Week 1 study guide balance.docx
Document Details
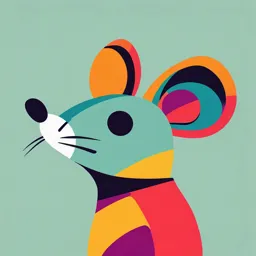
Uploaded by LowCostMeter2369
Columbus State Community College
Tags
Full Transcript
**[Water, Electrolyte, and Acid-Base Balance]** **"The cells of the body live in a fluid environment that requires electrolyte and acid-base concentrations maintained within a very narrow range. A balance is maintained by an integration of renal, hormonal, and neural functions."** **Changes in the...
**[Water, Electrolyte, and Acid-Base Balance]** **"The cells of the body live in a fluid environment that requires electrolyte and acid-base concentrations maintained within a very narrow range. A balance is maintained by an integration of renal, hormonal, and neural functions."** **Changes in the composition of:** - **Electrolytes affect electrical potentials of excitatory cells and cause shifts of fluid from one compartment to another that can affect cell function.** - **Fluid fluctuations affect blood volume and blood pressure.** - **Alterations in pH (measure of the acidity or alkalinity of a solution) disrupt the cellular function of enzyme systems and can cause cell injury.** **"Understanding how alterations occur and the body's ability to compensate or correct the disturbance is important for comprehending many pathophysiologic conditions." (McCance & Huether, 2014).** **Normal distribution of fluids** **Compartments** **Intracellular fluid (ICF) -- the "first space"** **Extracellular fluid (ECF) consisting of:** **Intravascular fluid -- the "second space"** **Interstitial fluid -- the "third space"** **Other -- mesothelial-lined spaces (pleura, pericardium, peritoneum), synovial spaces, CSF, glands, etc.** **Intracellular (ICF)** **60-70% of body water is in the cells** **40% of body weight in ICF** **Extracellular (ECF)** **Intravascular** **5% of body weight** **Intersitital** **15% of body weight** **Transcellular** **GI tract; cerebrospinal fluid; pleural, synovial and peritoneal fluid** **Disease processes -- those involving fluid shifts, electrolyte and acid-base disorders: *fluid compartments and normal distribution. Factors causing fluid shifts, electrolyte imbalances, acid-base imbalances*** **Body fluids are distributed among physiologic spaces and provide a transport medium for cellular and tissue function. Water moves among body compartments and is controlled by osmotic and hydrostatic forces. Sixty-seven percent of the body\'s water is intracellular fluid (ICF) and thirty-three percent is in the extracellular fluid (ECF). There are two ECF compartments - interstitial fluid and the intravascular fluid (blood plasma). Smaller ECF compartments include the lymph and the transcellular fluids -- cerebrospinal fluid (CSF), bile, joint fluid, intraocular fluid, pleural fluid, peritoneal fluid, digestive secretions, and bladder urine.** **The sum of the fluids in the fluid compartments is the total body water (TBW). The volume of TBW is a percent of body weight in kilograms. For example, the total volume of body water for a 70-kg person is about 42 liters. (McCance et al., 2019).** **Water Content** **Men have more water than women** **Lean body mass has more water than fat body mass** **The older you are the less water you have in your body** **Infants---70-80% water** **Adults---50-60% water** **Older adults---45-55% water** **Infants and the elderly are at the most risk for fluid-related problems** **FUNCTIONS OF BODY WATER** **Transport** **Nutrients, electrolytes, oxygen, waste products** **Regulates body temperature** **Lubricates** **Joints and membranes** **Medium for food digestion** **Water is the primary component of our bodies** **It is the solvent in which salts, nutrients and waste products are dissolved and transported** **Notice to achieve fluid balance water intake must equal water loss.** **Water intake averages 2500 mL/day** **Sources of water include:** - **Drinking water** - **Moisture in food** - **Water produced in metabolic processes, such as cellular respiration** **Water Output averages 2500 mL/day** **Most water is lost in urine, exhaled while breathing, evaporated from the skin, and small losses in feces and sweat.** **Regulation of Intake** - **Main regulator is thirst.** - **Dehydration (output\>intake) as little as 1% decrease in body water causes:** - **Decreased production of saliva** - **Increased blood osmotic pressure -- stimulates osmoreceptors in the hypothalamus -- ADH produced** - **Decreased blood volume -- RAAS stimulated The thirst center in hypothalamus is stimulated ( or mistakenly, the hunger center) and person feels thirsty** - **Wetting of the mouth and stretching of stomach or intestines decrease thirst before we take in too much water.** - **Water is absorbed, and blood osmotic pressure decreases.** **Regulation of Output** - **Through regulating urine concentration/dilution** - **ADH -- production stimulated by ↑ blood tonicity or decrease in volume.** - **Acts on distal convoluted tubules and collecting ducts of kidney -- permits reabsorption of water** - **Through regulating urine volume** - **ANP -- causes sodium (and water) loss when pressure in right atrium is too high** - **BNP \-- Causes sodium (and water) loss when pressure** - **in the left ventricle is too high** - **Aldosterone -- production is stimulated by angiotensin II through renin production** - **Causes sodium ( and water) to be reabsorbed** ***Water* balance of the body** **ADH mechanism. Osmoreceptors in hypothalamus cause ADH release if osmolarity rises. Additionally ADH produced if volume or pressure falls (receptors in atria and baroreceptors in large arteries of chest and neck) Acts on collecting duct of nephron to increase water retention. Also increases thirst and casues drinking behavior.** ***Salt and water* balance of the body (circulating volume control)** **Renin-Angiotensin-Aldosterone mechanism** **Although we are used to blaming this mechanism for high blood pressure in renal disease or established hypertension, it is a normal homeostatic mechanism that is appropriately triggered by reduced renal blood flow in dehydration or hypotension, and acts to restore blood pressure/volume by the actions of angiotensin and aldosterone.** **Atrial Natriuretic Protein, Brain Natriuretic Protein** **Are produced by the heart when it is stretched, and counteract the RAA mechanism by causing natriuretic ("diuresis of sodium")** **Symptoms of dehydration:** **Headache, thirst, dry skin and mucous membranes, elevated temperature, weight loss, and decreased or concentrated urine (with the exception of diabetes insipidus). Skin turgor may be normal or decreased.** **Symptoms of hypovolemia:** **Tachycardia, weak pulses, and postural hypotension** **REGULATION OF WATER BALANCE** **Pituitary regulation** **Hypothalamus senses plasma osmolality** **Increased osmolality---water deficit** **Stimulates thirst---client drinks more water** **Secretion of antidiuretic hormone (ADH) from the posterior pituitary** **Acts on renal distal and collecting tubules to cause water reabsorption; decreased urine output** **Decreased osmolality---water excess** **Decreased thirst** **inhibit secretion of ADH** **Adrenal cortical regulation** **Anterior pituitary secretes ACTH in response to physiologic stressor---water deficit** **Stimulates cortisol secretion from adrenal cortex** **Increased glucose production** **Decreased renal perfusion---volume deficit** **Causes kidney secretion of renin** **Renin converts angiotensin I to angiotensin II** **Angiotensin II stimulates aldosterone secretion by the adrenal cortex** **Aldosterone acts on renal tubules to increase Na^+^ reabsorption and K^+^ excretion** **When Na^+^ is retained, water is also reabsorbed; resolving the water deficit** **Renal regulation** **Kidneys regulate water balance by adjusting the volume of urine** **Cardiac regulation** **Atrial natriuretic factor (ANF) release from the atria is stimulated by increased blood volume/ pressure.** **ANF causes vasodilation, and increased excretion of Na^+^ and water to decrease blood volume** **Water loss** **GI sources** **Insensible water loss---lungs and skin** **EXAMPLE:** **Water toxicity** - **If we lose water by sweating, we also lose sodium.** - **Rapidly drinking large quantities of water decreases plasma sodium concentration initially, then see decrease in ISF as well.** - **Water is drawn into cells -- intracellular edema** - **This increases ISF tonicity, and water is drawn from blood** **Water balance is regulated by two mechanisms: pressure sensing organs (carotid and aortic artery volume/pressure receptors) and hypothalamic osmoreceptors (osmolality). These mechanisms affect the secretion of antidiuretic hormone (ADH) and water reabsorption by the kidney. ADH is secreted when plasma osmolality increases or circulating blood volume and blood pressure decrease. Increased plasma osmolality (solutes per mL) occurs when there is a water deficit or sodium excess related to total body water. The increased osmolality stimulates hypothalamic osmoreceptors and signals the posterior pituitary to release ADH. The action of ADH is to increase the permeability of the distal nephron to water, increasing water reabsorption, which increases intravascular volume, and promotes the increase in plasma volume and blood pressure. Urine concentration increases, and the reabsorbed water decreases plasma osmolality toward normal which then inhibits ADH secretion. ADH is controlled by a negative feedback loop related to plasma osmolality.