Cell Division and Apoptosis - CBG 223 - University of Lagos
Document Details
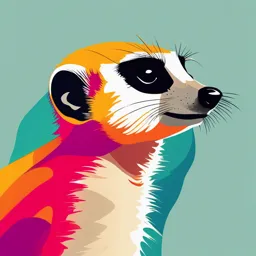
Uploaded by HardWorkingEpic
University of Lagos
2024
Bodunrin O. Ottu
Tags
Summary
This document is a lecture set on cell division and apoptosis. It covers the concept of cell division in prokaryotes and eukaryotes, the stages of the cell cycle, and the role of cyclins and CDKs in regulation. It also outlines cellular responses to stimuli, such as apoptosis.
Full Transcript
CELL DIVISION AND APOPTOSIS CBG 223 Bodunrin O. Ottu Department of Cell Biology and Genetics, Room 203, Faculty of Science, University of Lagos. E: [email protected] April 2024 LEARNING OBJECTIVES...
CELL DIVISION AND APOPTOSIS CBG 223 Bodunrin O. Ottu Department of Cell Biology and Genetics, Room 203, Faculty of Science, University of Lagos. E: [email protected] April 2024 LEARNING OBJECTIVES At the end of the lecture, student should be able to understand the concept of cell division in prokaryotes and eukaryotes the various stages of the cell cycle the role of cyclins and CDKs in the regulation of the cell cycle cellular motility and migration INTRODUCTION CBG 223 – Cell Physiology – Study of activities such as nutrition, cell growth, division, reproduction, differentiation, communication. Cell physiology is the study of how cells function and operate in living organisms. CELL PHYSIOLOGY…....CBG 211 Cell Structure: Understanding the different organelles within a cell, such as the nucleus, mitochondria, ER and Golgi apparatus, and how they contribute to cellular function. Cellular Energy Production: Investigating how cells generate energy through processes like cellular respiration (involving mitochondria) or photosynthesis (in plant cells). Cellular Metabolism: Exploring metabolic pathways within cells, such as glycolysis, the citric acid cycle, and oxidative phosphorylation, which are involved in energy production and the synthesis of biomolecules. CELL PHYSIOLOGY Cell Cycle and Division: Examining the stages of the cell cycle (interphase, mitosis, and cytokinesis) and how cells regulate their growth, replication, and division. Cellular Responses to Stimuli: Understanding how cells respond to various stimuli, such as stress, pathogens, and environmental changes, through processes like immune responses, cell differentiation, and apoptosis (programmed cell death). CELL PHYSIOLOGY Cellular Communication: Exploring how cells communicate with each other through signaling pathways, including chemical signals like hormones, neurotransmitters, and cytokines. Transport Processes: Studying how cells transport molecules such as nutrients, ions, and waste products across their membranes through processes like diffusion, osmosis, active transport, and endocytosis/exocytosis. Homeostasis: Investigating how cells maintain internal balance and stability despite changing external conditions, including pH regulation, temperature control, and osmotic balance. ❑ Recall that there are three domains of life -Archaea, Bacteria and Eukaryota. ❑ Archaea and Bacteria (unicellular prokaryotes) lack a nucleus and other organelles. ❑ Eukaryotes, unicellular or multicellular, have a nucleus and other membrane-bound organelles. Reece et al., 2010 CBG 223 – Cell Physiology – Study of activities such as nutrition, cell growth, division, reproduction, differentiation. The only way to make new cells is to duplicate a cell that already exists. A cell reproduces by performing an orderly sequence of events in which it duplicates its content and then divides into two. This cycle of duplication and division is known as the cell cycle. A dividing cell duplicates its DNA, allocates the two copies to opposite ends of the cell, and only then splits into daughter cells. Replication, segregation and cytokinesis. Except meiosis CELL GROWTH: EMBRYOID BODIES Day 2 EBs…. 4X Day 9 EBs…. 4X CELL DIFFERENTIATION: EBS AND CARDIOMYOCYTES Day 5 EBs….mp4 Day 7 miPSC~CM…. 4X Unlike prokaryotes, which undergo binary fission, cell division in eukaryotes is a bit complicated.Why? Because of their increased number of chromosomes, organelles and complexity. Eukaryotic cell division THE (EUKARYOTIC) CELL CYCLE STAGES OF THE CELL CYCLE In eukaryotic cells, the cell cycle consists of four phases. Interphase (G1, S, G2) and M-phase G0 phase - “resting” phase that we will discuss later STAGES OF THE CELL CYCLE – INTERPHASE Interphase (G1, S, G2) Gap phase 1 (G1) – when the cell grows and prepares to synthesize DNA Synthesis phase (S) – when DNA synthesis takes place and replicates the whole set of chromosomes A second gap period phase (G2) – in which the cell prepares for division. Interphase lasts for at least 91% of the total time required for the cell cycle. STAGES OF THE CELL CYCLE – THE MITOTIC PHASE The mitotic phase (M) – the actual division of the original cell into two daughter cells takes place. M phase involves two distinct division-related processes – mitosis and cytokinesis. In mitosis, the nuclear DNA of the cell condenses into visible chromosomes and is pulled apart by the mitotic spindle, a specialized structure made out of microtubules. STAGES OF THE CELL CYCLE – THE MITOTIC PHASE Mitosis takes place in four major stages: prophase (sometimes divided into early prophase and prometaphase), metaphase, anaphase and telophase. prophase, when chromosome condensation takes place. prometaphase-metaphase, in which two members of each pair of sister chromatids attach to microtubules and align themselves at the equator. anaphase, when sister chromatids are separated and move along the microtubules. CBG 222 telophase, with the reformation of nuclei and the decondensation of the chromosomes. STAGES OF THE CELL CYCLE – THE MITOTIC PHASE Mitosis is usually followed by cytokinesis. CBG Animal cells carry out cytokinesis by cleavage, 222 while plant cells form a cell plate. At the exit from mitosis, and depending upon the availability of nutrients and storage of energy-rich compounds, at the checkpoint, the new daughter cells may (1) re-enter G1 phase and keep cycling, (2) remain temporarily quiescent in a stage named G0 phase, or (3) be terminally differentiated and unable to re-enter the cell cycle In G0, the cell is not actively preparing to divide, it’s just performing its cellular function. E.g. it might conduct signals as a neuron. G0 could be permanent for some cells or temporary if the cell gets the right signals. A cell in G0 phase may be said to be quiescent or senescent. SUMMARY OF POINTS REGULATION OF THE CELL CYCLE Cell cycle regulation: How to ensure the orderly progression of events so that nuclear cycle is coordinated with cell growth and physical separation. Replication must occur once per cell cycle and precede chromosome segregation; Segregation must be complete before cytokinesis. How does the cell know when to enter/exit the cell cycle phases? REGULATION OF THE CELL CYCLE- Historical background Xenopus laevis oocytes was used by Dettlaf et al. (1964) to study factors that induced mitosis and meiosis. Oocytes that develop in the frog arrest in the G2 stage of the cell cycle for up to 8 months and are induced to enter meiosis I by progesterone. These oocytes proceed through meiosis I and arrest once more in metaphase of meiosis II. Meiosis is completed only when the nucleus is released from the second arrest following egg fertilization. Yoshio Masui and Clement Markert (1971) used these observations to study meiotic maturation in another frog, Rana pipiens. REGULATION OF THE They induced oocytes CELL maturation CYCLE by progesterone in vitro, removed cytoplasm from mature progesterone-treated oocytes and added to immature G2 arrested oocytes. Yoshio Masui The injected cytoplasm was able to induce maturation of immature oocytes 12 hours after being treated with progesterone and was shown to contain maturation promoting factor (MPF). It took another 17 years before MPF could be purified by Lohka et al. (1988). It was postulated that MPF was composed of two components, one of which contained kinase activity 1971 Masui and Markert First identification of MPF J. Exp. Zool. 177: 129-145 1984 Gerhart et al. Identification of pre-MPF J. Cell Biol. 98: 1247-1255 Lohka et al. 1988 Final purification of MPF Proc. Natl Acad. Sci. 85:3009-3013 REGULATION OF THE CELL CYCLE Hartwell used budding yeast to identify mutants that blocked specific stages of cell cycle progression. Nurse, working in fission yeast went on to isolate mutants that could also speed up the cell cycle and identified the original CDK kinase, Cdc2. Hunt identified extracts proteins that varied through the cell cycle in sea urchins hence "cyclins". 