Consolidation 1 - Notes - Nottingham Trent University PDF
Document Details
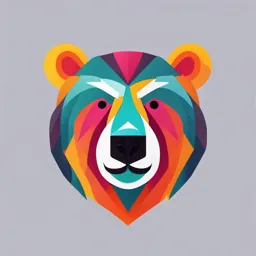
Uploaded by RecordSettingCliché4168
Nottingham Trent University
Tags
Summary
These notes provide supplementary information to the "Consolidation 1" videos for Nottingham Trent University's DESN22053 Ground Engineering module. They cover topics such as consolidation in saturated soils, the three stages of consolidation (immediate, primary, and secondary), drainage and consolidation, and normally/over-consolidated clays. The notes include detailed explanations and definitions.
Full Transcript
**Consolidation 1 - notes** These notes are supplementary to the "Consolidation 1" videos, and should be used in addition to the information presented in those videos. **Introduction** Previously we have discussed the increase in stresses in a soil that lead to shear failure. However, it is possi...
**Consolidation 1 - notes** These notes are supplementary to the "Consolidation 1" videos, and should be used in addition to the information presented in those videos. **Introduction** Previously we have discussed the increase in stresses in a soil that lead to shear failure. However, it is possible to subject soils to stresses which are not great enough to cause failure, but which cause strains within the soil, leading to a reduction in volume. For [saturated soils], the reduction in volume over time is known as **consolidation**. **So, what is consolidation?** **Saturated** soil contains only soil grains and water (i.e. no air). Both the soil grains and the water can be considered to be incompressible, and so volume can only change if water can drain out (i.e. the soil is in a '[drained]' condition). Thus, consolidation takes place as seepage occurs. (In unsaturated soil, containing soil grains, air and water, volume changes can occur if air is forced out -- this is known as **compaction**). During consolidation (and also compaction), loading of the soil will bring the soil particles closer together leading to an increase in strength and stiffness, and a reduction in volume of the soil. During consolidation the individual soil particles may re-arrange position, and the soil may also strain elastically. If the soil is unloaded it will swell as the elastic strain is recovered, but the positional re-arrangement of the soil particles will not be fully recovered. So, unloading the soil will give an increase in volume but not of the same magnitude as the volume reduction during consolidation. The swelling on unloading is known as '**heave**', and leads to reduction in strength and stiffness. There are three distinct stages to settlement/consolidation. Immediately upon loading, shear strains cause the soil to change shape (but the volume remains constant). This '**immediate settlement**' (which is relatively small) can be recovered on removal of the load. **Consolidation settlement** (also known as '**primary consolidation**') then takes place as water seepage occurs and soil particles re-arrange in a closer packed state: [this stage is where the great majority of the volume reduction takes place] and when consolidation calculations are conducted it is often only this stage which is taken in to account. Finally '**secondary settlement**' (i.e. long-term creep) may occur where slow long term changes in the soil structure take place, (e.g. plastic deformation of soil particles). Secondary settlement is normally relatively small and is often ignored. **Drainage and consolidation** Consolidation only occurs as water moves out of the soil, and so the rate at which this happens will govern the rate of consolidation. Hence, high permeability soils in **drained** conditions (i.e. where seepage can easily occur) will consolidate relatively rapidly, and soils in **undrained** conditions (i.e. very limited or no seepage) will consolidate very slowly (or not at all). Loading of a soil increases the total stress (σ) in the soil, which (in the very short term) results in an increase in the pore water pressure (u) in the soil. In drained conditions, this increase in pore water pressure very rapidly results in seepage as water is forced out of the soil. The soil particles thus become more closely packed because water movement out of the soil results in volume reduction of the soil. As this happens the increased total stress in the soil is transferred from the pore water to the framework of soil particles. Thus the effective stress, σ' (the stress passing through the soil framework or 'skeleton'), will increase. (Note: any increase in effective stress in a soil will result in an increase in its strength). For soils in undrained conditions, there is no or little water movement, meaning that there is no, or very slow, volume reduction. As a result, the increase in total stress on the soil does not lead to a quick increase in the effective stress of the soil, and thus the strength of the soil does not increase. For unconfined soils, the permeability of the soil will thus govern the rate of consolidation. **Normally- and over-consolidated clays** An **over-consolidated** clay refers to one which has, during its geological history, been subjected to a higher consolidating stress than it is under at present. (See previous module information for further information on 'over-consolidated' soil). Thus, as the soil has been previously consolidated under a higher stress than exists at the moment, then if the current stress is increased it may not cause a significant increase in the amount of consolidation (because it has, in effect, already been consolidated by the higher stress it was previously under, known as the 'pre-consolidation' stress). Only when the current applied stress is greater than the maximum previously applied stress will the soil consolidate as expected (i.e. behave as a **normally consolidated** soil). **Predicting the amount of consolidation** To express the amount of one-dimensional consolidation (i.e. the vertical downwards movement of the soil) we use need to know the ***coefficient of (volume) compressibility*** (m~v~) of the soil. This can be determined from laboratory tests (as will be conducted in soils lab 3, using an oedometer) where samples of soil are subjected to an applied stress and their subsequent reduction in height is recorded: ΔH = change in thickness of soil sample, caused by the increase in stress applied to the sample Δσ Once m~v~ has been determined from lab testing of soil samples, the same equation can be re-arranged to be used to determine the change in in-situ soil layer thicknesses on site, as a result of an applied stress: So, to calculate in-situ settlement amount (ΔH) we need to know the thickness of the soil layer on site, H~i~ (determined from a ground investigation), the in-situ increase in applied stress on the soil layer (Δσ) and the coefficient of compressibility (m~v~) for the given soil (i.e. determined from the lab test on the soil). Note: On its own, the coefficient of compressibility (m~v~) does not tell us how much a soil will consolidate: it is only when it is used in the consolidation equation above that it provides a useable parameter (ΔH) for the consolidation of a layer of soil.