** **As plasma osmolality increases, ADH increases, stimulating the kidneys to save water. When osmolality then decreases to normal, ADH secretion is inhibited.** **The second mechanism involves dehydration, with fluid losses from vomiting, diarrhea, or excessive sweating, and a decrease in blood volume and blood pressure. Baroreceptors (volume/pressure sensitive receptors) are stretch receptors that are sensitive to changes in arterial volume and are located in the right and left atria and large veins, and in the aorta, pulmonary arteries, and carotid sinus. When arterial and atrial pressure decreases, baroreceptors have less stretch and signal the hypothalamus to release ADH. ADH then promotes the reabsorption of water by the kidneys, promoting the restoration of plasma volume and blood pressure. Higher concentrations of ADH stimulate peripheral arterial vasoconstriction, thus increasing arterial blood pressure further. (McCance et al., 2019).** **Tonicity - In the human body, tonicity is the ability of the solution on one side of the cell membrane to attract water from the solution on the other side of the cell membrane. Normally, solutions inside and outside the cells have the same ability to attract water, so they are isotonic. See the container in the center of the graphic.** **Osmolality - The movement of water through the membrane from a solution with lower tonicity to a solution with higher tonicity is called osmosis. Substances that increase tonicity of solutions and thus trigger osmosis are called osmotically active substances. Sodium is the main osmotically active substance in the extracellular fluid and its amount can change significantly in dehydration. The measure of the amount of osmotically active substances in the solution is osmolality, which is expressed in milliosmoles of a solute per kilogram of water (mOsm/L). The normal range of the blood plasma osmolality is 285-295 mOsm/kg.** - **When dehydration does not affect the concentration of sodium in the extracellular fluid, it is called isonatremic dehydration or isotonic or iso-osmolar dehydration.** - **When dehydration results in an increased sodium concentration of the extracellular fluid, it is called hypernatremic dehydration or hypertonic or hyperosmolar dehydration.** - **When dehydration results in a decreased sodium concentration of the extracellular fluids, it is called hyponatremic or hypotonic or hypoosmolar dehydration.** **So Tonicity is the concentration of particles per volume of solution. Pictures so the different particle concentrations for hypo-, iso- and hyper-tonicity.** **CONTROLLING FLUID AND ELECTROLYTE MOVEMENT** **Diffusion---movement of molecules or electrolytes from an area of high concentration to an area of low concentration. Molecules move [down] the concentration gradient.** **Liquids, solids and gases** **Movement stops when solutes are equal on in both areas** **Membrane permeable to the diffusing substance** **Requires no energy to do.** **Facilitated Diffusion---diffusion accomplished by combining the diffusing molecule with a carrier molecule** **The membrane is impermeable to the diffusing molecule without the carrier** **Example: Glucose requires insulin to be transported into cells** **Active transport---Molecules or electrolytes move [against] the concentration gradient. They are transported into the cells from an area of lesser concentration to an area of higher concentration.** **Requires energy---adenosine triphosphate (ATP); produced in mitochondria.** **Example: Sodium-potassium pump---K^+^ is pumped into the cells and Na^+^ is pumped out of the cells; maintains the high ECF Na^+^ and ICF K^+^.** **Osmosis** **Osmosis is the primary method of *water* movement into and out of body fluid compartments.** **Osmosis is the net movement of water molecules through a selectively permeable membrane from an area of higher water concentration to an area of lower water concentration.** **The concentration of solutes determines the direction of water movement.** **CONTROLLING FLUID AND ELECTROLYTE MOVEMENT** **Osmosis---Movement of water between to compartments** **Membrane is permeable to water, but not to solutes** **Water moves from an area of low solute concentration to an area of high solute concentration; [water moves from the more dilute area to the more concentrated area]** **Movement stops when concentrations are equal; or when hydrostatic pressure becomes greater than osmotic pressure** **No energy required** **Osmotic pressure---amount of pressure required to stop osmotic flow of water** **Hydrostatic pressure---pressure generated by the fluid column; force of the fluid within a compartment** **Example: Blood pressure** **Osmotic pressure or colloid osmotic pressure---osmotic pressure exerted by proteins (colloids) in solution** **Protein molecules attract water, and pull fluid into the compartment they occupy** **Example: Plasma proteins will draw fluid into the IVF** **MEASURE OF OSMOTIC PRESSURE** **Osmolality---measures the osmotic force of solute per weight of the solvent; total solute concentration per kg of body water; pertains to fluids inside the body** **Osmolarity---measures total solute concentration per liter of solution; pertains to fluids outside the body** **So -- ions, proteins, glucose have the same ability to produce osmotic pressure.** **OSMOTIC MOVEMENT OF FLUID** **Fluid moves in and out of cells based on the concentration of the surrounding extracellular fluid** **Isotonic---surrounding fluid is the same concentration as the interior of the cells -- little or no movement of fluid between IC and EC fluid spaces** **Hypotonic---surrounding fluid is less concentrated than the fluid in the cells -- fluid moves from ECF space to ICF space, causing cell swelling and potentially cell rupture** **Hypertonic---surrounding fluid is more concentrated than the fluid in the cells -- fluid moves from ICF space to ECF space, causing cells to shrink** **What controls movement of fluid between the intracellular and extracellular compartment (through cell membrane)?** **Osmosis. The main factors in determining the osmotic balance are K+ and proteins (inside the cell) and Na+ (outside the cell). Should any imbalance exist, water will move through the cell membrane to re-establish equilibrium. If the cell is injured, and can not maintain its Na+/K+ pumps allowing Na+ to leak into the cell this translates to "where sodium goes water follows". Increased water in the cell may damage it further.** **Glucose, or calcium in excess in the extracellular fluid (diabetic coma, or hypercalcemia of cancer) also disrupt the osmotic balance, this time drawing water out of the cell and causing cellular dehydration. Rapid and large scale movement of fluid into or out of cells is damaging, especially to brain cells.** **MEASURE OF OSMOLALITY -- inside the body** **Indicator of body water balance** **Normal plasma omolality---275 to 295 mOsm/kg** **Greater than 295---water deficit** **Less than 275---water excess** **Determinants of plasma osmolality** **Na^+^, glucose, and urea** **Effective osmolality = 2(Na^+^) + Glu/18** **Can add urea into the equation** **2(Na^+^) + Glu/18 + BUN/2.8 = serum osmolality** **Overhydration -- More fluid intake than fluid output. Occurs with rapid infusion of large amounts of IV fluid. The increased intravascular volume, increases the workload on the heart due to increased preload (volume returning to the heart via the venous system), requiring the heart to pump harder and faster to pump the increased volume. Hear workload is also increased by increased afterload, increased arterial diastolic pressure, or the resistance the LV must pump against to eject blood into the aorta.** **Dehydration -- More fluid output than fluid intake. Occurs with excessive fluid losses, such as diarrhea, vomiting and sweating.** **CALCULATING FLUID BALANCE** **One liter of water weighs 2.2 lbs or 1 kg** **Sudden body weight change measures fluid gain or loss** **Example:** **A patient receiving diuretic therapy loses 3 kg or 6.6 lbs, he has lost 3 liters of fluid from the diuresis** **Dehydration Types** **Isosmotic -- Bleeding, Plasma exudate thru burned skin** **Hyperosmotic -- DI (ADH Loss), Decreased Intake** **Hypo-osmotic -- Renal Na+ Loss (Adrenal Insufficiency, where Na+ goes so does water)** **Dehydration is water deficiency in the body. In dehydration, fluids from the blood and the space between the cells (together called extracellular space) are lost first, which is followed by loss of fluid from within the cells (intracellular space). Dehydration can be categorized into isotonic, hypertonic and hypotonic, depending on how it affects the *tonicity* of the extracellular fluids.