2001 Nobel Prize in Physiology or Medicine was awarded to Leland H. Hartwell, Paul M. Nurse and Timothy R. Hunt for their ground-breaking work on cell cycle regulation. https://www.nobelprize.org/prizes/medicine/2001/press-release/ cdc2 Sp = cdc28 Sc cdc13 = cyclinB THE UNIFYING THEORY OF CELL CYCLE CONTROL Start gene by Hartwell It was postulated that MPF was composed of two components, one of which contained kinase activity MPF = cdc28 = cdc2 = CDK catalytic subunit CyclinB cdc13 cyclin regulatory subunit … … Cyclin-CDK complexes are negatively regulated by two different families of CDK inhibitors: CDK-inhibitor proteins (Cip/Kip) Inhibitors of kinases (Ink) CDK = cyclin Further control of the cell cycle progression is exerted through; ubiquitin mediated proteolysis of cyclins (e.g., D, E, A, and B) CDK inhibitors (e.g., p27) transcription factors (e.g., E2F) anaphase inhibitors, glue proteins, etc. REGULATION OF THE CELL CYCLE- CHECKPOINTS Hartwell used budding yeast to identify mutants that blocked specific stages of cell cycle progression. Weinert and Hartwell (1989) used the term checkpoint in relation to the gene products that negatively regulate the cell cycle in response to DNA damage in yeasts. DNA damage Rad9 Three checkpoints safeguard the normal progression of the cell cycle; at G1-S, at G2-M, and at the exit of mitosis. PAUL NURSE’S SCREEN IN S. POMBE Cdc2 | Serine/threonine kinase required for both G1/S and G2/M Cdc25 | Phosphatase specific for Cdc2 Wee1 | Dual specificity (tyrosine and threonine/serine) kinase PAUL NURSE’S SCREEN IN S. POMBE Adapted from Carneiro et al., 2010 REGULATION OF THE CELL CYCLE; ROLE OF CYCLINS AND CDKs Vermeulen et al. 2003 REGULATION OF THE CELL CYCLE; ROLE OF CYCLINS AND CDKs Three checkpoints safeguard the normal progression of the cell cycle; at G1-S, at G2-M, and at the exit of mitosis. Sperelakis, 2000 Lim and Kaldis, 2013 Lim and Kaldis, 2013 PROGRAMMED CELL DEATH (APOPTOSIS) Cell division has been found to be tightly controlled in association with other fundamental events in the cell's life, such as differentiation, senescence and programmed cell death. CELL MOTILITY Cellular motility is the spontaneous movement of a cell from one location to another by consumption of energy. The term encompasses several types of motion, including swimming, crawling, gliding and swarming. Cell motility is regarded as random cell movement while cell migration is a response to a cell attractant or repellent. Fritz-Laylin, 2020 CELL MOTILITY The two most prevalent forms of eukaryotic cell motility are flagellar-dependent swimming and actin-dependent cell migration, both of which are used by animal cells and unicellular eukaryotes alike. Flagellar motility is powered by whip-like organelles called flagella that propel cells through liquid or induce flow across surface-attached cells. Actin-dependent cell migration is driven by a variety of molecular mechanisms, all reliant on the dynamic turnover of actin networks that push and pull cells across or between solid surfaces Flagellar motility is often very rapid and microtubules are the basis of flagellar motility. Flagellar-based swimming in Batrachochytrium dendrobatidis Longitudinal section of a human sperm Crawling motility relies on the dynamic turnover of ephemeral actin polymer networks and are usually slower. Actin-dependent crawling in Naegleria gruberi MOTILITY IN SINGLE-CELLED ORGANISMS Many unicellular organisms rely on cell motility for basic functions such as sexual reproduction, hunting bacteria, as well as for their own unique purposes such as evading predation. Single-celled organisms move by a wide variety of distinct, and inventive, mechanisms, E.g. Many bacterial species use propeller-like appendages to swim, while some species of bacteria move by exuding glycosylated proteins that swell as they absorb water to propel the cell forward like a rocket engine. MOTILITY IN EUKARYOTIC Many unicellular organisms rely on cell motility for basic functions such as sexual reproduction, hunting bacteria, as well as for their own unique purposes such as evading predation. Single-celled organisms move by a wide variety of distinct, and inventive, mechanisms, E.g. Many bacterial species use propeller-like appendages to swim, while some species of bacteria move by exuding glycosylated proteins that swell as they absorb water to propel the cell forward like a rocket engine. MOTILITY IN EUKARYOTES VERSUS PROKARYOTES Whereas bacterial and archeal flagella are both assembled on the outside of the cell from unrelated proteins called flagellins, eukaryotic flagella, are assembled beneath the cell membrane from microtubules and hundreds of associated proteins. Bacterial and archeal flagella are powered by rotary motors at the base of the flagellum that cause the flagellum to twist. Eukaryotic flagella, in contrast, are powered throughout their length by the movement of dynein motors that cause microtubules to slide past each other and the entire flagellum to bend. CRAWLING MOTILITY: ACTIN POLYMERIZATION Involves the following three activities: 1. a pushing out of the membrane at the front of the cell to provide forward movement 2. contraction of the rear of the cell to keep the cell from simply flattening out indefinitely 3. interaction with one or more surfaces to provide traction, either through molecular adhesions, or by pushing against multiple surfaces. Lamellipodial motility relies on branched actin networks nucleated by the Arp2/3 complex. Elongation of these filaments pushes the front edge of the cell membrane forward. Blebbing motility results from dissociation of the plasma membrane from an underlying layer of actin called the ‘actin cortex’. Intracellular pressure then pushes out the plasma membrane to form a blister-like ‘bleb’. The actin cortex assembles under the bleb and the cycle repeats. Cortical flow arises when myosin motors contract actin networks at the rear of the cell, pulling the actin cortex backwards (purple arrows). Similar to how the backwards push on tank treads propel vehicles forward, cortical flow can result in forward movement of the cell. Filopodial motility uses actin bundles called filopodia that grow by adding actin monomers to their tips. Some cells build filopodia in conjunction with lamellipodia, while other cells use filopodia alone to move. THE CYTOSKELETON The cytoskeleton is a filamentous network that extends throughout the cell. Microtubules (MT) Intermediate filaments (IF)- only found in vertebrates Microfilaments (MF) or Actin filaments (AF) Two mouse fibroblast cells in interphase. Actin filaments (purple web), microtubules (yellow lines), and nuclei (green blobs in center) are labeled with fluorescent molecules. This image won first place in the Nikon 2003 Small World photo competition. Torsten Wittmann (2011), CIL:240. CIL. Dataset. https://doi.org/10.7295/W9CIL240. FUNCTIONS OF THE CYTOSKELETON Cell shape Act as an anchor for many proteins and organelles Resist mechanical stress Provide tracks for transport of vesicles, organelles, and other cargo Aid in the locomotion of many cell types They also help with the division of both the cytoplasm and the DNA during mitosis Microtubules (MT) https://open.oregonstate.education/cellbiology/chapter/cytoskeleton/ Microtubules (MT) Microtubules (MT) Intermediate filaments (IF) Intermediate filaments (IF) Intermediate filaments (IF) Actin filaments (AF) Actin filaments (AF) Actin filaments (AF) Actin filaments (AF) ANY QUESTIONS? APOPTOSIS CBG 223 Bodunrin O. Ottu Department of Cell Biology and Genetics, Room 203, Faculty of Science, University of Lagos. E: [email protected] May 2024 LEARNING OBJECTIVES At the end of the lecture, student should be able to understand the concept and characteristics of apoptosis and necrosis the role of caspases in apoptosis the pathways of apoptosis the importance of apoptosis INTRODUCTION – CELL INJURY AND CELL DEATH Cell injury results when cells are stressed so severely that they are no longer able to adapt. It also occurs when cells are exposed to damaging agents or suffer from intrinsic abnormalities. INTRODUCTION – CELL INJURY AND CELL DEATH Cell injury results when cells are stressed so severely that they are no longer able to adapt. It also occurs when cells are exposed to damaging agents or suffer from intrinsic abnormalities. Causes of cell injury include Chemical agents | conc. glucose or salt, air pollutants, insecticides, CO, asbestos and even drugs Infectious agents | viruses, tapeworms, bacteria, fungi, and protozoans Physical agents | trauma, extremes of temperatures, radiation, electric shock, and sudden changes in atmospheric pressure. Oxygen deprivation | hypoxia, or oxygen deficiency. Interferes with aerobic oxidative respiration. Genetic defects | SCA, inborn errors of metabolism Aging | cellular senescence leads to alterations in replicative and repair abilities of individual cells and tissues. Immune reactions | autoimmune reactions against one’s own tissues and allergic reactions against environmental substances. INTRODUCTION – CELL INJURY AND CELL DEATH Cell injury results when cells are stressed so severely that they are no longer able to adapt. It also occurs when cells are exposed to damaging agents or suffer from intrinsic abnormalities. Causes of cell injury include…. Types- Reversible cell injury and Cell death. INTRODUCTION – CELL INJURY AND CELL DEATH Reversible cell injury mild form of injury that causes reversible functional and morphologic changes or abnormalities if the damaging stimulus is removed. the injury has typically not progressed to severe membrane damage and nuclear dissolution. Cell death irreversible injury as a result of continuous damage such that the cell cannot recover and it dies. There are two types of cell death — necrosis and apoptosis. NECROSIS When damage to membranes is severe, enzymes leak out of lysosomes, enter the cytoplasm, and digest the cell, resulting in necrosis. Cellular contents also leak out through the damaged plasma membrane and elicit a host reaction (inflammation). Cell death due to physiological injury APOPTOSIS What makes a cell commit suicide? APOPTOSIS What makes a cell commit suicide? When a cell is deprived of positive signals e.g. growth factors When the cell’s DNA or proteins are damaged beyond repair Improper protein folding etc Causes of cell injury include…. APOPTOSIS Apoptosis is a pathway of cell death that is induced by a tightly regulated suicide program in which cells destined to die activate enzymes capable of degrading the cells’ own nuclear DNA as well as nuclear and cytoplasmic proteins. Caspases (cysteine-aspartic-acid-proteases) are involved in apoptosis and inflammation. Apoptosis is an active, energy-dependent, tightly regulated type of cell death Fragments of the apoptotic cells then break off, giving the appearance that is responsible for the name (apoptosis, “falling off”). APOPTOSIS – STEPS INVOLVED condensing of the cell nucleus and breaking it into pieces. breaking chromosomes into fragments containing multiple number of nucleosomes (a nucleosome ladder) condensing and fragmenting of cytoplasm into membrane bound apoptotic bodies phagocytosis APOPTOSIS – STEPS INVOLVED breaking chromosomes into fragments containing multiple number of nucleosomes (a nucleosome ladder) APOPTOSIS Alenzi, 2004 APOPTOSIS vs NECROSIS APOPTOSIS vs NECROSIS STRUCTURE OF CASPASES Abraham and Shaham, 2004 TYPES OF CASPASES Initiator caspases e.g. caspases -2, 8, 9 and 10. Executioner or effector caspases e.g. caspases -3, 6 and 7. Inflammatory caspases e.g. caspases -1, 4, 5. Examples given here are in mammals Regulatory proteins in caspase dependent apoptosis Bcl-2 family Anti-apoptotic Bcl-2, Bcl-x, Bcl-XL, Bcl-XS, Bcl-w, BAG Pro-apoptotic BH3, Bcl-10, Bax, Bak, Bad, Bid, Bim, Bik, and Blk Abraham and Shaham, 2004 Elmore, 2007 Elmore, 2007 TWO PATHWAYS TRIGGER APOPTOSIS DNA damage= ATM=p53=BAX TWO PATHWAYS TRIGGER APOPTOSIS DNA damage= ATM=p53=BAX INTRINSIC APOPTOTIC PATHWAY Ozaki and Nakagawara, 2011 Topham and Taylor, 2013 INTRINSIC AND EXTRINSIC PATHWAYS OF APOPTOSIS Ichim and Tait, 2016 Link… IMPORTANCE OF APOPTOSIS Embryogenesis - eliminates excess cells To destroy cells with DNA damage Cancer – p53 Cell homeostasis etc Abraham and Shaham, 2004 ANY QUESTIONS? Basics of Cells and Cell Signaling Basics of Cells and Cell Membrane A Brief History of Cells “Cellulae” in plant (dead cells) Robert Hooke (UK) 1665 Honeycomb structure Cell structure (“kernel”-nucleus) Robert Brown (UK) 1830s All plant tissues are composed Matthias Schleiden (German) of cells. 