PDH in Biochemistry PDF
Document Details
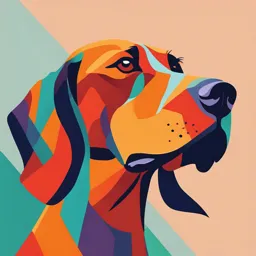
Uploaded by VeritableJadeite
University of Northern Philippines
Tags
Summary
This document provides an overview of the Pyruvate Dehydrogenase (PDH) complex in biochemistry, a crucial enzyme complex in cellular metabolism. It details the components, regulation, and significance of PDH in various metabolic processes.
Full Transcript
Pyruvate Dehydrogenase (PDH) in Biochemistry Pyruvate dehydrogenase (PDH) is a crucial enzyme complex in cellular metabolism that catalyzes the conversion of pyruvate (a product of glycolysis) into acetyl-CoA. This reaction is a pivotal step in linking glycolysis to the citric acid cycle, enabling...
Pyruvate Dehydrogenase (PDH) in Biochemistry Pyruvate dehydrogenase (PDH) is a crucial enzyme complex in cellular metabolism that catalyzes the conversion of pyruvate (a product of glycolysis) into acetyl-CoA. This reaction is a pivotal step in linking glycolysis to the citric acid cycle, enabling the efficient production of ATP through aerobic respiration. Components and Function The PDH complex is composed of three distinct enzymes: Pyruvate dehydrogenase (E1): This enzyme catalyzes the decarboxylation of pyruvate and the formation of a hydroxyethyl-TPP intermediate. Dihydrolipoyl transacetylase (E2): This enzyme transfers the acetyl group from the hydroxyethyl-TPP intermediate to lipoamide, forming acetyl-CoA. Dihydrolipoyl dehydrogenase (E3): This enzyme regenerates the oxidized form of lipoamide, producing NADH in the process. Regulation The activity of the PDH complex is tightly regulated to meet the cell's energy needs. Key factors influencing its activity include: Phosphorylation: PDH kinase phosphorylates PDH, inhibiting its activity. PDH phosphatase removes these phosphate groups, activating the enzyme. Allosteric regulation: Acetyl-CoA, NADH, and ATP are allosteric inhibitors of PDH, while pyruvate and ADP are allosteric activators. Significance in Metabolism The PDH complex plays a critical role in: Energy production: By converting pyruvate into acetyl-CoA, it fuels the citric acid cycle, leading to the production of ATP through oxidative phosphorylation. Metabolic regulation: The regulation of PDH activity helps to balance energy production with the needs of the cell. Anabolic processes: Acetyl-CoA is also a precursor for fatty acid synthesis and other anabolic pathways. In summary, the PDH complex is a vital enzyme in cellular metabolism, linking glycolysis to the citric acid cycle and playing a crucial role in energy production and metabolic regulation. Would you like to learn more about a specific aspect of PDH or its role in a particular metabolic pathway? – Recent Pyruvate Dehydrogenase Complex in Metabolism Glucose Storage Disease Overview Arterial Blood Gas Analysis Overview Glycolysis Explained Crafting Exam Questions Show more Gem manager Help Activity Settings Try Gemini Advanced Dipaculao, Aurora, Philippines Based on your places (Home) Update location Gemini was just updated. See update Conversation with Gemini PDH in Biochemistry Show drafts Pyruvate Dehydrogenase (PDH) in Biochemistry Pyruvate dehydrogenase (PDH) is a crucial enzyme complex in cellular metabolism that catalyzes the conversion of pyruvate (a product of glycolysis) into acetyl-CoA. This reaction is a pivotal step in linking glycolysis to the citric acid cycle, enabling the efficient production of ATP through aerobic respiration. Components and Function The PDH complex is composed of three distinct enzymes: Pyruvate dehydrogenase (E1): This enzyme catalyzes the decarboxylation of pyruvate and the formation of a hydroxyethyl-TPP intermediate. Dihydrolipoyl transacetylase (E2): This enzyme transfers the acetyl group from the hydroxyethyl-TPP intermediate to lipoamide, forming acetyl-CoA. Dihydrolipoyl dehydrogenase (E3): This enzyme regenerates the oxidized form of lipoamide, producing NADH in the process. Regulation The activity of the PDH complex is tightly regulated to meet the cell's energy needs. Key factors influencing its activity include: Phosphorylation: PDH kinase phosphorylates PDH, inhibiting its activity. PDH phosphatase removes these phosphate groups, activating the enzyme. Allosteric regulation: Acetyl-CoA, NADH, and ATP are allosteric inhibitors of PDH, while pyruvate and ADP are allosteric activators. Significance in Metabolism The PDH complex plays a critical role in: Energy production: By converting pyruvate into acetyl-CoA, it fuels the citric acid cycle, leading to the production of ATP through oxidative phosphorylation. Metabolic regulation: The regulation of PDH activity helps to balance energy production with the needs of the cell. Anabolic processes: Acetyl-CoA is also a precursor for fatty acid synthesis and other anabolic pathways. In summary, the PDH complex is