Week 1 Lecture 1 Tracers in Met v2 PDF
Document Details
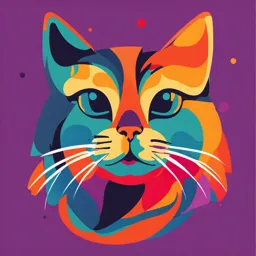
Uploaded by BenevolentRapture
null
Tags
Summary
This document provides a detailed overview of tracer-based methods in metabolic studies. It covers concepts like metabolic flux, radioisotopes, and their medical applications. The document also includes questions to test knowledge retention and further exploration.
Full Transcript
Week 1 Metabolic Tracers Tracer-Based Methods to Study Cellular Metabolism In Vitro Metabolic flux is the passage of a metabolite through a given pathway over time. Macro and microenvironments, hormonal signals and pharmacological perturbations draw unique...
Week 1 Metabolic Tracers Tracer-Based Methods to Study Cellular Metabolism In Vitro Metabolic flux is the passage of a metabolite through a given pathway over time. Macro and microenvironments, hormonal signals and pharmacological perturbations draw unique metabolic flux responses in different cells leading to a diverse range of metabolic phenotypes. These fluxes help elucidate regulation at the level of transcription, translation, post- translation and protein-metabolite interaction. Fluxes are altered under disease conditions such as cardiovascular disease and cancer, so understanding them is of critical value to address treatment efforts. They also provide a look into how microbes are wired informing metabolic engineering as well. https://biochem.web.utah.edu/iwasa/metabolism/chapter2.html https://biochem.web.utah.edu/iwasa/metabolism/chapter2.html https://biochem.web.utah.edu/iwasa/metabolism/chapter2.html https://biochem.web.utah.edu/iwasa/metabolism/chapter2.html 1. In the TCA cycle animation if conversion of pyruvate to acetyl-CoA is blocked what would happen? Do you know which enzyme carries out this reaction? Do you also know how pyruvate enters the mitochondrial matrix? https://biochem.web.utah.edu/iwasa/metabolism/chapter3.html How to investigate steps in a metabolic process Measure biosynthesis and decomposition using extremely small amounts of radioactive isotopes or stable isotopes. A radiotracer is a chemical compound where one or more atoms have been replaced by a radioisotope. Radioactive decay can be used to detect the abundance of metabolite. One can trace the path the radioisotope takes, going from reactant to product. Radioisotopes have been used extensively in studying metabolic pathways. The most commonly used are Hydrogen, Carbon, Phosphorous, Sulfur, and Iodine. Atoms First: An atom is composed of three types of particles: PROTONS, NEUTRONS, and ELECTRONS Each element has a unique number of protons, its atomic number. The number of protons (Z) determines many of the chemical and physical properties associated with an atom. The atomic mass is the sum of protons and neutrons (N), particles with nearly identical weights. (Electrons, negatively charged particles, have insignificant weight – to ecologists!) The atomic number = Z (Protons) The atomic mass = Z + N (Protons + Neutrons) Isotopes and Atomic Mass Radioactivity and Radioisotopes Energy given off spontaneously from the nucleus of an atom is called nuclear radiation. Elements that emit radiation are said to be radioactive. Radiation is a form of energy that we get from natural and human-made sources. In 1896, Henri Becquerel got an exposure on a photographic plate by exposing the plate to a rock that contained uranium. Radioactivity and Radioisotopes Biological Effects of Radiation Nuclear Decay Equation for b-Decay Beta Decay and Positron Emission In beta decay, a high-energy electron is emitted from the nucleus, and a neutron becomes a proton. Radiation Units and Half-Lives Every radioactive isotope emits radiation, at a different rate. Unstable isotopes emit radiation more rapidly. The rate of decay is measured as half-life, the time it takes for one-half (50%) of the atoms in a sample to decay. Decay can me measured by a Geiger counter. Medical Applications for Radioisotopes Nuclear radiation can be high-energy particles or high-energy rays. Some radioisotopes of elements are useful in medical imaging, as they concentrate in particular tissues. The radiation can create an image on a photographic plate or be detected by scanning sections of the body. Medical Applications for Radioisotopes [18F]fluoro-2-deoxy-glucose (FDG) PET-SCAN Warm Cold Garcia et