1838 Similar conclusions on animal Theodor Schwann 1839 (US) cells were reported All cells arise only from preexisting Rudolf Virchow (German) cells (Omnis cellula e cellula) 1855 Cell Theory Theodor Schwann posted two basic principles in 1839 (1) All organisms consist of one or more cells (2) The cells are the smallest living things and the basic units of structure for all organisms Rudolf Virchow added the third principle in 1855: (3) All cells arise only by division of a previously existing cells. Summary: The cell is not only the basic unit of structure for all organisms but also the basic unit of reproduction. Theodor Schwann: Schwann Cells and Cell Theory ▪ Between 1834-1838, Schwann isolated one type of cells that envelop nervous fibers. These cells were named Schwann cells ▪ In 1836, he discovered a enzyme in stomach, which is called pepsin. ▪ In 1839, he presented two principles on cells, which constitutes the important part of the Cell Theory Rudolf Virchow: leukemia and the cell theory ▪March 19, 1845 a Scottish physician John Bennett described an unusual case and diagnosed : “a suppuration of blood”. ▪After four month later Rudolf Virchow, (he was 24-year-old researcher and physician at that time) received a similar patient and recognized that the blood itself in the patient was abnormal. Virchow correctly identified the condition as blood disease, and named it leukämie in 1847 (later changed to leukemia). ▪After reported this case, Virchow moved to the area of cells and established the cell theory with Schwann. Cell Growth Based on cell theory, cell growth could be hyperplasia or hypertrophy: 1. Hyperplasia: cell growth by increasing in cell number. Such as liver, blood, the gut, and skin in adult animals. In these organs, hyperplasia is a major growth manner. 2. Hypertrophy: cell growth by increasing in cell size. Such as fat, muscle. Neoplasia: the abnormal growth of hyperplasia of cancer cells is called neoplasia. Hydrophilic and Hydrophobic Hydrophilic: Molecules that have an affinity for water and therefore dissolve readily in it are called hydrophilic. Most small organic molecules found in cells are hydrophilic, such as sugars, organic acids, and some of the amino acids. Hydrophobic: Molecules that are not very soluble in water are termed hydrophobic, such as lipid. If a ligand is hydrophilic, it must binds to its corresponding receptor on cell surface; If a ligand is hydrophobic, it can diffuse into cells and binds to the receptors located in the cytosol (or nucleus). These receptors are usually called nuclear receptors. Structure of Cell Membrane Structure of Cell Membrane ▪A membrane consists of lipids and membrane proteins. In most organisms other than bacteria, the membrane also contain sterols. ▪Most membrane lipids and proteins have both hydrophilic and hydrophobic regions and are amphipathic molecules. ▪As a result, the membrane is essentially a hydrophobic permeability barrier. Proteins Proteins have many physiological functions: structure components, enzymes, regulatory molecules, binding proteins etc. Protein kinase is a protein (enzyme) that modifies other proteins by chemically adding phosphate groups to them. Phosphatase is a protein (enzyme) that removes a phosphate group from its substrate (another protein/molecule) * * * * Cell Communication Cell-cell communication is important for organism survival and homeostasis. It governs basic cellular activities and coordinates cell actions. There are two types of communication ways: 1. Cell-cell Junctions (cell-cell physically interactions) tight junctions anchoring junctions gap junctions (in plant cells “plasmodesma”) Tight Junctions: multi-protein complexes hold cells of a same tissue together and prevent movement of water and water-soluble molecules between cells. For example: epithelial tissue. Anchoring Junctions: Extracellular portion of adhesion molecules (trans-membrane proteins) in a cell interacts with the extracellular portion of similar proteins on the surface of a neighboring cell. Cell-cell contact can also activate intracellular signaling pathway. Tight Junctions Anchoring Junctions Gap Junctions: There are a big gap between two cells but allows exchange of ions and some molecules between cells. (in plants: the gap junction is called plasmodesma). Animal cells Plat cells 2. Signal Transduction The process that converts a extra cellular signal (soluble chemical molecules) into a preprogramed sequence of events within cells, which will finally lead to gene transcription. ▪Phosphorylation and dephosphorylation ▪Signal cascade and amplification ▪ Local regulation (such as growth factors and paracrine hormones) ▪ Distal regulation (such as endocrine hormones which are secreted by glands and transported via blood). local regulation distal regulation Primary Message: Electrical Signals and Chemical Signals Electrical signals (neuron signaling): electrical impulses (action potentials) are transmitted along the specialized plasma membranes of nerve cells. The transmit speed is remarkably high. The communication through the electrical signals is mainly found in neuron cells, muscle cells, and gland cells. Chemical Signal The signaling cascade is induced by binding of chemical molecules (growth factors, neuron transmitters, hormones) to their corresponding receptors either located on the cell plasm membrane or cytosol/nucleus. Here is an example of common chemical stimuli (primary message): 1.Growth Factors and cytokines Growth Factors: Epidermal growth factors (EGFs) Transforming growth factor-α (TGFα) Insulin-like growth factors (IGFs)? Fibroblast growth factors (FGFs) Hepatocyte growth factor (HGF) Platelet-derived growth factors (PDGFs) Nerve growth factor (NGF) Cytokines Interleukins (IL) IL-1, IL-2, IL-3, IL-5, IL-6, IL-10, and IL-21 Interferons (IFNs) IFN-α, IFN-β, IFN-γ Colony-stimulating factors (CSFs), Colony-stimulating factor 1 (CSF-1). Erythropoietin (EPO) Granulocyte colony-stimulating factor (G-CSF) Granulocyte-macrophage colony-stimulating factor(GM-CSF) Leukaemia inhibitory factor (LIF) Stem cell factor (SCF) Thrombopoietin (TPO) Tumour necrosis factor α (TNFα) 2. Neurotransmitters Acetylcholine Dopamine. γ-Aminobutyric acid (GABA) 3. Hormones Insulin Progesterone Estrogen Glucocorticoid , Androgen Retinoic Acids Vitamin D3 Epinephrine Norepinephrine Rita Levi-Montalcini and Nerve growth factor (NGF) After graduated from a university in Italy, Rita Levi-Montalcini remained in the university as a Assistant. After two years she lost this job. During world war II she set up a laboratory in her bedroom and studied the growth of nerve fibers in chicken embryos. In 1946, Montalcini got a one-semester job from Washington University in St. Louis. After she duplicated her home work, she was offered a research associate job. In 1952 she isolated nerve growth factor (NGF) from certain cancerous tissues that caused extremely rapid growth of nerve cells. In 1986 Levi-Montalcini was awarded Nobel Prize in Physiology or Medicine jointly with Stanley Cohen for the discovery of NGF. Secondary messenger The binding of ligand with receptor on the cell surface often results in the production of some additional molecules within the cells, which play a critical role in transmitting signals from extracellular signaling and inducing signal cascade. Discovery of cAMP (second message) and Hormone Action Mechanisms. ▪Earl Wilbur Sutherland, Jr. (1915-1974), who together with colleague Theodore W. Rall, isolated cyclic adenosine monophosphate (cyclic AMP) in 1956 when he worked on epinephrine induced degradation of glycogen. ▪Following its isolation, he demonstrated the involvement of cyclic AMP in numerous metabolic processes including the activation of liver phosphorylase (LP) in the process of glycogenolysis. ▪In 1971 Earl Wilbur Sutherland was awarded the Nobel Prize in Physiology or Medicine for his discoveries concerning the mechanisms of the hormone action and discovery of cAMP when he was in Case Western Reserve University. Classifications of Signal Transducing Receptors I. Cell Surface (Transmembrane) Receptors: These receptors are located in the cell plasma membrane. 1) Ion channel linked receptors. The receptors function as an ion channel. Ligand binding causes the channel open or close. Therefore, they are also called ligand-gated ion channels. This type of signaling events are usually found in electrically active cells and controlled by action potential and neurotransmitters. 