greater the temperature difference, the greater the rate of heat exchange. Control of the temperature difference can be used to regulate body temperature. For example, if the environmental Convection from cool breeze Radiation from sand Conduction from hot sand Figure 17.11 Heat Exchange Heat exchange between a person and the environment occurs by convection, radiation, evaporation, and conduction. Arrows show the direction of net heat gain or loss in this environment. temperature is very cold, as occurs on a winter day, there is a large temperature difference between the body and the environment, and therefore a large loss of heat. Behaviorally, we can reduce the loss of heat by selecting a warmer environment, such as by going inside a heated house or by putting on extra clothes. Physiologically, the body controls the temperature difference through dilation and constriction of blood vessels in the skin. When the blood vessels dilate, they bring warm blood to the surface of the body, raising skin temperature; when the blood vessels constrict, blood flow and skin temperature decrease (see figure 5.8). Predict 8 Explain why constriction of skin blood vessels on a cold winter day is beneficial. When environmental temperature is greater than body temperature, dilation of blood vessels in the skin brings blood to the skin, causing an increase in skin temperature that decreases the gain of heat from the environment. At the same time, evaporation carries away excess heat to prevent heat gain and overheating. Body temperature regulation is an example of a negativefeedback system (figure 17.12). Maintenance of a specific body temperature is accomplished by neurons in the hypothalamus, which regulate body temperature around a set point. A small area in the anterior part of the hypothalamus can detect slight increases in body temperature through changes in blood temperature. As a Nutrition, Metabolism, and Body Temperature Regulation 3 4 Actions Homeostasis Disturbed: Body temperature increases. Start here Homeostasis Disturbed: Body temperature decreases. Actions Receptors in the skin and hypothalamus detect decreases in body temperature. The control center in the hypothalamus activates heat-conserving and heat-generating mechanisms. Effectors Respond: Increased sweating increases evaporative heat loss. Dilation of skin blood vessels increases heat loss from the skin. Behavioral modifications, such as taking off a jacket or seeking a cooler environment, increase heat loss. 5 6 Homeostasis Restored: Body temperature decreases. Body temperature (normal range) 1 2 Body temperature (normal range) Receptors in the skin and hypothalamus detect increases in body temperature. The control center in the hypothalamus activates heat loss mechanisms. Reactions Homeostasis Restored: Body temperature increases. Reactions Effectors Respond: Constriction of skin blood vessels decreases heat loss from the skin. Shivering increases heat production. Behavioral modifications, such as putting on a jacket or seeking a warmer environment, decrease heat loss. Homeostasis Figure 17.12 Temperature Regulation (1) Body temperature is within normal range. (2) Body temperature increases outside the normal range, which causes homeostasis to be disturbed. (3) Receptors in the skin and hypothalamus detect the increase in body temperature and the control center in the hypothalamus responds to the change in body temperature. (4)The effectors are activated. Blood vessels in the skin dilate and sweating increases to promote heat loss and evaporative cooling. (5) Body temperature decreases. (6) Body temperature returns to its normal range, and homeostasis is restored. 495 496 Chapter 17 CLINICAL IMPACT Hyperthermia is a condition in which heat gain in the body exceeds heat loss. Hyperthermia can result from exposure to a hot environment, exercise, or fever. Prolonged exposure to a hot environment can lead to heat exhaustion, in which normal temperature reduction mechanisms cannot keep pace with the excessive environmental heat, thus allowing the body temperature to rise. Heat exhaustion is characterized by cool, wet skin due to heavy sweating. Weakness, dizziness, and nausea usually occur as well. The heavy sweating can lead to dehydration, decreased blood volume, decreased blood pressure, and increased heart rate. Too Hot or Too Cold Treatment involves increasing heat loss by moving the person to a cooler environment, decreasing heat production by decreasing muscular activity, and replacing lost body fluids. Heat stroke results from an increase in the hypothalamic set point and is