a vital enzyme in cellular metabolism, linking glycolysis to the citric acid cycle and playing a crucial role in energy production and metabolic regulation. Would you like to learn more about a specific aspect of PDH or its role in a particular metabolic pathway? TCA in Biochemistry Show drafts The Citric Acid Cycle (TCA Cycle) in Biochemistry The tricarboxylic acid (TCA) cycle, also known as the Krebs cycle or citric acid cycle, is a central metabolic hub that plays a pivotal role in energy production through aerobic respiration. It occurs within the mitochondrial matrix of eukaryotic cells and the cytoplasm of prokaryotic cells. Key Steps and Reactions: The TCA cycle is a circular pathway that consists of a series of redox reactions. Here's a simplified overview of the steps involved: 1. Acetyl-CoA entry: Acetyl-CoA, a product of glycolysis, pyruvate oxidation, and fatty acid breakdown, enters the cycle and combines with oxaloacetate to form citrate. 2. Citrate to isocitrate: Citrate undergoes isomerization to form isocitrate. 3. Isocitrate to α-ketoglutarate: Isocitrate is oxidized and decarboxylated to produce α-ketoglutarate, generating a molecule of CO2 and a molecule of NADH. 4. α-Ketoglutarate to succinyl-CoA: α-Ketoglutarate undergoes oxidative decarboxylation to form succinyl-CoA, producing another molecule of CO2 and NADH. 5. Succinyl-CoA to succinate: Succinyl-CoA is converted to succinate, coupled with the substrate-level phosphorylation of GDP to GTP. 6. Succinate to fumarate: Succinate is oxidized to fumarate, producing FADH2. 7. Fumarate to malate: Fumarate is hydrated to form malate. 8. Malate to oxaloacetate: Malate is oxidized to regenerate oxaloacetate, producing another molecule of NADH and completing the cycle. Energy Production: For each turn of the TCA cycle, the following energy molecules are produced: 1 ATP (or GTP) through substrate-level phosphorylation 3 NADH 1 FADH2 These energy molecules are then used in the electron transport chain to generate ATP through oxidative phosphorylation, yielding a significant amount of cellular energy. Importance of the TCA Cycle: Energy production: The TCA cycle is essential for producing ATP, the primary energy currency of cells. Anabolism: Intermediates of the TCA cycle serve as precursors for the biosynthesis of amino acids, fatty acids, and other molecules. Catabolism: The cycle plays a role in the breakdown of amino acids and fatty acids. Regulation: The activity of the TCA cycle is regulated by various factors, including the availability of substrates, the energy needs of the cell, and allosteric regulation by molecules such as ATP and NADH. - Gemini was just updated. See update Conversation with Gemini PDH in Biochemistry Pyruvate Dehydrogenase (PDH) in Biochemistry Pyruvate dehydrogenase (PDH) is a crucial enzyme complex in cellular metabolism that catalyzes the conversion of pyruvate (a product of glycolysis) into acetyl-CoA. This reaction is a pivotal step in linking glycolysis to the citric acid cycle, enabling the efficient production of ATP through aerobic respiration. Components and Function The PDH complex is composed of three distinct enzymes: Pyruvate dehydrogenase (E1): This enzyme catalyzes the decarboxylation of pyruvate and the formation of a hydroxyethyl-TPP intermediate. Dihydrolipoyl transacetylase (E2): This enzyme transfers the acetyl group from the hydroxyethyl-TPP intermediate to lipoamide, forming acetyl-CoA. Dihydrolipoyl dehydrogenase (E3): This enzyme regenerates the oxidized form of lipoamide, producing NADH in the process. Regulation The activity of the PDH complex is tightly regulated to meet the cell's energy needs. Key factors influencing its activity include: Phosphorylation: PDH kinase phosphorylates PDH, inhibiting its activity. PDH phosphatase removes these phosphate groups, activating the enzyme. Allosteric regulation: Acetyl-CoA, NADH, and ATP are allosteric inhibitors of PDH, while pyruvate and ADP are allosteric activators. Significance in Metabolism The PDH complex plays a critical role in: Energy production: By converting pyruvate into acetyl-CoA, it fuels the citric acid cycle, leading to the production of ATP through oxidative phosphorylation. Metabolic regulation: The regulation of PDH activity helps to balance energy production with the needs of the cell. Anabolic processes: Acetyl-CoA is also a precursor for fatty acid synthesis and other anabolic pathways. In summary, the PDH complex is a vital enzyme in cellular metabolism, linking glycolysis to the citric acid cycle and playing a crucial role in energy production and metabolic regulation. Would you like to learn more about a specific aspect of PDH or its role in a particular metabolic pathway? TCA in Biochemistry The Citric Acid Cycle (TCA Cycle) in Biochemistry The tricarboxylic acid (TCA) cycle, also known as the Krebs cycle or citric acid cycle, is a central metabolic hub that plays a pivotal role in energy production through aerobic respiration. It occurs within the mitochondrial matrix of eukaryotic cells and the cytoplasm of prokaryotic cells. Key Steps and Reactions: The TCA cycle is a circular pathway that consists of a series of redox reactions. Here's a simplified overview of the steps involved: 1. Acetyl-CoA entry: Acetyl-CoA, a product of glycolysis, pyruvate oxidation, and fatty acid breakdown, enters the cycle and combines with oxaloacetate to form citrate. 