** **Why is it important to know if dehydration is iso-, hyper- or hypotonic? Because this can suggest the cause of dehydration and because hypotonic dehydration must be treated with great caution to avoid severe neurologic damage. (eHealthstar, 2015). http://www.ehealthstar.com/dehydration/types-pathophysiology** **Isotonic alterations involve proportional changes in water and the concentration of electrolytes. *Isotonic fluid loss* causes dehydration and hypovolemia, but the number and type of electrolytes and the osmolality of the blood remain within a normal range (280 to 294 mOsm). Causes include: hemorrhage, excessive wound drainage, and sweating. Clinical manifestations of loss of extracellular volume include: weight loss, dry skin and mucous membranes, decreased urine output, increased hematocrit, tachycardia, flattened jugular veins, and hypotension. (McCance et al., 2019).** **Hypertonic fluid alterations develop when the osmolality of the ECF is elevated higher than normal (greater than 294 mOsm). Causes include: hypernatremia -- increased sodium or decreased ECF water, or both. The hypertonicity of the ECF attracts water from the intracellular space, causing ICF dehydration and ECF hypervolemia. In contrast, a hypertonic state caused primarily by free water loss leads to *hypovolemia.* (McCance et al., 2019).** **Hypotonic fluid alterations occur when the osmolality of the ECF is less than normal (less than 280 mOsm). The cause is hyponatremia from free water excess, which leads to intracellular edema and cell swelling because water moves into the cell from the extracellular space. Cerebral and pulmonary edema are common as fluid moves into the cells, and the plasma volume decreases, leading to hypovolemia. (McCance et al., 2019).** **Overhydration** **Abnormal excess of ECF volume that results from an imbalance among fluid compartments controlled by diffusion (particles) and osmosis (water).** **Isotonic fluid excesses from proportional water and sodium gains results in hypervolemia (ECF) and weight gain. Causes include: excessive intravenous fluid infusion, hypersecretion of aldosterone, the effects of drugs such as cortisone, or failure of the kidneys. The diluting effect of excess plasma volume leads to decreased hematocrit and decreased plasma protein concentration. Clinical manifestations: Jugular veins distension, increased blood pressure and edema formation. (McCance & Huether, 2019).** **Hypertonic fluid alterations develop when the osmolality of the ECF is elevated higher than normal (greater than 294 mOsm) resulting from more sodium gain than water gain. Causes include: hypernatremia or a deficit of ECF free water, or both. The hypertonicity of the ECF attracts water from the intracellular space (osmosis), causing ICF dehydration and hypervolemia of ECF (McCance & Huether, 2019).** **Hypotonic fluid alterations occur when the osmolality of the ECF is less than normal (less than 280 mOsm) resulting from more water gain than sodium gain. Causes include: hyponatremia or water excess (water intoxication), renal failure and excess ADH. The ECF hypotonicity produce intracellular overhydration (cellular edema) and cell swelling when water moves into the cell producing ECF hypovolemia (McCance & Huether, 2019).** **What controls movement of fluid between plasma and interstitial space (through capillary wall)?** **Blue = transcellular fluid -- pleural, intraocular, pericardial, etc.** **Green = lymphatic** **Yellow = Plasma -- intravascular space** **Teal = Interstitial space (IV)** **Tan = intracellular space (IS)** **Hydrostatic pressure in blood vessels, tends to force fluid out of the capillaries into the interstitial space** **Oncotic pressure produced by osmotic forces of plasma proteins tend to pull fluid from the interstitial space back into the capillaries** **Starling's hypothesis -- The net filtration is determined by forces favoring filtration *minus* forces opposing filtration. Controls fluid movement between the IV space and the IS space** **The hydrostatic pressure is greater at the arterial portion of capillaries leading to filtration, and oncotic pressure is greater at the venous portion leading to fluid return to the IV space. But not all fluid is returns to the blood; some stays out and is collected by lymphatics** **BALANCING FLUID MOVEMENT BETWEEN CAPILLARIES AND INTERSTITIUM** **The distribution of water and the movement of nutrients and waste products between the plasma (IV space) and interstitial spaces (IS) occur due to a balance between hydrostatic pressure and osmotic forces at the arterial and venous ends of the capillaries. Water, sodium, and glucose move readily across the capillary membrane. Plasma proteins, primarily albumin, maintains the effective osmolality (concentration of solutes per kilogram of solution), and do not cross the capillary membrane. This is what produces oncotic pressure.** **Capillary osmotic forces are balanced by the intravascular hydrostatic pressure, which is determined by blood pressure and blood volume.** **As plasma flows from the arterial to the venous end of the capillary, four forces determine fluid movement out of the capillary and into the interstitial space (filtration) and fluid movement back into the capillary from the interstitial space (reabsorption):** **Forces that move fluid out of the capillaries** **Capillary hydrostatic pressure -- pushed fluid out of the capillaries** **Interstitial oncotic pressure -- pulls fluid out of the capillaries** **Forces that move fluid into the capillaries** **Capillary oncotic pressure -- pulls fluid back into the capillaries** **Interstitial hydrostatic pressure -- pushes fluid into the capillaries** **At arterial end of the capillary** **Capillary hydrostatic pressure is greater than plasma oncotic pressure, fluid is pushed out of the capillaries** **At venous end of the capillary** **Capillary hydrostatic pressure is less than plasma oncotic pressure, fluid is pulled back into the capillaries** **FLUID SHIFTS** **Shifts of fluid from plasma to interstitum---edema** **Rise in capillary hydrostatic pressure** **CHF, fluid overload, liver failure, obstruction of venous return, venous insufficiency** **Decrease in plasma oncotic pressure** **Excess protein loss (renal failure), decreased protein synthesis (liver disease), insufficient protein intake (poor nutrition).** **Increase in interstitial oncotic pressure** **Allow plasma proteins to accumulate in the interstitum\--burns, trauma, sepsis** **Shifts of fluid from interstitum to plasma---decrease edema** **Increase plasma oncotic pressure** **Administration of colloids---dextran, mannitol or hypertonic solutions** **Increase interstitial hydrostatic pressure** **Elastic stockings or sequential compression devices** **The overall effect is filtration at the arterial end and reabsorption at the venous end. Abnormalities in the capillary membrane, such as increased permeability, can alter the normal effects of fluid movement. For example, loss of capillary membrane integrity may allow the escape of plasma proteins into the interstitial space, increasing interstitial oncotic pressure, trapping fluid in the interstitium, and producing tissue edema (McCance & Huether, 2019).** **Mechanisms of Edema Formation** **Alterations in fluid balance** **Edema -- excess fluid in tissues as fluid moves from the vascular compartment. Distensible or loose tissues (such as lung) are most affected. Pulmonary edema = fluid in alveoli. Generalized edema of the whole body is called anasarca. Another term is hydrops, as in hydrops fetalis.** **Examples of causes of edema --** ***Increased capillary hydrostatic pressure* leading to increased filtration of fluid.** - **increased systemic venous pressure in right heart failure** - **increased pulmonary venous pressure in left heart failure** ***Decreased plasma proteins* leading to reduced oncotic pressure of blood.** - **reduced plasma protein synthesis in liver disease** ***Lymphatic obstruction*.** - **removal of lymph nodes** ***Increased permeability of capillary wall* as in inflammation (not only seen in infections, but in any cause of tissue injury).** - **Remember the five cardinal features of inflammation are "tumor" in the sense of mass (edema), calor (heat), rubor (redness), dolor (pain), and altered function.