Pulmonary Physiology - Pulmonary Mechanics PDF
Document Details
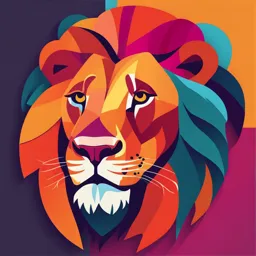
Uploaded by GodlikeUranus
European University Cyprus, School of Medicine
2021
Violetta Raffay
Tags
Summary
These lecture notes detail pulmonary and alveolar ventilation for undergraduate students at European University Cyprus. They cover the mechanics, different types of breathing, and factors that affect the rate and depth of respiration. The notes were adapted from Dr. Raffay's slides, as noted.
Full Transcript
Introduction & mechanics of pulmonary ventilation and Membrane Transport & alveolar ventilation Membrane Potentials (1) Violetta Raffay, MD, PhD Asst. Professor - School of Medicine EUC Feb 2021...
Introduction & mechanics of pulmonary ventilation and Membrane Transport & alveolar ventilation Membrane Potentials (1) Violetta Raffay, MD, PhD Asst. Professor - School of Medicine EUC Feb 2021 Dr Elina Psara The slides were adapted from Dr Raffay’s slides. [email protected] Pulmonary ventilation comprises of 2 major steps: 1. Inspiration 2. Expiration Inspiration is the process that causes air to enter the lungs Expiration is the process that causes air to leave the lungs A respiratory cycle is one sequence of inspiration and expiration Inspiration Muscle groups are used during normal inspiration: Diaphragm External intercostal muscles Additional muscles can be used if a bigger breath is required Contraction of the diaphragm and external intercostal muscles → larger thoracic cavity → more space for the lungs When the diaphragm contracts, it moves inferiorly toward the abdominal cavity Contraction of the external intercostal muscles moves the ribs upward and outward, causing the rib cage to expand Due to the adhesive force of the pleural fluid, the expansion of the thoracic cavity forces the lungs to stretch and expand as well This increase in volume leads to a decrease in intra-alveolar pressure, creating a pressure lower than atmospheric pressure As a result, a pressure gradient is created that drives air into the lungs Expiration The process of normal expiration is passive: energy is not required to push air out of the lungs Instead, as the diaphragm and intercostal muscles relax following inspiration, the elasticity of the lung tissue causes the lung to recoil The thoracic cavity and lungs decrease in volume, causing an increase in intra- alveolar pressure The intra-alveolar pressure rises above atmospheric pressure, creating a pressure gradient that causes air to leave the lungs Inspiration: expansion of the thoracic cavity Expiration: contraction of the thoracic cavity Different modes of breathing (1/2) There are different types, or modes, of breathing that require a slightly different process to allow inspiration and expiration Quiet breathing, aka eupnea: occurs at rest and does not require the cognitive thought of the individual. During quiet breathing, the diaphragm and external intercostals must contract A deep breath, called diaphragmatic breathing: requires the diaphragm to contract. As the diaphragm relaxes, air passively leaves the lungs A shallow breath, called costal breathing: requires contraction of the intercostal muscles. As the intercostal muscles relax, air passively leaves the lungs Different modes of breathing (2/2) Forced breathing, aka hyperpnea: can occur during exercise or actions that require the active manipulation of breathing (e.g. singing) Inspiration and expiration both occur due to muscle contractions. Apart from the diaphragm and intercostal muscles, other accessory muscles must also contract During forced inspiration, muscles of the neck (incl. scalenes) contract and lift the thoracic wall, ↑ lung volume During forced expiration: ▪ Accessory muscles of the abdomen (incl. obliques) contract, forcing abdominal organs upward against the diaphragm → diaphragm is further pushed into the thorax, pushing more air out ▪ Accessory muscles (primarily the internal intercostals) help to compress the rib cage → also reduces the volume of the thoracic cavity Respiratory volumes and capacities (1/3) Respiratory volume is the term used for various volumes of air moved by or associated with the lungs at a given point in the respiratory cycle. There are 4 major types of respiratory volumes: ― Tidal ― Residual ― Inspiratory reserve ― Expiratory reserve Respiratory volume is dependent on a variety of factors Measuring the different types of respiratory volumes can provide important clues about a person’s respiratory health Respiratory volumes and capacities (2/3) Tidal volume (TV): the amount of air that normally enters the lungs during quiet breathing (500 mL) Expiratory reserve volume (ERV): the extra volume of air that can be expired with maximum effort beyond the level reached at the end of a normal, quiet expiration (1100 mL for men and 800 mL in females) Inspiratory reserve volume (IRV): the extra volume of air that can be inspired with maximal effort after reaching the end of a