characterized by dry, flushed skin because sweating is inhibited. The person becomes confused and irritable, and can even become comatose. Treatment is the same as for heat exhaustion but also involves increasing evaporation from the skin by applying water to the skin or by placing the person in cool water. Hypothermia is a condition in which heat loss exceeds heat gain. The body’s result, mechanisms that cause heat loss, such as dilation of blood vessels in the skin and sweating, are activated, and body temperature decreases. A small area in the posterior hypothalamus can detect slight decreases in body temperature and can initiate heat gain by increasing muscular activity (shivering) and by initiating constriction of blood vessels in the skin. ANSWER TO LEARN TO PREDICT Although Sadie’s suggested snack did contain a lot of calories, which she and David needed for their day at the park, the food choices were not ideal. Most of the calories in the cookies and grape soda were actually from simple sugars. Eating large amounts of simple sugars, such as Sadie’s suggested snack, could result in large fluctuations in blood glucose levels. Though the children may initially have an increase in energy, they will most likely experience a drastic decrease in energy as well. normal temperature increase mechanisms are working, but they cannot keep pace with heat loss, and the body temperature decreases. Hypothermia usually results from prolonged exposure to a cold environment or even to a cool, damp environment because the moisture draws heat away from the body. Treatment for hypothermia calls for rewarming the body at a rate of a few degrees per hour. Frostbite is local damage to the skin or deeper tissues resulting from prolonged exposure to a cold environment. The best treatment for frostbite is immersion in a warm-water bath. Rubbing the affected area and applying local, dry heat should be avoided. Under some conditions, the hypothalamus set point is actually changed. For example, during a fever, the set point is raised. Heat-conserving and heat-producing mechanisms are stimulated, and body temperature increases. To recover from a fever, the set point is reduced to normal, heat loss mechanisms are initiated, and body temperature decreases. Foods that include complex carbohydrates, such as those in the snack Sadie’s mom suggested, have other nutrients, such as vitamins, many of which are necessary for normal metabolism. In addition, complex carbohydrates are digested and absorbed at a slower rate and do not contribute to drastic changes in blood glucose levels. Essentially, Sadie’s mom selected food that would provide the children with energy and additional beneficial nutrients. Answers to the rest of this chapter’s Predict questions are in Appendix E. SUMMARY 17.1 Nutrition (p. 476) Nutrition is the ingestion and use of food. Nutrients 1. Nutrients, the chemicals used by the body, consist of carbohydrates, lipids, proteins, vitamins, minerals, and water. 2. Essential nutrients either cannot be produced by the body or cannot be produced in adequate amounts. 3. The MyPlate icon provides a visual reminder for making choices at mealtime, by selecting healthy foods from five food groups. Half the meal should be fruits and vegetables. Kilocalories A kilocalorie is the energy required to raise the temperature of 1000 g of water 1°C. A kilocalorie (Calorie) is the unit of measurement used to express the energy content of food. Nutrition, Metabolism, and Body Temperature Regulation Carbohydrates 1. Carbohydrates include monosaccharides, disaccharides, and polysaccharides. 2. Most of the carbohydrates we ingest are from plants. 3. Carbohydrates are used as an energy source and for making DNA, RNA, and ATP. 4. The Acceptable Macronutrient Distribution Range (AMDR) for carbohydrates is 45–65% of total kilocalories. Lipids 1. Lipids include triglycerides, steroids, phospholipids, and fat-soluble vitamins. Triglycerides are a major source of energy. Eicosanoids are involved in inflammation, tissue repair, and smooth muscle contraction. Cholesterol and phospholipids are part of the cell membrane. Some steroid hormones regulate the reproductive system. 2. The AMDR for lipids is 20–35%. Proteins 1. Proteins are chains of amino acids. 2. Animal proteins tend to be complete proteins, whereas plant proteins tend to be incomplete. 3. Proteins are involved in structural strength, muscle contraction, regulation, buffering, clotting, transport, ion channels, receptors, and the immune system. 