** - **EXAMPLE -- Intracellular dehydration** - **Increased ECF osmolality---water deficit or increased solute load** - **Pulls fluid out of the cells, cells shrink, until osmolality is similar** - **Mannitol for increased intracranial pressure** - **Diabetic coma: Plasma glucose is very high and is increasing the osmotic value of the blood. Water is pulled out of the brain cells by the hypertonic blood. The neurons malfunction and the person loses consciousness** **MOVEMENT OF FLUID FROM ECF to ICF** **Decreased ECF osmolality---water excess** **Fluid moves into the cells, the cells swell** **Confusion and altered level of consciousness with hyponatremia** **Electrolyte imbalances** **Sodium** **Hypernatremia** **Can be true gain of sodium (IV hypertonic saline or sodium bibcarb, excess aldosterone) or loss of water from ECF (inadequate intake causing hyperosmolarity)** **Hyponatremia** **Inability to excrete water (heart failure, renal failure), post head injury or surgery (SIADH), hypotonic IV fluids. Causes cell swelling and can be fatal** ** - hyponatremia after ecstasy** **Potassium** **Hypokalemia -- serum K+ less than 3.5meq-L as a result of reduced intake, increased entry into cells or increased loss as from diarrhea or diuretic use.** **Hyperkalemia -- less common but seen with acidosis, renal failure.** **Calcium** **Hypocalcemia -- nutritional causes, decreased PTH or vitamin D.** **Hypercalcemia -- increased PTH from parathyroid adenoma or other tumors producing PTH, bone destruction from bone metastases (breast and prostate cancers are common causes), sarcoidosis** **Both calcium and potassium are extremely important in nerve and muscle excitability, and both high and low values have fatal cardiac and brain effects.** **ELECTROLYTES** **Split into ions in solution (body water)** **Ions---electrically charged particles** **Cations---positive charge** **Na^+^. K^+^, Ca^2+^, Mg ^2+^** **Anions---negative charge** **Cl^-^, HCO~3~^-^, and PO~4~^3-^, proteins** **When ions combine to make compounds, they combine to make the substance electrically neutral** **NaHCO~3~, KCl, MgSO~4~, NaCl** **MEASURING ELECTROLYTES** **mg/dl---milligrams per deciliter** **mmol/L---millimoles per liter** **Millimoles---atomic weight of a substance in milligrams; attomic weight of Na is 23, so a millimole of Na is 23 mg.** **mEq/L---milliequivalents per liter** **Concentration of the electrolyte in solution** **Measure of chemical activity---more practical to use that the measures of weight** **Electrolyte Balance is a function of electrolyte gains through food and fluid ingestion and input from metabolic processes, and electrolyte losses from sweating, urine and stool.** **Sodium is 90% of the ECF cations (positively charged ions), and with its anions (negatively charged ions) chloride and bicarbonate, regulates extracellular osmotic forces, water balance, and ECF volume. Sodium is also important for conducting nerve impulses, regulation of acid-base balance, participation in cellular chemical reactions, and transport of substances across the cell membrane.** **Normal serum sodium concentration (135 to 145 mEq/L) is maintained through reabsorption by the kidneys, which is controlled by aldosterone (a mineralocorticoid secreted by the adrenal cortex) and the RAAS. The RAAS is activated when renal blood flow, or serum sodium concentrations, are reduced \> Renin isecreted by the juxtaglomerular cells of the kidney \> stimulates the formation of angiotensin I \> In the lungs, angiotensin-converting enzyme (ACE) converts angiotensin I to angiotensin II \> Angiotensin II causes vasoconstriction, increasing blood pressure and stimulating the secretion of aldosterone \> Aldosterone promotes sodium and water reabsorption by the proximal tubules of the kidneys \> conserves sodium, increasing blood volume and blood pressure \> Aldosterone also increases excretion of potassium by the distal tubule of the kidney \> reducing potassium concentrations in the ECF \> When sodium levels, blood volume, and renal perfusion return to normal \> then release of renin is inhibited. (McCance & Huether, 2019).** **Natriuretic peptides are hormones that include:** - **atrial natriuretic peptide (ANP) produced by myoendocrine atrial cells** - **brain natriuretic peptide (BNP---named brain since it was first discovered in porcine brain) produced by myoendocrine ventricular cells,** - **urodilatin (renal natriuretic peptide, an ANP analog) synthesized within the kidney** **ANP and BNP are released when there is an increase in atrial pressure/volume, as seen in heart failure. ANP and BNP increase sodium and water excretion by the kidneys, which lowers blood volume and pressure.** **Urodilatin is released from distal tubular kidney cells when there is increased arterial pressure and increased renal blood flow. These hormones are antagonists to the renin-angiotensin-aldosterone system (RAAS). When atrial pressure is lowered, then the release of ANP and BNP is inhibited (McCance & Huether, 2019).** **Hypernatremia** **Hypernatremia occurs when serum sodium levels exceed 145 mEq/L, causing hypertonicity. Hypernatremia can be hypovolemic, euvolemic, or hypervolemic depending on the ECF water volume. These electrolyte imbalances are produced by the relative amounts of body sodium compared to body water.** **Hypovolemic hypernatremia occurs where there is a greater loss of body water compared to loss of body sodium. Causes include:** - **use of loop diuretics,** - **osmotic diuresis (from hyperglycemia related to uncontrolled diabetes mellitus),** - **gastrointestinal losses,** - **failure of the kidneys to concentrate urine.** **Euvolemic hypernatremia occurs when there is a *loss of free water* and no sodium losses. Causes include:** - **inadequate water intake;** - **excessive sweating;** - **fever with hyperventilation and increased water loss from lungs;** - **burns; vomiting; diarrhea;** - **diabetes insipidus (lack of ADH or inadequate renal response to ADH).** **Insufficient water intake occurs particularly in infants, comatose, confused, immobilized, or receiving gastric feedings. ** **Hypervolemic hypernatremia occurs when there is a greater increase in total body sodium level compared to an increase in total body water, resulting in hypervolemia. Causes include:** - **infusion of hypertonic saline solutions (e.g., because sodium replacement for treatment of salt depletion can occur with renal impairment, heart failure, or gastrointestinal \[GI\] losses)** - **oversecretion of adrenocorticotropic hormone (ACTH) or aldosterone (e.g., Cushing syndrome, adrenal hyperplasia)** - **near--salt water drowning.** **Symptoms include weight gain, bounding pulse, and increased blood pressure.** **Because chloride follows sodium, hyperchloremia (elevation of serum chloride concentration greater than 105 mEq/L) often accompanies hypernatremia, as well as plasma bicarbonate deficits, as in hyperchloremic metabolic acidosis (McCance & Huether, 2019).** **Hyponatremia** **Hyponatremia occurs when the serum sodium concentration decreases to less than 135 mEq/L, caused by loss of sodium, inadequate intake of sodium, or dilution of sodium by water excess. Hyponatremia can be classified as hypovolemic, euvolemic, or hypervolemic when there are changes in intravascular volume or as hypotonic, isotonic, or hypertonic when there are changes in osmolality.** **Hypovolemic hyponatremia occurs when there is a greater loss of body sodium compared to the loss of total body water. The extracellular volume is decreased. Causes include:** - **prolonged vomiting,** - **severe diarrhea,** - **inadequate secretion of aldosterone (e.g., adrenal insufficiency)** - **renal losses from diuretics.** **Euvolemic hyponatremia occurs when there is loss of sodium without a significant loss of water (pure sodium deficit and a hypotonic hyponatremia). Causes include:** - **syndrome of inappropriate antidiuretic hormone (SIADH) -- water retention** - **hypothyroidism,** - **pneumonia** - **glucocorticoid deficiency. ** - **Inadequate intake of dietary sodium in individuals consuming low-sodium diets, particularly with use of diuretics.** **Dilutional hypotonic hyponatremia (water intoxication) occurs when there is intake of large amounts of free water. Causes include:** - **when excessive sweating stimulates thirst and intake of large amounts of free water, which dilutes sodium concentration.** - **psychogenic disorders develop water intoxication from compulsive water drinking.** - **tap water enemas,** - **near--fresh water drowning,** - **use of selective-serotonin reuptake inhibitors (SSRIs)** - **SIADH.** **When the body is functioning normally, it is almost impossible to produce an excess of TBW because water balance is regulated by the kidneys.** **Hypervolemic hyponatremia occurs when both TBW and sodium levels are increased, but TBW exceeds the increase in sodium levels. Causes include:** - **congestive heart failure,** - **cirrhosis of the liver** - **nephrotic syndrome.** **Edema is present.** **Hypertonic hyponatremia develops with the shift of water from the ICF to the ECF, as occurs with hyperglycemia, hyperlipidemia, and hyperproteinemia. The ECF is hypertonic from elevated glucose, lipids or plasma proteins compared to ICF, which promotes osmosis of water into the ECF, which then dilutes the concentration of sodium (pseudohyponatremia) and other electrolytes (McCance & Huether, 2019).