2) Enzyme-linked receptors. These receptors have enzymatic activity in their intracellular domain and are capable of autophosphorylation and phosphorylation of target molecules in the cytosol. There are two types of enzyme-linked receptors: ▪ receptor tyrosine kinase ▪ receptor serine/threonine kinase. Example of receptor tyrosine kinase 3)G-protein linked receptor: these receptors do not have kinase activity but couple with G-protein in their intracellular domain. Activated G-protein by the receptors then activates a enzyme. 4) Other receptors (Non-ligand gated channel, Non-G protein-linked receptor, non-enzyme linked receptors) Example: death receptors. The signal pathway usually has little phosphorylation or dephosphorylation process. II. Nuclear receptors These receptors are located in cytosol and nucleus. They function as transcription factors, because they can enter the nucleus, bind to DNA, and regulate DNA transcription. Nuclear receptors usually have two binding sites: one for ligand and one for DNA. What is a transcription factor? A molecule(s) (protein) that can bind DNA (usually at promoter region) and regulate DNA transcription is called transcription factor. Fig. Diagram of Signal transduction pathway induced by activation of cell surface receptor Fig. Diagram of signaling pathway induced by activation of nuclear receptors. Key concept Major Information about cell theory Hyperplasia and hypertrophy Metastases, neoplasia Hydrophilic and hydrophobic Membrane structure Protein kinase and protein phosphatases Primary and secondary messages Cell surface receptors Receptor tyrosine kinase Receptor serine/threonine kinase G-protein-linked receptors Nuclear receptors Transport across cell membrane Types of cell membrane transport Factors affecting transport Cell membrane Chemical gradient Electrical gradient Rate of transport Passive transport Diffusion Osmosis Facilitated diffusion Active transport Pumps phagocytosis Endocytosis/exocytosis Factors affecting transport: cell membrane The cell needs to absorb and excrete various compounds throughout its life. These compounds need to pass through the membrane which is made from a phospholipid bilayer The phospholipid bilayer is formed by phospholipid molecules bipolar molecule: the fatty acid side is hydrophobic, the phosphoric side is hydrophilic The membrane is permeable The membrane is impermeable to: to: ❖ Small, charged molecules ❖ H2O ❖ Gases (O2, CO2, N2) ❖ “large molecules” such as ❖ Lipids amino acids, glucose and ❖ Small, neutral molecules (such larger as urea) ❖ These compounds must go through channels present in the membrane in order to enter or exit the cell Factors affecting transport: Chemical gradient Compound moves from an area of high concentration to low concentration (or concentration gradient) All compounds permeable to the phospholipid bilayer will move this way Factors affecting transport: Electrical force Positive ions are attracted to negative ions and vice versa Ions are repelled by ions of the same charge (+ against + and – against -) Movement across the cell membrane Both chemical and electrical forces (electrochemical force) drive the movement of compounds across the cell membrane Factors affecting the rate of transport The rate of transport will depend on: ❖ The concentration gradient ❖ The compound permeability to the membrane ❖ The type and number of charges present on the compound Crossing the cell membrane – fats and oils can pass directly through inside cell lipid sat waste sugar aa H2O outside cell Types of Transport Proteins Channel proteins are embedded in the cell membrane & have a pore for materials to cross Carrier proteins can change shape to move material from one side of the membrane to the other Cell membrane channels Need to make “doors” through membrane – protein channels allow substances in & out specific channels allow specific material in & out H2O channel, salt channel, sugar channel, etc. inside cell outside cell Protein channels Proteins act as doors in the membrane channels to move specific molecules through cell membrane HIGH LOW Passive transport Compounds will move from area of high concentration toward area of lower concentration No ATP is needed for this type of transport Passive transport mainly TWO types A-Osmosis B-Diffusion-diffusion again two types a-simple diffusion- no energy needed b- facilitated diffusion- no energy needed -help through a protein channel Osmosis Each compound obeys the law of diffusion diffusion of water from HIGH concentration of water to LOW concentration of water across a semi-permeable membrane However, some compounds are unable to cross the cell membrane (glucose, electrolytes…) Water can cross will enter or exit the cell depending its concentration gradient. where is osmosis important Cells in Solutions PLASMOLYSIS Isotonic Solution Hypotonic Hypertonic Solution Solution NO NET MOVEMENT OF H2O (equal amounts entering & leaving) CYTOLYSIS PLASMOLYSIS Diffusion Simple diffusion- no energy needed Movement across higher to lower concentration gradient. Facilitated diffusion- Some compounds are unable to diffuse through the membrane. They will be allow to cross if the membrane has proteins that can bind these compounds and enable to cross toward the area of lower concentration Simple and facilitated diffusion simple diffusion facilitated diffusion lipid inside cell inside cell H2O protein channel H2O outside cell outside cell Simple Diffusion ❖ Doesn’t require energy ❖ Moves high to low concentration ❖Example: Oxygen or water diffusing into a cell and carbon dioxide diffusing out. Simple Diffusion The rate of diffusion will be increased when there is : Concentration: the difference in between two areas (the gradient) causes diffusion. The greater the difference in concentration, the faster the diffusion. Molecular size: smaller substances diffuse more quickly. Large molecules (such as starches and proteins) simply cannot diffuse through. Shape of Ion/Molecule: a substance’s shape may prevent it from diffusing rapidly, where others may have a shape that aids their diffusion. Viscosity of the Medium: the lower the viscosity, the more slowly molecules can move through it. Movement of the Medium: currents will aid diffusion. Like the wind in air, cytoplasmic steaming (constant movement of the cytoplasm) will aid diffusion in the cell. Solubility: lipid - soluble molecules will dissolve through the phospholipid bilayer easily, as will gases like CO2 and O2. Polarity: water will diffuse, but because of its polarity, it will not pass through the non-polar phospholipids. Instead, water passes though specialized protein ion channels Facilitated diffusion ❖Doesn’t require energy ❖Uses transport proteins to move high to low concentration Examples: Glucose or amino acids moving from blood into a cell. where is facilitated transport important Active Transport - Pumps - phagocytosis - Endocytosis/exocytosis Active transport ❖ATP (energy) is needed → pump ❖Moves materials from LOW to HIGH concentration ❖AGAINST concentration gradient Example-1 ATPase pumps The most common: Na/K pumps re- establish membrane potential. Present in all cells. Two K+ ions are exchanged with 3 Na + ions EXAMPLES OF ACTIVE TRANSPORT Example 2: the thyroid gland accumulates iodine as it is needed to manufacture the hormone thyroxin. The iodine concentration can be as much as 25 times more concentrated in the thyroid than in blood. Example 3: In order to make ATP in the mitochondria, a proton pump (hydrogen ion) is required. where is active transport important Endocytosis Endocytosis: (“Endo” means “in”). Endocytosis is the taking in of molecules or particles by invagination of the cell membrane forming a vesicle. Integrity of plasma membrane is maintained. This requires energy. Endocytosis is fallowed by exocytosis on the other side. – Transcytosis, vesicle trafficking, or cytopempsis. There are two types of endocytosis 1. pinocytosis (cell drinking): small molecules are ingested and a vesicle is immediately formed. This is seen in small intestine cells (villi) 2. phagocytosis (cell eating): large particles, (visible with light microscope) are invaginated into the cell (ie: white blood cells ‘eat’ bacteria Phagocytosis Used to engulf large particles such as food, bacteria, etc. into vesicles Called “Cell Eating Receptor-Mediated Endocytosis Some integral proteins have receptors on their surface to recognize & take in hormones, cholesterol, etc. Exocytosis Exocytosis: (“Exo” means “out”.) Exocytosis is the reverse of endocytosis. This is where a cell releases the contents of a vesicle outside of the cell. These contents may be wastes, proteins, hormones, or some other product for secretion. This also requires energy. Example: vesicles from the Golgi fuse with the plasma membrane and the proteins are released outside of the cell. Fusion of vesicle with plasma membrane is mediated by a number of accessory proteins- SNARE protein. Require stimulus and Ca. Exception- Renin from JG cells and PTH from parathyroid gland by decrease in intracellular Ca. Constitutive Secretion- Immunoglobulin from plasma Cells and collagen from fibroblast. Regulated- endocrine gland, pancreatic acinar cells Membrane Transport Proteins 1. Water Channels or Aquaporins (AQPs) – 12 types Amount of water is regulated by No. of AQPs They are known as gated channel although they are pores. Two types a) Aquaporins- only water. b) Aquaglyceroporins- also for small molecules. 2- Ion Channels- All cells specially on excitable cells – Neurons and muscle cells Selective and non selective Gated – voltage gated and extracellular agonist or antagonist gated ex – acetylcholine gated cationic specific channel at motor end plate of skeletal muscle. Conductance- 1-2 picosimens and > 100 picosimens. Ex- Na, K, Ca, Cl, Anion , cation. 3.Solute Carriers- > 40 types , > 300 transporters. three gps-1. Uniporters- single molecule across the membrane (GLUT ) 2. Symporters- Two or more molecules Ex- Na-k-cl Symporter (Kidney) Na - Glucose Cotransporter. 