al. Mol Imaging Biol. 2006 8(1):24-9 Medical Applications for Radioisotopes The two main uses of medical radioisotopes Diagnosing diseased states Therapeutically treating diseased tissues When diagnosing a diseased state, a minimum amount of radioisotope is administered. The isotope is for detection only and should have minimal effects on body tissue. Radioisotope used this way is a tracer. Medical Applications for Radioisotopes Gamma emitters are useful for diagnosis because gamma radiation can easily exit the body. If tissue is functioning normally, the radioisotope will be evenly distributed throughout the organ. If there is a nonfunctioning area in the tissue, a “cold” spot is seen. Unusual activity, like rapidly dividing cancer cells, shows up as a “hot” spot. Medical Applications for Radioisotopes Positron Emission Tomography Positron Emission Tomography PET scans are used to identify functional abnormalities in organs and tissues. Fluorine-18 has a half-life of 110 min. The fluorine isotope emits a positron as it decays to form oxygen-18. The positron comes into contact with an electron, and gamma radiation is produced and detected by the scanner. This type of scan is commonly used for the brain. Schematic diagram of human stable isotope infusion study. It is typical that one or more stable isotope tracers are introduced intravenously into the systemic circulation via a catheter placed on one arm. Blood (or other accessible tissues such as muscle and adipose tissues) samples are collected from the other arm (or hand) before and after the tracer infusion. Isotopic enrichment (tracer to tracee ratio, or TTR) of a compound of interest is subsequently determined by means of GC/MS. Mass Spectrometry The primary function of mass spectrometer is to quantify the abundance of a specific mass of ion that is fragmented. Ion fragmentation can be achieved by electron impact ionization that generates fragment of ion. After passing through ionization source, fragmented ions with specific mass(m)/charge(z) ratio can travel through the mass analyzer and hit the electron multiplier (photomultiplier). For biological samples, the charge is generally one, so samples are separated entirely on the basis of mass. The photomultiplier generates a signal, the intensity of which is directly proportional to the abundance of the ions. Mass Spectrometry Ionization: Often electron impact ionization (EI). Samples are first separated in the column and then pass through an electron beam. If the sample molecule is struck by an electron, sufficient energy is imparted to remove an electron from the sample molecule. M + e-- à M+ + 2e-- Gas Chromatography Separation Separation by GC occurs within the column. The sample containing multiple compounds is injected into the column together with the mobile phase. (In GC, the mobile phase is a gas referred to as the carrier gas. He is frequently used.) Both the sample and the mobile phase travel through the column, but the rate of progression within the column differs depending on the compound. Accordingly, differences arise in the times at which the respective compounds arrive at the column outlet. As a result, a separation between each compound occurs. The row of peaks drawn when the electrical signals output from the GC detector are plotted on the vertical axis and the elapsed time after sample injection is plotted on the horizontal axis is called a chromatogram. The components passing through the column are transported by the mobile phase (gas phase) while being partitioned from and adsorbed into the stationary phase (liquid phase and solid phase). Carrier gas typically Helium and Nitrogen. Gas Chromatography Separation A typical chromatogram is shown here. The horizontal axis shows the time until the component reaches the detector. The vertical axis shows the signal intensity. The part at which nothing is detected is called the baseline, and the part where a component is detected is called a peak. The time from when the sample is injected into the system until the peaks appear is called the retention time. As the elution times for each component differ, each component can be separated and detected. Mass Spectrometry Compounds that do not vaporize or are highly reactive cannot be analyzed by GC MS. EI: Electron Ionization A=analyte EI is the most appropriate technique for relatively small (m.w.