normal, quiet inspiration (3000 mL) Residual volume (RV): the volume of air remaining in the lungs after maximal exhalation The residual volume makes breathing easier by preventing the alveoli from collapsing Respiratory volumes and capacities (3/3) Respiratory capacity is the combination of two or more selected volumes, which further describes the amount of air in the lungs during a given time Total lung capacity (TLC): the sum of all of the lung volumes (TV, ERV, IRV, and RV) → represents the total amount of air a person can hold in the lungs after a forceful inhalation TLC is about 6000 mL air for men, and about 4200 mL for women Vital capacity (VC): the total amount of air exhaled after maximal inhalation → the sum of all of the volumes except residual volume (TV, ERV, and IRV): (4000-5000 mL) Inspiratory capacity (IC): the maximum amount of air that can be inhaled past a normal tidal expiration, is the sum of the tidal volume and inspiratory reserve volume Functional residual capacity (FRC): the amount of air that remains in the lung after a normal tidal expiration; it is the sum of expiratory reserve volume and residual volume Respiratory volumes and capacities These two graphs show (a) respiratory volumes and (b) the combination of volumes that results in respiratory capacity Pulmonary Instrument Measures Function function test Volume of air that is exhaled after maximum Forced vital capacity (FVC) inhalation Forced expiratory volume (FEV) Volume of air exhaled in one breath Forced expiratory flow, Air flow in the middle of exhalation 25-75 percent Peak expiratory flow (PEF) Rate of exhalation Maximum voluntary Volume of air that can be inspired and ventilation (MVV) expired in 1 minute Volume of air that can be slowly exhaled Slow vital capacity (SVC) Spirometry Spirometer after inhaling past the tidal volume Volume of air in the lungs after maximum Total lung capacity (TLC) inhalation Functional residual capacity Volume of air left in the lungs after normal (FRC) expiration Volume of air in the lungs after maximum Residual volume (RV) exhalation Maximum volume of air that the lungs Total lung capacity (TLC) can hold The volume of air that can be exhaled Expiratory reserve volume (ERV) beyond normal exhalation Blood gas Concentration of oxygen and carbon dioxide Gas diffusion Arterial blood gases analyser in the blood Dead space Dead space represents the volume of ventilated air that does not participate in gas exchange. There are 2 types of dead space: Anatomical dead space is represented by the volume of air that fills the conducting zone of respiration made up by the nose, trachea, and bronchi. This volume is considered to be 30% of normal tidal volume → 150 mL Physiologic or total dead space is equal to anatomic plus alveolar dead space Alveolar dead space: the volume of air in the respiratory zone that does not take part in gas exchange. In a healthy adult, alveolar dead space can be considered negligible, meaning that physiologic dead space = anatomical dead space Respiratory rate and control of ventilation Breathing usually occurs without thought, although at times you can consciously control it, such as when you swim under water, sing a song, or blow bubbles The respiratory rate (RR) is the total number of breaths, or respiratory cycles, that occur each minute RR can be an important indicator of disease, as the rate may ↑ or ↓ during an illness or in a disease condition The normal RR of a child ↓ from birth to RR is controlled by the respiratory adolescence. A child under 1 year of age has center located within the medulla a normal RR between 30 and 60 breaths per oblongata in the brain, which minute, but by the time a child is about 10 responds primarily to changes in years old, the normal RR is closer to 18 to 30. CO2, O2, and pH levels in the By adolescence, the normal RR is similar to blood that of adults, 12 to 18 breaths per minute. IN ADULTS: 12-20 breaths per minute Ventilation control centers The control of ventilation is a complex interplay of multiple regions in the brain that signal the muscles used in pulmonary ventilation to contract The result is typically a rhythmic, consistent ventilation rate that provides the body with sufficient amounts of O2, while adequately removing CO2 Summary of ventilation regulation System component Function Medullary respiratory center Sets the basic rhythm of breathing Generatesthe breathing rhythm and integrates data coming into the Ventral respiratory group (VRG) medulla Integrates input from the stretch receptors and the Dorsal respiratory group (DRG) chemoreceptors in the periphery Pontine respiratory group (PRG) Influences and modifies the medulla oblongata’s functions; includes the pneumotaxic and apneustic centers Aortic body Monitors blood PCO2, PO2, and pH Carotid body Monitors blood PCO2, PO2, and pH Hypothalamus Monitors emotional state and body temperature Cortical areas of the brain Control voluntary breathing Proprioceptors Sendimpulses