3. Antiporters- Two or more molecules in opposite directions Ex :Na- H antiporter ( PH regulation) 3Na- Ca , Cl- HCO3 4.ATP DEPENDENT TRANSPORTERS 1. ATPase Ion Transporters 1. P- Type- gate phosphorylted during transport. Na- K ATP ase. 2. V- Type- Vacuolar H- ATPase – urine acidification on Vacules like endosomes and lysosomes. 2. ATP – binding cassette (ABC) transporters – 7 subgroups transport diverse group of ions ex- Cl, Cholesterol, bile acids, drugs, iron and organic anions. EX:- Cystic fibrosis transmembrane regulator. Multidrug Resistance Protein. organic Anions.. Molecular Motors: Kinesin- over the microtubule Dynein- retrogate transport Myosin- over the microfilaments.- 18 types a VISUAL TRANSDUCTION Overview of Questions How do our eyes respond to light? Why do our eyes have two different sets of receptors – rods and cones? Fig.1 The electromagnetic spectrum, showing the wide range of energy in the environment and the small range within this spectrum, called visible light, that we can see. Light is the Stimulus for Vision Electromagnetic spectrum – Energy is described by wavelength. – Spectrum ranges from short wavelength gamma rays to long wavelength radio waves. – Visible spectrum for humans ranges from 400 to 700 nanometers. – Most perceived light is reflected light of surfaces. Fig.2 An image of the cup is focused on the retina, which lines the back of the eye. The close-up of the retina on the right shows the receptors and other neurons that make up the retina. Focusing Images on the Retina The cornea, which is fixed, accounts for about 80% of focusing. The lens, which adjusts shape for object distance, accounts for the other 20%. – Accommodation results when ciliary muscles are tightened which causes the lens to thicken. Light rays pass through the lens more sharply and focus near objects on retina. Eye Structures Fig. 3 Cross section of the vertebrate eye Note how an object in the visual field produces an inverted image on the retina. Light goes through other layers of neurons before being transduced at the rod and cone photoreceptors Photoreceptors send the light information to the bipolar cells, which send it to the ganglion cells, and the information exits the retina to the brain Horizontal cells and amacrine cells modulate the information Blind Spot Blind spot - place where optic nerve leaves the eye –We don’t see it because: one eye covers the blind spot of the other. it is located at edge of the visual field. the brain “fills in” the spot. Fig.4 There are no receptors at the place where the optic nerve leaves the eye. This enables the receptor’s ganglion cell fibers to flow into the optic nerve. The absence of receptors in this area creates the blind spot. Visual Coding and Retinal Receptors The Eye and Its Connections to the Brain Pupil-opening in the center of the eye that allows light to pass through Lens-focuses the light on the retina Retina-back surface of the eye that contains the photoreceptors The Fovea-point of central focus on the retina blind spot-the point where the optic nerve leaves the eye Diseases that Affect the Retina Macular degeneration –Fovea and small surrounding area are destroyed –Creates a “blind spot” on retina –Most common in older individuals –“Wet” causes vision loss due to abnormal blood vessel growth –“Dry” results from atrophy of the retinal pigment, which causes vision loss through loss of photoreceptors Awareness. Most stimuli that are received, transduced and coded are then perceived. E.g, when smelling a flower, scent molecules strike olfactory receptors in the nose (reception). This produces a chemical reaction that depolarizes the resting potentials of the olfactory receptors, they fire (transduction) and this information is passed via the olfactory nerve to the olfactory bulb at the base of the brain (coding). The olfactory bulb then sends connections to various parts of prefrontal cortex where smells are recognised (awareness). The Visual System. Light enters the eye through an opening in the centre of the iris called the pupil. The light is focused by the cornea and lens and projected onto the retina - the light sensitive cells that line the rear of the eyeball. Light from the top left of the visual scene strikes the bottom right of the retina and vice versa so the visual image on the retina is upside down and reversed. The centre of the retina is called the macula and this is the most sensitive part of the retina used for resolving fine detail. The most precise region of visual analysis takes place within the macula at the fovea, a small region where receptors are tightly packed. Photoreceptors A photoreceptor cell is a specialized type of neuron found in the retina that is capable of photo transduction. they convert light (visible electromagnetic radiation) into signals that can stimulate biological processes.there are two types of photoreceptors:- Rods cones Rods are highly sensitive to light, and thus are good for dim light or night vision. They are able to capture more light, but they do not respond well to moving stimuli because their response time is slow. Cones are not as sensitive to dim light, and are able to respond quickly, and therefore transduce moving stimuli. They are used more in daytime vision, and also are able to detect color because three classes of cones express three different photopigments. Cones are more concentrated in the fovea of the retina. How much light does it take? Prior physical evidence showed that it takes one photon to isomerize a pigment molecule. Purpose of Hecht experiment - to determine how many pigment molecules need to be isomerized for a person to see Fig. 5 The observer in Hecht et al.’s (1942) experiment could see a spot of light containing 100 photons. Of these, 50 photons reached the retina, and 7 photons were absorbed by visual pigment molecules. Experiment by Hecht Results showed: –a person can see a light if seven rod receptors are activated simultaneously. –a rod receptor can be activated by the isomerization of just one visual pigment molecule. Rods and Cones Differences between rods and cones ◦ Shape Rods - large and cylindrical Cones - small and tapered ◦ Distribution on retina Fovea consists solely of cones. Peripheral retina has both rods and cones. ◦ More rods than cones in periphery. ◦ Number - about 120 million rods and 5 million cones How do our eyes convert light into neural signal? Transduction First take a look at receptors we find in the retina. Visual Pigment Regeneration Process needed for transduction: –Retinal molecule changes shape –Opsin molecule separates –The retina shows pigment bleaching. –Retinal and opsin must recombine to respond to light. –Cone pigment regenerates in six minutes. –Rod pigment takes over 30 minutes to regenerate. Rods and Cones Fig. 6 Fig. 7 (a) Rod receptor showing discs in the outer segment. (b) Close-up of one disc showing one visual pigment molecule in the membrane. (c) Close-up showing how the protein opsin in one visual pigment molecule crosses the disc membrane seven times. The light-sensitive retinal molecule is attached to the opsin at the place indicated. Each disk contains:- 1- photopigment (rhodopsin) 2- G protein transducin 3-CGMP phosphodiesterase enzyme Transduction of Light into Nerve Impulses Rod Receptor have outer segments, which contain: – Visual pigment molecules, which have two components: Opsin - a large protein Retinal - a light sensitive molecule Visual transduction occurs when the retinal absorbs one photon. – Retinal changes it shape, called isomerization. Fig. 8 Model of a visual pigment molecule. The horizontal part of the model shows a tiny portion of the huge opsin molecule near where the retinal is attached. The smaller molecule on top of the opsin is the light-sensitive retinal. The model on the left shows the retinal molecule’s shape before it absorbs light. The model on the right shows the retinal molecule’s shape after it absorbs light. This change in shape is one of the steps that results in the generation of an electrical response in the receptor. Transduction in Rods Absorption of light causes rhodopsin to break into opsin and retinal. Enzymes break down cyclic GMP. Fewer sodium channels remain open. Cell hyperpolarizes in light (it’s depolarized in the dark). Photoreceptors produce graded potentials. Visual Transduction: The Conversion of Physical Energy to Neural Energy Photopigments are located in the membrane of the outer segment of rods and cones Each pigment consists of an opsin (a protein) and retinal (vitamin A derivative) In the dark, membrane NA+/CA++ channels are open -> glutamate is released which depolarizes the membrane (K+ channels are also open: net effect results in depolarization) Light splits the opsin and retinal apart-> Activates transducin (G protein)-> Activates phosphodiesterase-> Reduces cGMP -> closes NA+/CA++ channels (K+ remain open) The net effect of light is to hyperpolarize the retinal receptor and reduce the release of glutamate Fig. 9 Phototransduction in rod photoreceptors Fig. 10 Rhodopsin (from rods) is a combination of retinal, the light- absorbing molecule, and a large protein called opsin. The opsins are closely related in structure to the G- protein-coupled receptors. Fig. 11 (A) Phototransduction - dark current AMPLIFICATION! One rhodopsin molecule absorbs one photon 500 transducin molecules are activated 500 phosphodiesterase molecules are activated 105 cyclic GMP molecules are hydrolyzed 250 cation channels close 106-107 Na+ ions per second are prevented from entering the cell for a period of ~1 second rod cell membrane is hyperpolarized by 1 mV Phototransduction - dark currentLight hyperpolarizes the photoreceptor With light stimulation, the cGMP channels are closed. Pathway: light rhodopsin rhodopsin transducin transducin phosphodiesterase phosphodiesterase cGMP cGMP cGMP-gated channel Mechanisms of Receptor Regulation D D D D P P P P P P Arrestin (2) Phosphorylation Arrestin α β α α γ (3) Arrestin (4) Clustering in Clathrin binding clathrin-coated (7) Recycling pits (1) Agonist binding and G protein (5) Endocytosis activation Endosomes D P P (6) Dissociation of agonist: Arrestin (8) Traffic to Dephosphorylation lysosomes Sorting between cycling Lysosomes and lysosomal pathways Light Transduction DARK LIGHT trans-retinal transformed to cis-retinal cis-retinal transformed to trans-retinal cis-retinal and opsin form rhodopsin trans-retinal and opsin dissociate rhodopsin activates guanylate cyclase (GC) now active opsin activates transducin GC increases the synthesis of cGMP transducin activates PDE cGMP opens Na+ channels PDE breaks down cGMP to 5’-GMP rod cell depolarizes 5’GMP closes Na+ channels increases the release of glutamate rod cell hyperpolarizes (darkness adjustment–waiting for rhodosin) reduces the release of glutamate Rhodopsin Cascade Rhodopsin molecule LIGHT Rod cell disc Inside Rod cell Outside 1 photon of light can block the entry of 1,000,000 Na+ ions Basics of Cells and Cell Signaling Basics of Cells and Cell Membrane A Brief History of Cells “Cellulae” in plant (dead cells) Robert Hooke (UK) 1665 Honeycomb structure Cell structure (“kernel”-nucleus) Robert Brown (UK) 1830s All plant tissues are composed Matthias Schleiden (German) of cells. 