2. Citrate to isocitrate: Citrate undergoes isomerization to form isocitrate. 3. Isocitrate to α-ketoglutarate: Isocitrate is oxidized and decarboxylated to produce α-ketoglutarate, generating a molecule of CO2 and a molecule of NADH. 4. α-Ketoglutarate to succinyl-CoA: α-Ketoglutarate undergoes oxidative decarboxylation to form succinyl-CoA, producing another molecule of CO2 and NADH. 5. Succinyl-CoA to succinate: Succinyl-CoA is converted to succinate, coupled with the substrate-level phosphorylation of GDP to GTP. 6. Succinate to fumarate: Succinate is oxidized to fumarate, producing FADH2. 7. Fumarate to malate: Fumarate is hydrated to form malate. 8. Malate to oxaloacetate: Malate is oxidized to regenerate oxaloacetate, producing another molecule of NADH and completing the cycle. Energy Production: For each turn of the TCA cycle, the following energy molecules are produced: 1 ATP (or GTP) through substrate-level phosphorylation 3 NADH 1 FADH2 These energy molecules are then used in the electron transport chain to generate ATP through oxidative phosphorylation, yielding a significant amount of cellular energy. Importance of the TCA Cycle: Energy production: The TCA cycle is essential for producing ATP, the primary energy currency of cells. Anabolism: Intermediates of the TCA cycle serve as precursors for the biosynthesis of amino acids, fatty acids, and other molecules. Catabolism: The cycle plays a role in the breakdown of amino acids and fatty acids. Regulation: The activity of the TCA cycle is regulated by various factors, including the availability of substrates, the energy needs of the cell, and allosteric regulation by molecules such as ATP and NADH. Would you like to learn more about a specific aspect of the TCA cycle, such as its regulation, anabolic functions, or its role in diseases? ETC in biochemistry www.numerade.com/ask/question/explain-chemiosmotic-coupling-and-describe-the-orga nelle-where-it-occurs-the-mitochondria-explain-the-following-processes-that-occur-in-cel ls-by-which-energy-is-generated-glycolysis-the-kreb-09931/ Electron Transport Chain (ETC) in Biochemistry The electron transport chain (ETC) is a series of protein complexes located in the inner mitochondrial membrane of eukaryotic cells and the cell membrane of prokaryotic cells. It plays a crucial role in aerobic respiration, generating the majority of ATP, the cell's primary energy currency. Components and Function: The ETC consists of four major protein complexes: 1. Complex I (NADH dehydrogenase): This complex accepts electrons from NADH and transfers them to ubiquinone (CoQ). 2. Complex II (succinate dehydrogenase): This complex accepts electrons from FADH2 (produced in the TCA cycle) and transfers them to ubiquinone. 3. Complex III (cytochrome bc1 complex): This complex transfers electrons from ubiquinone to cytochrome c. 4. Complex IV (cytochrome oxidase): This complex transfers electrons from cytochrome c to molecular oxygen, producing water. As electrons move through these complexes, they release energy, which is used to pump protons (H+) from the mitochondrial matrix into the intermembrane space, creating a proton gradient. This proton gradient drives the synthesis of ATP by the ATP synthase enzyme. ATP Synthesis: ATP synthase is a protein complex embedded in the inner mitochondrial membrane. It utilizes the proton gradient created by the ETC to drive the synthesis of ATP from ADP and inorganic phosphate (Pi). The flow of protons through ATP synthase powers the rotation of a mechanical component, which in turn catalyzes the synthesis of ATP. Energy Yield: The ETC is incredibly efficient in energy production. For each pair of electrons transferred from NADH to oxygen, approximately 3 ATP molecules are produced. For each pair of electrons transferred from FADH2 to oxygen, approximately 2 ATP molecules are produced. Regulation: The activity of the ETC is regulated by factors such as the availability of substrates (NADH and FADH2), the oxygen concentration, and the energy needs of the cell. In summary, the ETC is a vital component of aerobic respiration, responsible for generating the majority of ATP. It utilizes the energy released from electron transfer to create a proton gradient, which drives the synthesis of ATP by ATP synthase.