regarding joint and muscle movements Pulmonary irritant reflexes Protect the respiratory zonesof the system from foreign material Inflation reflex Protects the lungs from over-inflating Respiratory centers of the brain (1/3) Neurons that innervate the muscles of the respiratory system are responsible for controlling and regulating pulmonary ventilation The major brain centers involved in pulmonary ventilation are the medulla oblongata and the pontine respiratory group Respiratory centers of the brain (2/3) The medulla oblongata contains the dorsal respiratory group (DRG) and the ventral respiratory group (VRG) The DRG is involved in maintaining a constant breathing rhythm by stimulating the diaphragm and intercostal muscles to contract, resulting in inspiration When activity in the DRG ceases, it no longer stimulates the diaphragm and intercostals to contract, allowing them to relax, resulting in expiration The VRG is involved in forced breathing only, as the neurons in the VRG stimulate the accessory muscles involved in forced breathing to contract, resulting in forced inspiration The VRG also stimulates the accessory muscles involved in forced expiration to contract Respiratory centers of the brain (3/3) The second respiratory center of the brain is located within the pons, called the pontine respiratory group, and consists of the apneustic and pneumotaxic centers The apneustic center is a double cluster of neuronal cell bodies that stimulate neurons in the DRG, controlling the depth of inspiration, particularly for deep breathing The pneumotaxic center is a network of neurons that inhibits the activity of neurons in the DRG, allowing relaxation after inspiration, and thus controlling the overall rate Responsible for limiting inspiration, providing an inspiratory off- switch Factors that affect the rate and depth of respiration (1/6) The respiratory rate and the depth of inspiration are regulated by the medulla oblongata and pons The medulla oblongata and pons respond to systemic stimuli It is a dose-response, positive-feedback relationship; the greater the stimulus, the greater the response Increasing stimuli results in forced breathing Multiple systemic factors are involved in stimulating the brain to produce pulmonary ventilation Factors that affect the rate and depth of respiration (2/6) The major factor that stimulates the medulla oblongata and pons to produce respiration is surprisingly not [O2], but rather the [CO2] in the blood As you recall, CO2 is a waste product of cellular respiration and can be toxic ― Concentrations of chemicals are sensed by chemoreceptors A central chemoreceptor is one of the specialised receptors that are in the brain and brainstem A peripheral chemoreceptor is one of the specialised receptors located in the carotid arteries and aortic arch Concentration changes in certain substances, such as CO2 or H+, stimulate these receptors, which in turn signal the respiration centers of the brain Factors that affect the rate and depth of respiration (3/6) As the [CO2] in the blood ↑, it diffuses across the BBB, where it collects in the extracellular fluid; ↑ CO2 levels lead to ↑ levels of H+, ↓ pH The ↑ in H+ in the brain triggers the central chemoreceptors to stimulate the respiratory centers to initiate contraction of the diaphragm and intercostal muscles As a result, the rate and depth of respiration ↑, allowing more CO2 to be expelled, which brings more air into and out of the lungs promoting a ↓ in the blood levels of CO2, and therefore H+, in the blood In contrast, low levels of CO2 in the blood cause low levels of H+ in the brain, leading to a ↓ in the rate and depth of pulmonary ventilation, producing shallow, slow breathing Factors that affect the rate and depth of respiration (4/6) Another factor involved in influencing the respiratory activity of the brain is systemic arterial concentrations of H+ Increasing CO2 levels can lead to increased H+ levels, as mentioned before, as well as other metabolic activities, such as lactic acid accumulation after strenuous exercise Peripheral chemoreceptors of the aortic arch and carotid arteries sense arterial levels of H+ When peripheral chemoreceptors sense decreasing, or more acidic, pH levels, they stimulate an increase in ventilation to remove CO2 from the blood at a quicker rate Removal of CO2 from the blood helps to reduce H+, thus increasing systemic pH Factors that affect the rate and depth of respiration (5/6) Blood levels of O2 are also important in influencing respiratory rate The peripheral chemoreceptors are responsible for sensing large changes in blood O2 levels If blood O2 levels become quite low—about 60 mmHg or less—then peripheral chemoreceptors stimulate an ↑ in respiratory activity The chemoreceptors are only able to sense dissolved oxygen molecules, not the O2 that is bound to hemoglobin ― The majority of O2 is bound by haemoglobin; when dissolved levels of O2 drop, haemoglobin releases O2 Therefore, a large drop in O2 