1838 Similar conclusions on animal Theodor Schwann 1839 (US) cells were reported All cells arise only from preexisting Rudolf Virchow (German) cells (Omnis cellula e cellula) 1855 Cell Theory Theodor Schwann posted two basic principles in 1839 (1) All organisms consist of one or more cells (2) The cells are the smallest living things and the basic units of structure for all organisms Rudolf Virchow added the third principle in 1855: (3) All cells arise only by division of a previously existing cells. Summary: The cell is not only the basic unit of structure for all organisms but also the basic unit of reproduction. Theodor Schwann: Schwann Cells and Cell Theory ▪ Between 1834-1838, Schwann isolated one type of cells that envelop nervous fibers. These cells were named Schwann cells ▪ In 1836, he discovered a enzyme in stomach, which is called pepsin. ▪ In 1839, he presented two principles on cells, which constitutes the important part of the Cell Theory Rudolf Virchow: leukemia and the cell theory ▪March 19, 1845 a Scottish physician John Bennett described an unusual case and diagnosed : “a suppuration of blood”. ▪After four month later Rudolf Virchow, (he was 24-year-old researcher and physician at that time) received a similar patient and recognized that the blood itself in the patient was abnormal. Virchow correctly identified the condition as blood disease, and named it leukämie in 1847 (later changed to leukemia). ▪After reported this case, Virchow moved to the area of cells and established the cell theory with Schwann. Cell Growth Based on cell theory, cell growth could be hyperplasia or hypertrophy: 1. Hyperplasia: cell growth by increasing in cell number. Such as liver, blood, the gut, and skin in adult animals. In these organs, hyperplasia is a major growth manner. 2. Hypertrophy: cell growth by increasing in cell size. Such as fat, muscle. Neoplasia: the abnormal growth of hyperplasia of cancer cells is called neoplasia. Hydrophilic and Hydrophobic Hydrophilic: Molecules that have an affinity for water and therefore dissolve readily in it are called hydrophilic. Most small organic molecules found in cells are hydrophilic, such as sugars, organic acids, and some of the amino acids. Hydrophobic: Molecules that are not very soluble in water are termed hydrophobic, such as lipid. If a ligand is hydrophilic, it must binds to its corresponding receptor on cell surface; If a ligand is hydrophobic, it can diffuse into cells and binds to the receptors located in the cytosol (or nucleus). These receptors are usually called nuclear receptors. Structure of Cell Membrane Structure of Cell Membrane ▪A membrane consists of lipids and membrane proteins. In most organisms other than bacteria, the membrane also contain sterols. ▪Most membrane lipids and proteins have both hydrophilic and hydrophobic regions and are amphipathic molecules. ▪As a result, the membrane is essentially a hydrophobic permeability barrier. Proteins Proteins have many physiological functions: structure components, enzymes, regulatory molecules, binding proteins etc. Protein kinase is a protein (enzyme) that modifies other proteins by chemically adding phosphate groups to them. Phosphatase is a protein (enzyme) that removes a phosphate group from its substrate (another protein/molecule) * * * * Cell Communication Cell-cell communication is important for organism survival and homeostasis. It governs basic cellular activities and coordinates cell actions. There are two types of communication ways: 1. Cell-cell Junctions (cell-cell physically interactions) tight junctions anchoring junctions gap junctions (in plant cells “plasmodesma”) Tight Junctions: multi-protein complexes hold cells of a same tissue together and prevent movement of water and water-soluble molecules between cells. For example: epithelial tissue. Anchoring Junctions: Extracellular portion of adhesion molecules (trans-membrane proteins) in a cell interacts with the extracellular portion of similar proteins on the surface of a neighboring cell. Cell-cell contact can also activate intracellular signaling pathway. Tight Junctions Anchoring Junctions Gap Junctions: There are a big gap between two cells but allows exchange of ions and some molecules between cells. (in plants: the gap junction is called plasmodesma). Animal cells Plat cells 2. Signal Transduction The process that converts a extra cellular signal (soluble chemical molecules) into a preprogramed sequence of events within cells, which will finally lead to gene transcription. ▪Phosphorylation and dephosphorylation ▪Signal cascade and amplification ▪ Local regulation (such as growth factors and paracrine hormones) ▪ Distal regulation (such as endocrine hormones which are secreted by glands and transported via blood). local regulation distal regulation Primary Message: Electrical Signals and Chemical Signals Electrical signals (neuron signaling): electrical impulses (action potentials) are transmitted along the specialized plasma membranes of nerve cells. The transmit speed is remarkably high. The communication through the electrical signals is mainly found in neuron cells, muscle cells, and gland cells. Chemical Signal The signaling cascade is induced by binding of chemical molecules (growth factors, neuron transmitters, hormones) to their corresponding receptors either located on the cell plasm membrane or cytosol/nucleus. Here is an example of common chemical stimuli (primary message): 1.Growth Factors and cytokines Growth Factors: Epidermal growth factors (EGFs) Transforming growth factor-α (TGFα) Insulin-like growth factors (IGFs)? Fibroblast growth factors (FGFs) Hepatocyte growth factor (HGF) Platelet-derived growth factors (PDGFs) Nerve growth factor (NGF) Cytokines Interleukins (IL) IL-1, IL-2, IL-3, IL-5, IL-6, IL-10, and IL-21 Interferons (IFNs) IFN-α, IFN-β, IFN-γ Colony-stimulating factors (CSFs), Colony-stimulating factor 1 (CSF-1). Erythropoietin (EPO) Granulocyte colony-stimulating factor (G-CSF) Granulocyte-macrophage colony-stimulating factor(GM-CSF) Leukaemia inhibitory factor (LIF) Stem cell factor (SCF) Thrombopoietin (TPO) Tumour necrosis factor α (TNFα) 2. Neurotransmitters Acetylcholine Dopamine. γ-Aminobutyric acid (GABA) 3. Hormones Insulin Progesterone Estrogen Glucocorticoid , Androgen Retinoic Acids Vitamin D3 Epinephrine Norepinephrine Rita Levi-Montalcini and Nerve growth factor (NGF) After graduated from a university in Italy, Rita Levi-Montalcini remained in the university as a Assistant. After two years she lost this job. During world war II she set up a laboratory in her bedroom and studied the growth of nerve fibers in chicken embryos. In 1946, Montalcini got a one-semester job from Washington University in St. Louis. After she duplicated her home work, she was offered a research associate job. In 1952 she isolated nerve growth factor (NGF) from certain cancerous tissues that caused extremely rapid growth of nerve cells. In 1986 Levi-Montalcini was awarded Nobel Prize in Physiology or Medicine jointly with Stanley Cohen for the discovery of NGF. Secondary messenger The binding of ligand with receptor on the cell surface often results in the production of some additional molecules within the cells, which play a critical role in transmitting signals from extracellular signaling and inducing signal cascade. Discovery of cAMP (second message) and Hormone Action Mechanisms. ▪Earl Wilbur Sutherland, Jr. (1915-1974), who together with colleague Theodore W. Rall, isolated cyclic adenosine monophosphate (cyclic AMP) in 1956 when he worked on epinephrine induced degradation of glycogen. ▪Following its isolation, he demonstrated the involvement of cyclic AMP in numerous metabolic processes including the activation of liver phosphorylase (LP) in the process of glycogenolysis. ▪In 1971 Earl Wilbur Sutherland was awarded the Nobel Prize in Physiology or Medicine for his discoveries concerning the mechanisms of the hormone action and discovery of cAMP when he was in Case Western Reserve University. Classifications of Signal Transducing Receptors I. Cell Surface (Transmembrane) Receptors: These receptors are located in the cell plasma membrane. 1) Ion channel linked receptors. The receptors function as an ion channel. Ligand binding causes the channel open or close. Therefore, they are also called ligand-gated ion channels. This type of signaling events are usually found in electrically active cells and controlled by action potential and neurotransmitters. 