** **Potassium (K^+^) is the most abundant intracellular electrolyte and is also found in small amounts in ECF. The ICF concentration of K^+^ is about 150 to 160 mEq/L; the ECF concentration is about 3.5 to 5.0 mEq/L** **K+ levels primarily regulated by the Na^+^ /K^+^ pump -- moving K+ into the ICF and preventing accumulation of K+ in ECF** **Kidneys also assist in significant regulation -- aldosterone promotes Na+ retention and K+ excretion** **Normal physiologic process of aging ⇓ ability to concentrate urine ⇑ K^+^ loss** **Physiologic functions of potassium (K^+^)** - **Regulation of intracellular osmolarity -- most abundant cation in ICF** - **Regulation of protein synthesis** - **Regulation of glucose & storage - potassium helps in transporting the glucose through the cell membrane** - **Maintenance of action potentials in excitable membranes of neurons and muscle cells (skeletal, smooth, and cardiac)** - **Maintenance of plasma acid-base balance -- H+/K+ exchange between ECF and ICF as a first line buffer for acidosis or alkalosis** **HYPOKALEMIA** **K^+^ \< 3.5 mEq/L** **Causes:** **Cellular damage---trauma, injury, surgery** **Vomiting and diarrhea** **Losses from medications** **Laxatives** **Antibiotics** **Insulin and glucose** **Alpha adrenergic blockers** **Beta~2~ agonists** **Potassium wasting diuretics** **S&S** **N&V** **Dysrhythmias** **Abdominal distension** **Muscle weakness** **HYPERKALEMIA** **K^+^ \> 5.3 mEq/L** **Causes:** **Renal insufficiency/failure** **massive trauma** **insulin deficiency** **Addison disease** **use of potassium salt substitutes** **metabolic acidosis.** **Medications** **Excess K^+^ replacement** **K^+^ sparing diuretics** **ACE inhibitors** **Beta1 blockers** **NSAIDS** **Immunosuppressive drugs** **Heparin** **S&S** **N&V** **Abdominal cramps** **Oliguria** **Bradycardia** **Muscle weakness** **Numbness and tingling** **Hypokalemia occurs when the serum K^+^ concentration decreases to less than 3.5 mEq/L. Usually, lowered serum K^+^ level indicates a loss of total body K^+^, because K^+^ is lost from the ECF. There is a change in the concentration gradient that favors movement of K^+^ out of the cell into the ECF. This depletes IC K+, and although the amount of EC K+ is maintained, the amount of total body K^+^ is depleted. Therefore, EC K+ levels (serum K+ in a BMP) may not indicate the total body K+ level -- you cannot measure IC K+.** **Factors for the development of hypokalemia include reduced intake of potassium, increased entry of K^+^ into cells, and increased losses of body K^+^. Metabolic imbalances can produce K+ deficits:** - **Respiratory alkalosis -- Hydrogen ions (H+) move out of the cell to correct the alkalosis (lack of H+), which causes K^+^ to move into the cell to maintain an ionic balance, decreasing EC K+.** - **Insulin also promotes cellular uptake of K^+^ and can cause an ECF potassium deficit, particularly with the intake of high carbohydrate loads. Severe, even fatal, hypokalemia may occur if insulin is administered without also providing K^+^ supplements.** - **Diabetic ketoacidosis, where there is increased hydrogen ion concentration in the ECF (acidosis), H^+^ shift into the cells in exchange for K^+^. The mechanism reduces EC H+ to compensate for the metabolic acidosis. At the same time osmotic diuresis is occurring from hyperglycemia, and the EC K+ is lost in the urine. So, the EC K+ gain from the H+/K+ shifts is countered by K+ loss in the urine and serum K+ level is normal, although total body K^+^ is depleted.** - **GI and renal disorders also cause potassium losses. Diarrhea, intestinal drainage tubes or fistulae, and laxative abuse also may result in EC K+ losses and hypokalemia. Vomiting or nasogastric suction depletes potassium, due to K^+^ lost from the gastric fluid. However, volume depletion and the metabolic alkalosis from gastric losses cause sodium, chloride, and hydrogen ion losses. These fluid and sodium losses stimulate aldosterone and ADH secretion (water and Na+ is reabsorbed and K+ is lost by the kidneys).** - **Increased secretion of K^+^ by the distal tubule is the cause of renal K+ losses. Causes include:** - **Use of diuretics,** - **excessive aldosterone secretion,** - **increased distal tubular flow rate,** - **low plasma magnesium concentration** - **Primary hyperaldosteronism with excessive secretion of aldosterone from an adrenal adenoma (McCance & Huether, 2019).** **Hyperkalemia** **ECF potassium level of *greater than 5.0 mEq/L* is hyperkalemia. Excess serum K^+^ is caused by: ** - ***excessive intake of K+,*** - **increased dietary K^+^ intake usually does not cause hyperkalemia** - **increased K+ intake related to treatment, such as blood products, administration of intravenous boluses of penicillin G, or replacement of K^+^ can precipitate hyperkalemia** - **a shift of potassium from the ICF to the ECF -- changes in cell membrane permeability -- hypoxia, acidosis or insulin deficiency** - **Hypoxia can impair transport across the cell membrane -- inhibit Na+/K+ pump -- K+ diffuses down its concentration gradient into the ECF** - **In acidosis, H+ excess in ECF is exchanged for K+ from the ICF -- causing increased K+ in ECF** - **Since insulin promote K+ entry into cells, insulin deficiency would produce hyperkalemia** - ***decreased renal excretion*, that produces oliguria (UO \< 30 mL/hr) is accompanied by elevated ECF K+.** - **In acute renal failure K^+^ levels rise more rapidly with more serious consequences than the slower rises associated with chronic renal failure.** - **Hypoaldosteronism causes reabsorption of K+, causing hyperkalemia** - **EKG Changes with Potassium Imbalances.** - **Hypokalemia -- Cardiac effects** - **Prolonged PR interval** - **Peaked P wave** - **Flattened T wave** - **Prominent U wave** - **Hyperkalemia -- Cardiac effects** - **Wide Flat P wave** - **Prolonged PR interval** - **Decreased R Wave amplitude** - **Widened QRS** - **Tall Peaked T Waves** - **Depressed ST (Pseudo Anteroseptal Infarct)** - **Potentially Fatal Arrythmias** **Hyperkalemia \> Stimulates adrenal cortex to increase aldosterone secretion \> renal tubules increase reabsorption of Na+ and excretion of K+ \> Na+ are conserved and K+ are lost. The electrolyte consequences of aldosterone action is for the kidneys to save sodium and water and to excrete potassium. Can produce hypernatremia and hypokalemia.** **Acidosis is an excess of H+ in solution (plasma). One way to compensate for the acidosis is to move H+ into the cells. In order for the cells to be electrically neutral, K+ diffuses out of the cells, resulting in hyperkalemia. -- You see the mechanism in use in DKA, when patient present with hyperkalemia.** **Alkalosis is a deficiency of H+ in solution (plasma). One way to compensate for the alkalosis is to move H+ out of the cells, into the plasma. In order for the cells to be electrically neutral, K+ diffuses into the cells, resulting in hypokalemia** **Calcium (Ca^++^) is required for many essential metabolic processes in the body:** - **major cation needed for the structure of bones and teeth** - **enzymatic cofactor for blood clotting,** - **a requirement for hormone secretion** - **necessary for the function of cell receptors.** - **Contraction of muscles and delayed repolarization of cardiac muscle** - **transmission of nerve impulses.** **Regulation of Ca++** - **Intracellular calcium is located primarily in the mitochondria** - **Parathyroid hormone -- increases ECF Ca++ by reabsorption of Ca++ from bone, GI tract and glomerular filtrate** - **Calcitonin -- decreases ECF Ca++ by depositing Ca++ into bone, inhibiting Ca++ absorption for the GI tract and promoting Ca++ excretion by the kidneys** **HYPOCALCEMIA** **Ca^2+^ \< 8.5 mg/dL** **Causes:** **Hypoparathyroidism** **Vitamin D deficiency** **Multiple blood transfusions** **S&S** **Anxiety, irritability, tetany (spasmotic muscle contractions, numbness and tingling of fingers, convulsions** - **Assess Trousseau's and Chvostek's signs** **Pathophysiology** **Hypocalcemia is when total serum calcium is less than 8.5 mg/dL and ionized levels are less than 5.5 mg/dL. Calcium deficits are due to:** - **inadequate intestinal absorption of calcium,** - **Inadequate intake of dietary sources of Ca++ - dairy products and green, leafy vegetables** - **Excessive intake of dietary phosphorus -- binds with calcium so neither mineral is absorbed.** - **decreases in levels of PTH (surgical removal of parathyroid or thyroid glands), and** - **Decreased vitamin D - inadequate intake, avoidance of sunlight (decreases intestinal absorption of Ca++), malabsorption of fat and fat soluble vitamins.** - **deposition of calcium into bone or soft tissue** - **Blood transfusion -- citrate solution that is the anticoagulant for stored blood products, binds Ca++, decreasing its availability for physiologic processes.** - **Pancreatitis causes the release of lipase into tissues, producing fee fatty acids that bind calcium, so that Ca++ are not available for physiologic processes.** - **Metabolic or respiratory alkalosis enhances protein binding of calcium ions. (McCance & Huether, 2019).