levels is required to stimulate the chemoreceptors of the aortic arch and carotid arteries Factors that affect the rate and depth of respiration (6/6) The hypothalamus and other brain regions associated with the limbic system also play roles in influencing the regulation of breathing by interacting with the respiratory centers The brain regions associated with the limbic system are involved in regulating respiration in response to emotions, pain, and temperature ― For example, an ↑ in body temperature causes an ↑ in respiratory rate Feeling excited or the fight-or-flight response will also result in an ↑ in respiratory rate Pulmonary physiology - Pulmonary mechanics Membrane Transport & Membrane Potentials (1) Violetta Raffay, MD, PhD Asst. Professor - School of Medicine EUC Feb 2021 The slides were adapted from Dr Raffay’s slides. Dr Elina Psara [email protected] Basic pulmonary mechanics Air needs to go in and air needs to come out ― Inspiration and expiration Inspiration (active) Most important muscle of inspiration is the diaphragm ― Innervated by phrenic nerves (ribs, spine) Lungs ↑ in size by expanding downwards (vertical dimension; resting breathing 1-2 cm and max can move up to 10 cm) and ↑ outwards, transverse and A-P diameter (external intercostals; bucket handle move) Accessory muscles of respiration (scalenes, sternocleidomastoid) Accessory muscles of respiration Basic pulmonary mechanics Basic pulmonary mechanics Expiration (passive) Results from the elastic recoil of the lung The most important muscles are the abdominal muscles They push the abdominal content inward and thus the air of the lung outward (abdominal recti, transversus abdomini, obliques) Internal intercostal muscles Basic pulmonary pressure-volume loops We create a negative pressure around the lung and the lung inflates The difference between the transpulmonary pressure of inhalation (increasing volume) and the pressure of exhalation (decreasing volume) is called hysteresis Compliance of the lung (lung tissue and surface tension) Decreased compliance in fibrosis/oedema Increased compliance (not same recoil pressure) – emphysema Emphysema is a lung condition that causes shortness of breath. In people with emphysema, the air sacs in the lungs (alveoli) are damaged. Over time, the inner walls of the air sacs weaken and rupture — creating larger air spaces instead of many small ones Basic pulmonary pressure-volume loops Factors involved in lung compliance: Elasticity from the elastin in connective tissue and surface tension, which is decreased by surfactant production Surfactant reduces surface tension and this reduces the collapsibility of the lung Basic pulmonary pressure-volume relation Basic pulmonary surface tension Surface tension (cohesive bonding of molecules of liquids) Prevents collapse Surfactant dipamitoyl phosphatidyl choline (DPPC) produced by type II alveolar cells Basic pulmonary air flow Laminar flow refers to the state of flow in which air moves through a tube in parallel layers, with no disruption between the layers, and the central layers flowing with the greatest velocity Turbulent flow refers to when air is not flowing in parallel layers, and direction, velocity and pressure within the flow of air become chaotic Basic pulmonary air flow Laminar-turbulent transitional flow is a mixture of laminar and turbulent flow, with turbulence in the center of the pipe, and laminar flow near the edges Small airways (laminar) → trachea (turbulent) Basic pulmonary air flow Poiseuille's Law, also known as the Hagen-Poiseuille equation, gives us the relationship between airway resistance and the diameter of the airway Reynolds number is the ratio of gas density to gas viscosity Reynolds number < 2300 = laminar flow Basic pulmonary air flow As lung volume ↑, airway diameter ↑ → airway resistance ↓ exponentially As lung volume ↓, airway diameter ↓ As lung volume ↑, conductance also ↑ Conductance is an expression of the amount of air reaching the alveoli per unit of time per unit of pressure Basic pulmonary air flow Basic pulmonary air flow Relations between expiratory and inspiratory effort (as represented by the intrapleural pressure) and flow rates Mechanism of dynamic compression in forced expiration Dynamic airway compression occurs when the pressure surrounding the airway exceeds the pressure within the airway lumen Question 1 What is the best definition of tidal volume? A. The maximum amount of air that can be forcibly exhaled after a maximum inhalation B. The amount of air that remains in the lungs after a forced exhalation C. The amount of air that can be forcibly inhaled after a normal exhalation D. The amount of air that moves in and out of the lungs during normal breathing. Question 2 Which of the following muscles are primarily used in inspiration? A. Diaphragm and external intercostals B. Internal intercostals and diaphragm C. External intercostals and abdominal muscles D. Internal intercostals and abdominal muscles Thank you!