2) Enzyme-linked receptors. These receptors have enzymatic activity in their intracellular domain and are capable of autophosphorylation and phosphorylation of target molecules in the cytosol. There are two types of enzyme-linked receptors: ▪ receptor tyrosine kinase ▪ receptor serine/threonine kinase. Example of receptor tyrosine kinase 3)G-protein linked receptor: these receptors do not have kinase activity but couple with G-protein in their intracellular domain. Activated G-protein by the receptors then activates a enzyme. 4) Other receptors (Non-ligand gated channel, Non-G protein-linked receptor, non-enzyme linked receptors) Example: death receptors. The signal pathway usually has little phosphorylation or dephosphorylation process. II. Nuclear receptors These receptors are located in cytosol and nucleus. They function as transcription factors, because they can enter the nucleus, bind to DNA, and regulate DNA transcription. Nuclear receptors usually have two binding sites: one for ligand and one for DNA. What is a transcription factor? A molecule(s) (protein) that can bind DNA (usually at promoter region) and regulate DNA transcription is called transcription factor. Fig. Diagram of Signal transduction pathway induced by activation of cell surface receptor Fig. Diagram of signaling pathway induced by activation of nuclear receptors. Key concept Major Information about cell theory Hyperplasia and hypertrophy Metastases, neoplasia Hydrophilic and hydrophobic Membrane structure Protein kinase and protein phosphatases Primary and secondary messages Cell surface receptors Receptor tyrosine kinase Receptor serine/threonine kinase G-protein-linked receptors Nuclear receptors Cell Physiology CBG 223 Olfaction and Gustation By: Dr. O. O. Iroanya Olfaction and Gustation Olfaction and gustation make up the chemical senses. They provide information about the external environment. These two sensory systems are anatomically and morphologically different. Olfaction and gustation share certain common features, e.g. transduction mechanisms in 2 of these chemical senses involve G-protein-coupled receptors and changes in intracellular cyclic nucleotides, particularly cAMP. Olfaction and Gustation Their specialized sensory receptors are stimulated by chemical molecules, and the functions of the two systems often complement each other as special visceral afferents. Taste and smell are the principal systems for distinguishing flavors. Flavor of foods is dependent upon the 1. Oral sensory system 2. Salivary secretion and 3. Mastication e.g. wine tasters typically depend on the sensation of taste (flavor) and olfaction (smell) to distinguish between different wines. GUSTATION Gustation is the special sense associated with the tongue. Tactile, thermal, and nociceptive sensory input from the oral mucosa contributes to the quality of food. Saliva also is a vital factor in maintaining acuity of taste receptor cells. Saliva acts as a solvent for polar solutes, transports solutes to the taste receptors, acts as buffer for acidic foods and has a reparative action on the lingual epithelium. Gustation This is the special sense associated with the tongue. The surface of the tongue and all the oral cavity, is lined by a stratified squamous epithelium. Papillae are raised bumps on the tongue that contains the structures for gustatory transduction. Each taste bud is flask-like in shape, its broad base resting on the corium, and its neck opening, the gustatory pore, between the cells of the epithelium. The taste bud is formed by two kinds of cells e.g. supporting cells and gustatory cells. Basic / Primary tastes There are five basic tastes that the tongue is sensitive to are 1. salt 2. Sweet 3. Bitter 4. Sour 5. Umami (savory or meaty) a Japanese word that means “delicious taste,”, the detection of glutamates Taste Our perception of the 5 basic taste qualities in the gustatory system, depends on taste transduction pathways, through Taste receptor cells G proteins ion channels Effector enzymes. Taste sensations Sweet, bitter, and umami, work with a signal through a G protein-coupled receptor. Salty and sour, work with ion channels. Transduction of the five tastes happens through different mechanisms that reflect the molecular composition of the tastant e.g. A salty tastant (containing NaCl) provides the sodium ions (Na+) that enter the taste neurons, exciting them directly. Sour tastants are acids which belong to the thermoreceptor protein family. Binding of an acid or other sour-tasting molecule triggers a change in the ion channel which increases hydrogen ion (H+) concentrations in the taste neurons; thus, depolarizing them. Sweet, bitter, and umami tastants require a G-protein-coupled receptor. These tastants bind to their respective receptors, thereby exciting the specialized Gustatory system Scientists describe seven tastes - Astringent, Bitter, pungent (e.g. chili), Salty, Sour, Sweet, Umami The taste buds contain the receptors for taste and are located around the papillae. They are involved in detecting the five (known) elements of taste perception: bitter, salty, sour, sweet, and umami. Parts of the food dissolved in saliva come into contact with taste receptors through taste pores (small openings in the tongue epithelium). Information detected by clusters of various receptors and ion channels are sent by the taste receptor cells to the gustatory areas of the brain through the seventh, ninth and tenth cranial. nerves. Taste buds are found within the structure of the papillae and taste cells are replaced approximately every 10 days Taste buds contain specialized gustatory receptor cells used in transduction of taste stimuli. These gustatory receptor cells are sensitive to the chemicals contained within foods that are ingested, and they release neurotransmitters based on the amount of the chemical in the food. Neurotransmitters from the gustatory cells can activate sensory neurons in the facial, glossopharyngeal, and vagus cranial nerves. Types of papillae There are four types of papillae This classification is based on their appearance 1. Circumvallate - most people have only about 10 to 14 Circumvallate papillae. They are found at the back of the oral part of the tongue and are arranged in a circular-shaped row just in front of the sulcus terminalis of the tongue. Its are associated with ducts of Von Ebner’s glands, and are innervated by the glossopharyngeal nerve. 2. Filiform - are thin, long large papillae arranged in a V shaped pattern at the base of the tongue. They don’t have taste buds, are mechanical and are not involved in gustation. Filiform papillae are characterized by increased keratinization and are the most numerous. 3. Foliate – foliate papillae are ridges and grooves towards the posterior part of the roof of the mouth that are found on lateral margins. They are innervated by facial nerve and glossopharyngeal nerve (anterior and posterior papillae respectively). 4. Fungiform - longitudinal section looks slightly mushroom-shaped and they are mostly found at the tip and on the sides of the tongue. They are innervated by facial nerve. Sensory Transduction Taste sensation can be induced by many diverse taste solutes. The pattern of membrane potential change include depolarization, depolarization followed by hyperpolarization, or only hyperpolarization. Action potentials in the taste receptor cells lead to an increase Ca2+ influx through voltage-gated membrane channels with the release of Ca2+ from intracellular stores. In response to this cation, neurotransmitter is released, this produces synaptic potentials in the dendrites of the sensory nerves and action potentials in afferent nerve fibers. Bitterness Bitterness is the taste that detects bases. Bitterness and sweetness are sensed by G protein-coupled receptors coupled to the G protein GUSTDUCIN Bitter taste receptors are known specifically as T2R's (taste receptors, type 2). They are identified not only by their ability to taste for certain "bitter" ligands, but also by the morphology of the receptor itself (surface bound, monomeric). There are a large diversity of bitter-tasting molecules e.g. alkaloids. Alkaloids are nitrogen-containing molecules that often have a basic pH. Alkaloids are commonly found in bitter-tasting plant products, e.g. coffee, hops, tannins, tea, and aspirin. A plant is less susceptible to microbe infection and less attractive to herbivores if it contains toxic alkaloids. Consequently, the function of bitter taste may primarily be related to stimulating the gag reflex to avoid ingesting poisons. The highest concentration of bitter receptors appear to be in the posterior tongue, where a gag reflex could still spit out poisonous food. The transduction of bitter tastes involves several mechanisms: 1) Blockage of the efflux of K+ by a number of hydrophilic bitter substances creates a depolarizing potential; 2) Interaction with a receptor membrane receptor coupled to the G protein, gustducin, and activation of cAMP dependent protein kinase with blockage of K+ channels; and 3) Involves a receptor protein linked to G-protein and activation of phospholipase C, which results in substrate hydrolysis to IP3, releasing Ca2+ from intracellular stores. Saltiness Saltiness is a taste produced by the perception of sodium ions (Na+) in the saliva (and other salts though to a lesser degree). The ions of salt, especially sodium (Na+), can pass directly through ion channels in the tongue, leading to an action potential. When salt is consumed, the salt crystals dissociate into the component ions Na+ and Cl– This dissolves into the saliva in the mouth. The Na+ concentration becomes high outside the gustatory cells, creating a strong concentration gradient that drives the diffusion of the ion into the cells. The entry of Na+ into these cells results in the depolarization of the cell membrane and the generation of a receptor potential. The taste of salts is facilitated by Na+ ions which do not interact with a membrane receptor but diffuse through a Na+ channel located in the microvilli and apical membrane. Anions such as Cl- contribute to the salty taste, alternatively anions are transported into these cells by a paracellular route. The influx of these ions of salt suggests a depolarization in the apical membrane Sour This is the perception of H+ concentration. Similar to sodium ions in salts, these hydrogen ions enter the cell and trigger depolarization. Basically, sour flavors are the perception of acids in our food. Increasing hydrogen ion concentrations in the saliva (lowering saliva pH) triggers progressively stronger graded potentials in the gustatory cells. For example, orange juice—which contains citric acid—will taste sour because it has a pH value of approximately 3. The mechanism for detecting sour taste is similar to that which detects salt taste. 