4. The AMDR for protein is 10–35% of total kilocalories. Vitamins 1. Most vitamins are not produced by the body and must be obtained in the diet. Some vitamins can be formed from provitamins. 2. Vitamins are important in energy production, nucleic acid synthesis, growth, and blood clotting. 3. Vitamins are classified as either fat-soluble or water-soluble. 4. Recommended dietary allowances (RDAs) are a guide for estimating the nutritional needs of groups of people on the basis of their age, their gender, and other factors. Minerals Minerals are essential for normal metabolic functions. They are involved with establishing the resting membrane potential; generating action potentials; adding mechanical strength to bones and teeth; combining with organic molecules; and acting as coenzymes, buffers, or regulators of osmotic pressure. Daily Values 1. Daily Values are dietary references that can be used to plan a healthful diet. 2. Daily Values for vitamins and minerals are based on Reference Daily Intakes (RDIs), which are generally the highest 1968 RDA value of an age category. 3. Daily Values are based on Daily Reference Values. The Daily Reference Values for energy-producing nutrients (carbohydrates, total fat, saturated fat, and proteins) and dietary fiber are recommended percentages of the total kilocalories ingested daily for each nutrient. The Daily Reference Values for total fats, saturated fats, cholesterol, and sodium are the uppermost limits considered desirable because of their link to diseases. 4. The % Daily Value is the percentage of the recommended Daily Value of a nutrient found in one serving of a particular food. 17.2 Metabolism (p. 484) 1. Metabolism consists of catabolism and anabolism. Catabolism, the breakdown of molecules, gives off energy. Anabolism, the synthesis of molecules, requires energy. 497 2. The energy in carbohydrates, lipids, and proteins is used to produce ATP. 3. The energy from ATP can be used for active transport, muscle contraction, and the synthesis of molecules. Regulation of Metabolism 1. A biochemical pathway is a series of chemical reactions, some of which release energy that can be used to synthesize ATP. 2. Each step in a biochemical pathway requires enzymes. 3. Enzyme synthesis is determined by DNA. Enzyme activity is modified by receptor-mediated and end-product processes. Carbohydrate Metabolism 1. Glycolysis is the breakdown of glucose to two pyruvic acid molecules. Two ATP molecules are also produced. 2. Anaerobic respiration is the breakdown of glucose in the absence of oxygen to two lactic acid molecules and two ATP molecules. 3. Lactic acid can be converted to glucose using aerobically produced ATP; the necessary oxygen contributes to the oxygen deficit. 4. Aerobic respiration is the breakdown of glucose in the presence of oxygen to produce carbon dioxide, water, and 38 molecules of ATP. The first phase of aerobic respiration is glycolysis; the second phase is the conversion of pyruvic acid to acetyl-CoA; the third phase is the citric acid cycle; and the fourth phase is the electrontransport chain, which uses carrier molecules, such as NADH, to synthesize ATP. Lipid Metabolism 1. Lipids are broken down in adipose tissue, and fatty acids are released into the blood. 2. Fatty acids are taken up by cells and broken down into acetyl-CoA, which can enter the citric acid cycle. Acetyl-CoA can also be converted into ketones by the liver. Ketones released from the liver into the blood are used as energy sources by other cells. Protein Metabolism 1. Amino acids are used to synthesize proteins. 2. Amino acids can be used for energy, yielding ammonia as a by-product. Ammonia is converted to urea and excreted by the kidneys. Metabolic States 1. In the absorptive state, nutrients are used as energy, with the remainder being stored. 2. In the postabsorptive state, stored nutrients are used for energy. Metabolic Rate 1. The metabolic rate is the total energy expenditure per unit of time. 2. Metabolic energy is used for basal metabolism, muscular activity, and the assimilation of food. 17.3 Body Temperature Regulation (p. 494) 1. Body temperature is a balance between heat gain and heat loss. 2. Heat is produced through metabolism. 3. Heat is exchanged through radiation, conduction, convection, and evaporation. 4. The greater the temperature difference, the greater the rate of heat exchange. 5. Body temperature is maintained around a set point by neural circuits in the hypothalamus. 6. Dilation of blood vessels in the skin and sweating increase heat loss from the body. 7. Constriction of blood vessels in the skin and shivering promote heat gain by the body. 498 Chapter 17 Review and Comprehension 1. Define a nutrient, and list the six major classes of nutrients. What is an essential nutrient? 