** **HYPERCALCEMIA** **Ca^2+^ \> 10.5 mg/dL** **Causes:** **Hyperparathyroidism** **Vitamin D excess** **bone metastases with calcium resorption from breast, prostate, renal, and cervical cancer; sarcoidosis;** **many tumors that produce PTH;** **calcium-containing antacids** **S&S** **Many nonspecific; fatigue, weakness, lethargy, anorexia, nausea, constipation;** **impaired renal function, kidney stones;** **dysrhythmias, bradycardia, cardiac arrest;** **bone pain, osteoporosis, fractures** **Pathophysiology** **Hypercalcemia is total serum calcium concentrations exceeding 10.5 mg/dL The etiology of elevated calcium include:** - **hyperparathyroidism (which can be associated with thyrotoxicosis);** - **bone metastases with calcium resorption from breast, prostate, or cervical cancer, or hematologic malignancy;** - **sarcoidosis;** - **excess vitamin D.** - **Prolonged immobilization - enhanced bone resorption and decreased calcium deposition into bone.** - **Acidosis decreases serum binding of calcium to albumin, increasing ionized calcium levels.(McCance & Huether, 2019).** **Calcium and phosphate concentrations are rigidly controlled, related to the product of calcium (Ca^++^) and phosphate (HPO~4~^-2^) concentrations, which is a constant (*K*) \[Ca^++^ × HPO~4~^-2^ = *K*\]. So, if the concentration of one ion increases, the other ion decreases, and visa versa.** **Three hormones regulate Ca^++^ and PO~4~^-2^ concentrations.** - **parathyroid hormone (PTH),** - **vitamin D** - **calcitonin.** **These substances work together to determine:** - **dietary calcium and phosphate absorbed from the intestine,** - **calcium and phosphate deposited in bone or absorbed from bone,** - **renal reabsorption and excretion of calcium and phosphate by the kidney** **The parathyroid glands secrete PTH when serum calcium levels are low. PTH is required for the renal regulation of calcium and phosphate. It stimulates reabsorption of calcium by the distal tubule of the nephron and inhibits phosphate reabsorption by the proximal tubule of the nephron, increasing serum calcium and increased urinary excretion of phosphate. ** **Vitamin D (cholecalciferol) is also needed for calcium regulation. It is a fat-soluble vitamin ingested in food or synthesized in the skin in the presence of sunlight. The combination of low calcium and increased PTH secretion causes the renal activation of vitamin D. The activated vitamin D (vitamin D~3~---calcitriol) acts to increase absorption of calcium and phosphate in the small intestine, enhance bone calcification, and increase renal tubular reabsorption of calcium and increase excretion of phosphate. When renal failure occurs, vitamin D is not activated; serum calcium levels decrease; and phosphate levels increase.** **When calcium levels increase, an opposite physiologic process occurs, leading to suppression of PTH secretion, decreased renal vitamin D activation, decreased intestinal calcium absorption, and increased renal phosphate reabsorption. Calcitonin (produced by C cells of the thyroid gland) decreases ECF calcium by inhibiting osteoclastic activity in bone, so less calcium is released from bone into the ECF.** **Calcium in the blood stream is either freely ionized in plasma (biologically active) or bound to plasma proteins (biologically inactive). The portion of ionized versus bound calcium is influenced by pH -- acidosis has increased ionized calcium, and alkalosis has increased protein-bound calcium (McCance & Huether, 2019).** **Most of phosphate (HPO~4~^-2^) is found in bone (85%). Small amounts of phosphate are In the plasma as phospholipids, phosphate esters, and ionized phosphate. The normal serum phosphate is 2.5 to 4.5 mg/dL. Intracellular phosphate is in the important energy source - adenosine triphosphate (ATP). Phosphate also acts as a buffer in the regulation of acid-base balance (McCance & Huether, 2019).** **HYPOPHOSPHATEMIA** **HPO~4~^2-^ \< 2.0 mg/dL** **S&S** **Conditions related to reduced capacity for oxygen transport by red blood cells and disturbed energy metabolism; leukocyte and platelet dysfunction; deranged nerve and muscle function; in severe cases, irritability, confusion, numbness, coma, convulsions; possibly respiratory failure (because of muscle weakness), cardiomyopathies, bone resorption (leading to rickets or osteomalacia)** **Pathophysiology** **Hypophosphatemia is a serum phosphate level less than 2.0 mg/dL. Causes include:** - **intestinal malabsorption** - **Vitamin D deficiency** - **use of magnesium- and aluminum-containing antacids (which bind with phosphorus),** - **Long term alcohol abuse** - **increased renal excretion of phosphate.** - **Hyperparathyroidism** - **Respiratory alkalosis can cause severe hypophosphatemia because of cellular use of phosphorus for accelerated glycolysis (ATP) formation (McCance & Huether, 2019).** **HYPERHOSPHATEMIA** **PO~4~^2-^ \> 4.7 mg/dL** **S&S** **Symptoms primarily related to low serum calcium levels (caused by high phosphate levels) similar to symptoms of hypocalcemia; when prolonged, calcification of soft tissues in lungs, kidneys, joints** **Pathophysiology** **Hyperphosphatemia, or an elevated serum phosphate level of more than 4.7 mg/dL. Causes include:** - **addition of phosphorus to the ECF** - **loss of glomerular filtration (kidney disease).** - **cell destruction can cause hyperphosphatemia, because most phosphate is intracellular.** - **Long-term use of phosphate-containing enemas or laxatives also may lead to hyperphosphatemia.** - **Hypoparathyroidism causes elevated phosphate by increasing renal tubular reabsorption of phosphate.** **High levels of serum phosphate will lower serum calcium -- hypocalcemia (McCance & Huether, 2019).** **Chloride is the most common ECF anion, and provides electroneutrality to sodium. Chloride movement is passive and follows the active transport of sodium by the Na+/K+ pump. Chloride increases or decreases are proportional to changes in sodium. Chloride concentration varies inversely with changes in the concentration of bicarbonate (HCO~3~ ^−^).** **Hyperchloremia** **Because chloride follows sodium, hyperchloremia (elevation of serum chloride concentration greater than 105 mEq/L) accompanies hypernatremia, as well as plasma bicarbonate deficits, as in hyperchloremic metabolic acidosis. There are no specific symptoms for chloride excess.** **Hypochloremia** **Hypochloremia, a low level of serum chloride (less than 97 mEq/L), occurs with hyponatremia or an elevated bicarbonate concentration, as in metabolic alkalosis (McCance & Huether, 2019).** **Acid-base balance** **Acid-base balance and hydrogen ion concentration is regulated within a narrow range for the body to function. Hydrogen ions are necessary to maintain membrane integrity and the speed of enzymatic reactions. Most pathologic conditions disturb acid-base balance, and the pH change may be more harmful than the disease process.** **The hydrogen ion concentration, \[H^+^\], is commonly expressed as the pH, the negative logarithm of hydrogen ions in solution. The symbol pH represents the acidity or alkalinity of a solution. As the \[H^+^\] increases, the pH decreases; likewise, as the \[H^+^\] decreases, the pH increases. The greater the \[H^+^\], the more acidic the solution and the lower the pH. The lower the \[H^+^\], the more basic the solution and the higher the pH. In biologic fluids, a pH of less than 7.35 is defined as acidic and a pH greater than 7.45 is defined as basic. The ideal pH is 7.4.** **Our bodies are acid producing through cellular metabolism of proteins, carbohydrates, and fats, and from loss of alkaline fluids in the stools. To maintain a normal pH, acid must be neutralized or excreted. The lungs and kidneys are the regulators of acid-base balance, by working together.** **Body acids exist in two forms: volatile (respiratory acids---eliminated as carbon dioxide \[CO~2~\] gas) and nonvolatile (metabolic acids---eliminated by the kidney or metabolized by the liver). The volatile acid is carbonic acid (H~2~CO~3~), which is formed from the combination of carbon dioxide and water:** **Regulated by the lungs Regulated by the kidneys** **CO~2~ + H~2~O ↔ H~2~CO~3~ ↔ H^+^~\_~ + HCO3^-^** **Carbonic acid dissociates into carbon dioxide and water in the presence of carbonic anhydrase. CO~2~ (a volatile acid) is eliminated by pulmonary ventilation.** **Where as, nonvolatile acids are produced from the incomplete metabolism of proteins, carbohydrates, and fats. The non-volatile acids (H+ in the equation) are eliminated by the renal tubules in conjunction with the regulation of the concentration of bicarbonate (HCO~3~^-^ in the equation).** **So, the carbonic acid/bicarbonate chemical equation can move in either direction to eliminate volatile acids (CO2) or non-volatile acids (H+) and with the help of body buffer systems and renal conservation of HCO~3~^-^, acid-base balance is regulated (McCance & Huether, 2019).