2. What is a kilocalorie (Calorie)? Distinguish between a Calorie and a calorie. 3. List some sources of carbohydrates, lipids, and proteins in the diet. 4. List the recommended consumption amounts of carbohydrates, lipids, and proteins. 5. What are vitamins and provitamins? Name the water-soluble vitamins and the fat-soluble vitamins. List some of the functions of vitamins. 6. What are the Recommended Dietary Allowances (RDAs)? 7. List some of the minerals, and give their functions. 8. What are the Daily Values? How are the Daily Values related to total daily kilocaloric intake? Why are some Daily Values considered the uppermost amounts that should be consumed? 9. Define a % Daily Value. 10. Define a biochemical pathway. How are the steps in a biochemical pathway controlled? What are three ways in which enzymes are regulated? 11. Describe glycolysis. What molecule is the end product of glycolysis? How many ATP and NADH molecules are produced? 12. What determines whether the pyruvic acid produced in glycolysis becomes lactic acid or acetyl-CoA? 13. Describe the two phases of anaerobic respiration. How many ATP molecules are produced? What happens to the lactic acid produced when oxygen becomes available? 14. Define aerobic respiration, and state how many ATP molecules are produced. 15. Describe the citric acid cycle. 16. What is the function of the electron-transport chain? 17. What happens to the carbon atoms in ingested food during metabolism? What happens to the oxygen we breathe in during metabolism? 18. Describe the events occurring during the absorptive and postabsorptive metabolic states. 19. What is meant by metabolic rate? Name three ways that the body uses metabolic energy. 20. Describe how heat is produced by and lost from the body. How is body temperature regulated? Critical Thinking 1. One serving of a food contains 2 g of saturated fat. What % Daily Value for saturated fat would appear on the food label? (See the bottom of figure 17.2a for information needed to answer this question.) 2. An active teenage boy consumes 3000 kcal/day. What is the maximum amount (weight) of total fats he should consume, according to the % Daily Values? 3. If the teenager in question 2 eats a food that has a total fat content of 10 g/serving, what is his total fat % Daily Value? 4. Suppose the food in question 3 is in a package that lists a serving size of 12 cup, with four servings in the package. If the teenager eats half the contents of the package (1 cup), how much of his % Daily Value does he consume? 5. Why can some people lose weight on a 1200 kcal/day diet, whereas other people cannot? 6. Lotta Bulk, a bodybuilder, wanted to increase her muscle mass. Knowing that proteins are the main components of muscle, she consumed large amounts of protein daily (high-protein diet), along with small amounts of lipids and carbohydrates. Explain why this strategy will or will not work. 7. After consuming a high-protein diet for several days, does Lotta Bulk’s urine contain less, the same amount of, or more urea than before she consumed the proteins? Explain. 8. Thyroid hormone is known to increase the activity of the sodiumpotassium exchange pump, which is an active-transport mechanism, thereby increasing the breakdown of ATP. If a person produced excess amounts of thyroid hormone, what effect would this have on basal metabolic rate, body weight, and body temperature? How might the body attempt to compensate for the changes in body weight and temperature? 9. On learning that sweat evaporation results in the loss of calories, an anatomy and physiology student decides that sweating is an easier way to lose weight than dieting. He knows that a liter (about a quart) of water weighs 1000 g, which is equivalent to 580,000 cal (or 580 kcal) of heat when lost as sweat. He believes that instead of reducing his caloric intake by 580 kcal/day, he can lose about a pound of fat a week by losing a liter of sweat every day in the sauna. Will this approach work? Explain. 10. It is recommended that a person on a diet drink six to eight glasses of cool water per day. How could this practice aid weight loss? 11. In some diseases, an infection results in a high fever. The patient is on the way to recovery when the crisis is over and body temperature begins to return to normal. If you were looking for symptoms in a patient who had just passed through the crisis state, would you look for a dry, pale skin or a wet, flushed skin? Explain. Answers in Appendix D