** **The respiratory system regulates acid-base balance by controlling the rate of ventilation when there is metabolic acidosis or alkalosis. Central chemoreceptors sense increases or decreases in pH and PaCO~2~ When acidemia exists, the respiratory rate increases (eliminating CO~2~ and reducing carbonic acid concentration). When alkalemia occurs, the respiratory rate decreases (retaining CO~2~ and increasing carbonic acid concentration)" (McCance & Huether, 2019, p. 124).** **This flow chart demonstrates CO2 balance. CO2 accumulates from cellular activity and H+ accumulate (metabolic acidosis). Increases in CO2 and H+ simulate the respiratory centers in the medulla to increase the rate and depth of breathing (hyperventilation). This causes increase CO2 elimination by the lungs, returning CO2 and H+ concentrations to normal.** **The reverse occurs when CO2 cellular production is decreased and there are less H+ (or more OH-) (metabolic alkalosis). Decreases in CO2 and H+ inhibit the respiratory centers in the medulla to decrease rate and depth of breathing (hypoventilation). This causes retention of CO2 by the lungs, returning CO2 and H+ concentrations to normal** **"The distal tubule of the kidney regulates acid-base balance by secreting hydrogen into the urine and regenerating bicarbonate with a maximum urine acidity of a pH of about 4.4 to 4.7. Buffers in the tubular fluid combine with hydrogen ions, allowing more H^+^ to be secreted before the limiting pH value is reached. \[This mechanism allows the urine to accept more H+ than it could without the buffers, increasing the H+ excretion capacity of the kidneys\]. Dibasic phosphate (HPO~4~^-2^) and ammonia (NH~3~) are two important renal buffers because they can attach hydrogen ions and be secreted into the urine. Dibasic phosphate is filtered at the glomerulus. About 75% is reabsorbed, and the remainder is available for buffering H^+^. Secreted H^+^ combines with HPO~4~^-2^ to form monobasic phosphate (H~2~PO~4~^-^). The remaining negative charge on the molecule makes it lipid insoluble, preventing it from diffusing back across the tubular cells and into the blood. Thus the H~2~PO~4~^-^ containing the secreted H^+^ is excreted in the urine" (McCance & Huether, 2019, p. 124).** **To summarize: HPO~4~^-2^ + H^+^ = H~2~PO~4~^-^ So, dibasic phosphate ion and hydrogen ion combines in the ultrafiltrate to produce monobasic phosphate ion. Then the monobasic phosphate (H~2~PO~4~^-^) ion excretes a H^+^ in the urine.** **Beside the compensatory mechanisms in the lungs (eliminate volatile acids) and kidneys (eliminate non-volatile acids) to maintain pH WNL, there are also buffer systems.** **Buffer systems are chemical pairs -- A buffer is a weak acid and its conjugate base or salt.** **Plasma - ECF -- carbonic acid- bicarbonate (20:1 ratio) and hemoglobin.** **Intracellular -- phosphate and protein -- provide the first line of defense to maintain normal pH** **Renal buffering -- in addition to bicarbonate dibasic phosphate and ammonia -- can attach hydrogen ions** **The buffer pairs maintain pH within normal limits by absorbing excessive H^+^ (acid) or hydroxyl ions (OH^−^) (base). The buffers are located in ICF and ECF, and function at different rates. An important factor for effective buffering is the *pK value,* which represents the pH at which each buffer pair is half dissociated -- or separated into its chemical components. Buffer pairs can associate and dissociate.** **The *pK* is the most effective rate for the chemical reaction of each buffer system, where there is an equal concentration of the weak acid and its conjugate base. This occurs when *pK* equals pH. For the bicarbonate--carbonic acid buffer system, the pK is 6.1. This value is not as high as the pK for other buffer systems, but this buffer system is still very effective because carbon dioxide is rapidly removed from the blood by the lungs (McCance & Huether, 2019).** **Now let's look at the various buffer systems.** **Carbonic Acid--Bicarbonate Buffering** **The carbonic acid--bicarbonate buffer pair operates in the lung and the kidney. As the amount of carbon dioxide increases or decreases, the amount of H~2~CO~3~ changes in the same direction. The relationship between the levels of bicarbonate and carbonic acid is expressed as a ratio of 20 : 1 (bicarbonate/carbonic acid) when the pH is normal.** **[\[HCO~e~^-^\] = 24 mEq/L] = = ** **\[H~2~CO~3~\] = 0.03 x 40 mm Hg 1.2 1** **When the values for \[HCO~3~^-^\] and PaCO~2~ (\[H~2~CO~3~\]) increase or decrease proportionately, the 20 : 1 ratio is maintained.** **To decrease acidosis, the lungs can decrease the amount of carbonic acid by exhaling CO~2~ and leaving water. OR The kidneys can reabsorb bicarbonate or regenerate new bicarbonate from CO~2~ and water. The kidneys can also excrete non-volatile acids. The renal mechanism does not act as rapidly as the lungs, but the two systems are very effective together because acid concentration can be rapidly adjusted by the lungs and bicarbonate is easily reabsorbed or regenerated by the kidneys. The pH equation can be symbolically expressed as follows:** **pH = [Renal regulation (slow)] = [Metabolic acid-base function] = [20 ]** **Pulmonary regulation (fast) Respiratory acid base function. 1** **Changes in the numerator or the denominator will change the pH. For example, if the amount of bicarbonate is decreased (the numerator), the pH also decreases, causing acidosis. The pH can be returned to a normal range if the value of the denominator or the amount of carbonic acid also decreases.** **See the graphic for the balance between bicarbonate and carbonic acid on the next slide.** **This graphic shows the relationship between one part of carbonic acid to 20 parts of bicarbonate ions. This balance keeps pH within the normal range. If one of the components is increased or decreased, there must be a proportionate increase or decrease in the other component of acid-base balance.** **The pH equation can be symbolically expressed as follows:** **pH = [Renal regulation (slow)] = [Metabolic acid-base function] = [20 ]** **Pulmonary regulation (fast) Respiratory acid base function. 1** **Changes in the numerator or the denominator will change the pH. For example, if the amount of bicarbonate is decreased (the numerator), the pH also decreases, causing acidosis. The pH can be returned to a normal range if the value of the denominator or the amount of carbonic acid also decreases.** **When a disease process causes an alteration in the bicarbonate/carbonic acid ratio, the kidneys or lungs (i.e., the organ not responsible for causing the alteration) respond to restore the ratio and maintain a normal pH. This is known as compensation. With compensation, a 20 : 1 ratio may be achieved, but the actual values for  and carbonic acid and H~2~CO~3~ concentrations are not normal.** **The respiratory system compensates for changes in pH by increasing or decreasing ventilation, a rapid response occurring within minutes to hours. The renal system compensates by producing more acidic or more alkaline urine, which may take hours to days. Correction occurs when the values for both components of the buffer pair ratio (bicarbonate and carbonic acid) return to normal (McCance & Huether, 2019).** **Protein Buffering** **"Both intracellular and extracellular proteins have negative charges and can serve as buffers for H^+^, but because most proteins are inside cells, they are primarily an intracellular buffer system. Hemoglobin (Hb) is an excellent intracellular blood buffer because of its ability to bind with H^+^ (forming HHb) and carbon dioxide (forming HHbCO~2~). Hemoglobin bound to H^+^ becomes a weak acid. Less oxygen-saturated hemoglobin (venous blood) is a better buffer than hemoglobin saturated with oxygen (arterial blood). (McCance & Huether, 2019, p. 123).** **The regulators work at different rates** **Buffers are the first line of defense because they work almost instantaneously.** **Secondary defenses take longer to work:** **Respiratory mechanisms take several minutes to hours** **Renal mechanisms may take several days** **Acid-Base Imbalances** **Acid-base imbalances** **Metabolic acidosis -- lactic acidosis, renal failure, diabetic ketoacidosis.** **Decreased pH and HCO~3~-** **[Compensation] -- hyperventilation leading to decreased PCO~2~ , pH approaches normal.** ** ** **Metabolic alkalosis -- vomiting, excessive bicarbonate intake, diuretics.Increased pH and HCO~3~-More complicated if it is due to prolonged vomiting (hypochloremic).** **The kidneys try to maintain electrolyte balance by reabsorbing Na+ and excreting H+.** **Therapeutic correction by IV fluids containing NaCl and K+, this allows the kidneys to excrete HCO~3~-. [Compensation] (respiratory) by decreasing rate and depth of** **respirations to retain CO~2,~ but not very effective.** **[Result] - Increased pCO~2~ and pH approaches normal.** ** ** **Respiratory acidosis -- depressed respiration, airway obstruction, decreased respiratory effort due to brain problem or oversedation, chest wall problems, disorders of lungs (pneumonia, PE, COPD, etc.)** **Decreased pH and increased CO~2~** **[Compensation] -- kidneys excrete H+, save HCO~3~-** **[Result] -- Increased HCO~3~- and pH approaches normal.** ** ** **Respiratory alkalosis - hyperventilation due to lung or heart disease, anxiety, hypermetabolic states (fever, etc).** **Increased pH, decreased pCO~2~** **[Compensation] -- if chronic, kidneys decrease H+ excretion and HCO~3~- absorption.** **[Result] -- decreased HCO~3~-and pH approaches normal.** ** ** **This goal is to keep pH within a normal range.** **LAST NAME** **Determine the last name first** **Look at the pH on the ABGs** **Normal pH is 7.35 -- 7.45** ** ** **Acidosis** **pH less than 7.35** **Alkalosis** **pH greater than 7.45** **The more H+ in solution -- The more acid or lower the pH is.** **MIDDLE NAME** **Determine the cause of the acid-base imbalance** **Respiratory** **Acidosis** **pCO2 greater than 45** **Alkalosis** **pCO2 less than 35** **Metabolic** **Acidosis** **HCO3- less than 22** **Alkalosis** **HCO3- greater than 28** **FIRST NAME** **Degree of compensation/correction** **Uncompensated** **No change in the other parameter. If pCO2 is too high, but HCO3- is normal** **Partially compensated** **The other parameter is changing toward the opposite direction, but pH is still abnormal. If pCO2 is too high, HCO3- is elevated, but pH is still too low.** **Corrected** **The other parameter has changed sufficiently in the opposite direction so that the pH has returned to normal. If pCO2 is too high, HCO3- is elevated, but pH is normal.** **Respiratory Acidosis** **Pathophysiology.** **Acid-base imbalance that have a respiratory etiology are caused by increases or decreases of alveolar ventilation related to the metabolic production of carbon dioxide. Respiratory acidosis occurs when there is alveolar hypoventilation. Carbon dioxide is retained, increasing \[H^+^\] (as H~2~CO~3~), thus decreasing the ratio of HCO3- to PCO~2~, and producing acidosis. Carbon dioxide excess in the blood is hypercapnia. The common causes of respiratory acidosis include:** - **depression of the respiratory center (brainstem trauma, oversedation),** - **paralysis of the respiratory muscles,** - **disorders of the chest wall (kyphoscoliosis, pickwickian syndrome, flail chest),** - **disorders of the lung parenchyma (e.g., pneumonitis, pulmonary edema, and chronic obstructive lung disease).** **Respiratory acidosis may be acute or chronic. Acute respiratory acidosis develops rapidly from airway obstruction. There is no effective compensation for acute respiratory acidosis because the renal buffer mechanism takes too long to function -- hours to days. Additionally, the protein buffers do not provide effective compensation elevated PaCO~2~. Acute uncompensated respiratory acidosis is characterized by decreased arterial pH, elevated PaCO~2~, and normal or slightly increased bicarbonate concentration.** **Chronic respiratory acidosis occurs with chronic obstructive pulmonary disease and deformities of the chest wall or neuromuscular disorders. Renal compensation is effective because respiratory acidosis occurs slowly and the renal system has time to compensate over several days. The acidosis produced from CO~2~ retention stimulates the kidney to secrete hydrogen ions and regenerate bicarbonate. Serum bicarbonate and PaCO~2~ levels are elevated, and pH is restored toward normal (McCance & Huether, 2019).** **Respiratory Alkalosis** **Pathophysiology.** **Respiratory alkalosis occurs with alveolar hyperventilation and decreased concentration of plasma carbon dioxide (hypocapnia), thus increasing the ratio of HCO3- to PaCO~2~ (H~2~CO~3~). Stimulation of ventilation is precipitated by:** - **hypoxemia (high altitudes);** - **hypermetabolic states (fever, anemia, and thyrotoxicosis);** - **early salicylate intoxication;** - **anxiety or panic disorder.** - **Incorrect use of mechanical ventilators can cause iatrogenic respiratory alkalosis -- when tidal volume or respiratory rate are too high.** **Acute respiratory alkalosis occurs within minutes of hyperventilation. Cellular buffers provide immediate compensation (i.e., protein and shifts of H^+^ from ICF to ECF). With chronic respiratory alkalosis, renal compensation decreases H^+^ excretion and increases bicarbonate reabsorption, restoring the pH toward normal.** **Secondary respiratory alkalosis may develop from hyperventilation stimulated by metabolic acidosis, causing a mixed acid-base disorder. We will cover mixed acid-base imbalances later. (McCance & Huether, 2019).** **Metabolic Acidosis** **Pathophysiology.** **Metabolic acidosis occurs when the concentration of non--volatile acids increase or bicarbonate (base) is lost from ECF and cannot be regenerated by the kidneys. Metabolic acidosis can occur quickly (poor perfusion or hypoxemia), or slowly, (renal failure, starvation states, or diabetic ketoacidosis). There is a decrease in the 20 : 1 ratio of HCO3- and H~2~CO~3~.** **The buffer systems compensate for the excess acid:** - **H+ will move to the ICF, and K+ will move to the ECF to maintain an ionic balance, taking H+ out of the ECF, and increasing the pH.** - **HCO3- combines with H+ (buffering) to increase the pH.** - **The respiratory system stimulates hyperventilation, lowering the PaCO~2~ and the amount of H~2~CO~3~ circulating in the blood.** - **The kidneys excrete the excess acid as NH4+ and acid (H2PO4-).** **Severe or prolonged acidosis will deplete the buffer systems and H^+^ load will continue to increase, and the pH continues to decrease. The ratio of bicarbonate to carbonic acid decreases to less than 20 : 1. Metabolic acidosis produces hyperkalemia from K+ redistribution to ECF, and reabsorption in the renal collecting tubule. There is also an hypercalcemia because acidosis decreases the amount of calcium bound to albumin (McCance & Huether, 2019).** **Metabolic Alkalosis** **Pathophysiology.** **Metabolic alkalosis occurs when bicarbonate concentration is increased, and the ratio of HCO3- and H~2~CO~3~ is greater than 20 : 1. Conditions that can result in metabolic alkalosis include:** - **hydrogen and chloride depletion (i.e., prolonged vomiting, gastric suctioning),** - **excessive bicarbonate intake,** - **hyperaldosteronism with hypokalemia,** - **diuretic therapy.** **Respiratory compensation for metabolic alkalosis occurs when the elevated pH inhibits the respiratory center in the brain stem. Hypoventilation is produced by decreasing the rate and depth of ventilation, causing retention of carbon dioxide. The ratio of HCO3- concentration to H~2~CO~3~ concentration is reduced toward normal. Respiratory compensation is not very efficient therefore, respiratory alkalosis requires treatment (McCance & Huether, 2019. p.125).** **ABG analysis:** **pH 7.30 pCO2 30 HCO3 18** **Acidosis -- pH \< 7.30** **Metabolic -- HCO3 \< 21** **Compensation - pCO2 \< 35** **Not corrected -- pH is not normal** **pH 7.34 pCO2 55 HCO3 30** **Acidosis -- pH \< 7.35** **Respiratory -- pCO2 \> 45** **Compensation -- HCO3 \> 28** **Nearly corrected -- pH is almost normal** **pH 7.50 pCO2 30 HCO3 20** **Alkalosis -- pH \> 7.45** **Respiratory -- pCO2 \< 35** **Compensation -- HCO3 \< 21** **Not corrected -- pH is not normal.** **The anion gap can be used to distinguish different types of metabolic acidosis.  Normally, the concentrations of cations and anions in the plasma are equivalent. Some anions, such as protein, sulfates, phosphates, and organic acids, however, are not measured in laboratory studies. Therefore, the normal anion gap represents these unmeasured negative ions (sulfate, phosphate, lactate, keto acids, albumin). A convenient measure of the anion gap is the difference between the sum of Na^+^ and K^+^ concentrations and the sum of HCO3- and Cl^−^ concentrations, or about 10 to 12 mEq/L:** **\[Na^+^ + K^+^\] -- \[HCO~3~^-^ + Cl^-^\] = Anion gap \[140 + 4\] -- \[24 + 110\] = 10 mEq/L** **"In metabolic acidosis a normal anion gap is characteristic of conditions related to bicarbonate loss with retention of chloride to maintain an ionic balance. This is called hyperchloremic metabolic acidosis and it occurs with renal failure or prolonged diarrhea with bicarbonate loss. An elevated anion gap is characteristic of acidosis associated with accumulation of anions other than chloride" (McCance & Huether, 2019, p. 125).** **ANION GAP** **(Na+ + K+) -- (Cl- + HCO3-) = anion gap** **"Mixed acid-base disorders are when two or more primary acid-base disorders occur at the same time. They are more common in hospitalized individuals, often those in critical care with comorbid conditions (i.e., combined metabolic and respiratory disorders or combinations of acute and chronic disorders). For these individuals the clinical history and analysis of electrolytes, medications, the anion gap, and plasma and urine osmolality are informative. The primary disorder is assessed and then the degree of compensation is evaluated to determine if it is adequate or greater or lesser than expected. Renal and respiratory compensation rarely returns the pH to normal. Therefore, mixed acid-base disorders can have alterations in PaCO~2~ and bicarbonate and a normal pH" (McCance & Huether, 2019, p. 130).**