1. Hydrogen ion channels detect the concentration of hydronium ions (H3O+ ions) that have dissociated from an acid. Hydrogen ions are capable of permeating the amiloride-sensitive sodium channels, but this is not the only mechanism involved in detecting the quality of sourness. 2. Hydrogen ions also inhibit the potassium channel, which normally functions to hyperpolarize the cell. Thus, by a combination of direct intake of hydrogen ions (which itself depolarizes the cell) and the inhibition of the hyperpolarizing channel, sourness causes the taste cell to act in this specific manner. The hydrogen proton of acids and sour foods can influx through the Na+ channels, or through a proton transport membrane protein Some acids block the efflux of K+ at the microvilli. The resulting influx of protons or a reduction in K+ conductance will initiate receptor potentials in response to the quality of sour tastes. Sweetness Sweetness is produced by the presence of sugars, some proteins and a few other substances. Sweetness is often associated to aldehydes and ketones which contain carbonyl group. Sweetness is detected by a variety of G protein coupled receptors coupled to the G protein GUSTDUCIN found on the taste buds. At least two different variants of the "sweetness receptors" need to be activated for the brain to register sweetness. The compounds which the brain senses as sweet are consequently compounds that can bind with varying bond strength to several different sweetness receptors. The differences between the different sweetness receptors is mainly in the binding site of the G protein coupled receptors. Sweet tasting solutes, sugars and related substances, bind to membrane receptor proteins which are coupled to a G- protein (gustducin), which activates adenylyl cyclase (AC). Cyclic AMP (cAMP) dependent protein kinase (PKA) reduces K+ efflux in the apical membrane and produces membrane depolarization. Some sweet solutes and non-sugar sweeteners interact with a receptor membrane protein through a G protein, which activates phospholipase C. A second messenger, inositol triphosphate (IP3), is synthesized which releases Ca2+ from intracellular stores. Accumulation of Ca2+ depolarizes the cell, releasing neurotransmitter at the synapse. Umami This is a Japanese word meaning "savory" or "meaty" specifically, to the detection of glutamates Glutamates are commonly found in fermented and aged foods, meats, cheese e.g. parmesan and roquefort cheese, other protein-heavy foods as well as soy sauce and fish sauce. It is also found in significant amounts in some unfermented foods e.g. walnuts, grapes, broccoli, tomatoes, and mushrooms The action of umami receptors explains why foods treated with monosodium glutamate often taste fuller or just better. The receptors responsible for umami sensation is a modified form of mGluR4, in which the end of the molecule is missing. The researchers named it 'taste-mGluR4'. Like sweet and bitter, it is based on the activation of G protein–coupled receptors by a specific molecule. The molecule that activates this receptor is the amino acid L-glutamate. Umami is also provided by the nucleotides disodium 5’-inosine monophosphate (IMP) and disodium 5’-guanosine monophosphate (GMP). IMP and GMP are naturally present in many protein-rich foods. There is a synergistic effect between monosodium glutamate (MSG), IMP and GMP which together in certain ratios produce a strong umami taste. A subset of savoury taste buds responds specifically to glutamate in the same way that sweet ones respond to sugar. Glutamate binds to a variant of G protein coupled glutamate receptors. Umami, is predicted to interact with a ligand-gated inotropic glutamate receptor coupled to gustducin and to Ca2+ channel membrane proteins five sensations, each associated with a critical body function: Sweet nutritious food Sour H+ Salty Na+ Umami (savory) nutritious food Bitter toxins Step Bitter Sweet Umami Sour Salty 1 Receptor activation Activation of the G-protein gustducin entry of H+ or Na+ through 2 (there are ~24 other G-proteins that have leak channels been identified) Sour: also closes K+ channels 3 Multiple second messenger pathways Depolarization Opening of voltage-gated Ca2+ channels and/or release of Ca2+ from 4 intracellular stores Neurotransmitter release ATP for bitter, sweet, umami 5 Neurotransmitter release serotonin (5HT) for sour and salty Disorders of gustation 1. Ageusia – this is the complete absence of sensation 2. Hypogeusia – whereby there is reduced sensation 3. Dysgeusia - Distortion or perversion (i.e. perception when not present) 4. Cacogeusia - Extremely unpleasant perception (revolting) Olfaction This is a specialized sensory receptor cell which responds to an endogenous or induced chemical substance and generates a biological signal i.e. chemoreception that forms the sense of smell. As a chemical sensor, the olfactory system detects hazards e.g., spoiled food and chemical dangers, food, provides both sensual pleasure (the odor of flowers and perfume) and pheremones (influences social and sexual behavior). The cellular and molecular machinery for olfactory transduction is located in the olfactory cilia. Olfaction begins when odorants carried by inhaled air bind to specific sites on olfactory receptors located in the roof of the two nasal cavities of the human nose, just below and between the eyes. To provide sensory properties, the odorant must possess certain molecular properties e.g. 1. It must have some water solubility 2. Possess adequate high vapor pressure 3. Low polarity 4. Some lipophilicity (ability to dissolve in fat), and 5. Surface activity. Odorant molecules bind to a receptor protein (R). Olfactants are chaperoned to the receptor by olfactory binding proteins coupled to an olfactory specific G-protein (Golf). Golf activates a type III adenylyl cyclase (AC), increasing the concentration of intracellular cAMP levels. cAMP targets an olfactory-specific cyclic-nucleotide gated ion channel (CNG), allowing cations, particularly Na and Ca, to flow down their electrochemical gradients into the cell. This increase in cAMP cause the opening of non-specific ligand-gated cation channels, which leads to depolarization of the olfactory receptor neurons The Ca entering the cell is able to activate a Ca-activated Cl channel, which would allow Cl to flow out of the cell, thereby further increasing the depolarization. Elevated intracellular Ca causes adaptation by at least two different molecular steps: 1. Inhibition of the activity of adenylyl cyclase through CAMKII-dependent phosphorylation and 2. Down-regulation of the affinity of the CNG channel to cAMP. This depolarization, amplified by a Ca2+-activated Cl- current, is conducted passively from the cilia to the axon hillock region of the olfactory receptor neuron, where action potentials are generated and transmitted to the olfactory bulb. Olfactory transduction consists of 4 steps 1. Activation of the receptor protein (R) Olfactants are chaperoned to the receptor by olfactory binding proteins 2. The activated receptor stimulates a G-protein, Golf 3. Golf activates an effector protein, adenylate cyclase III, that increases cAMP concentrations 4. Increases in cAMP cause the opening of non-specific ligand-gated cation channels, which leads to depolarization Olfactory epithelia The primary olfactory epithelium is located at the top of the nasal cavity Odourants are dissolved in the mucus layer and are bound to olfactory binding proteins that chaperone the passage of the odourants to the cilia-like projections of the olfactory receptor neurons (ORNs) The olfactory receptor membrane is found on the primary afferent neurons unlike other special sense receptors. Olfactory receptor cells are the only neurons that are regularly replaced throughout life i.e. through differentiation of supporting basal cells. The olfactory pathway is the only sensory pathway that does not relay in the thalamus ORN axons enter the CNS through the cribriform plate to synapse in the olfactory bulb The direct connections to the limbic system explain why odours are usually the most powerful sensory stimuli for arousing memories Disorders of olfaction Anosmia – this is the inability to smell Dysosmia – things smell different from memory or expectation Hyperosmia – this is an abnormally acute sense of smell Hyposmia – whereby there is decreased ability to smell Olfactory reference syndrome – is a psychological disorder that causes the patient to imagine he or she has strong body odor Parosmia – a situation where things smell worse than they should Phantosmia – this is "hallucinated smell", often unpleasant in nature Muscle Physiology Muscle Tissue Skeletal Muscle Cardiac Muscle Smooth Muscle Cardiac Muscle Branching cells One/two nuclei per cell Striated Involuntary Medium speed contractions Smooth Muscle Fusiform cells One nucleus per cell Nonstriated Involuntary Slow, wave-like contractions Skeletal Muscle Long cylindrical cells Many nuclei per cell Striated Voluntary Rapid contractions Skeletal Muscle Produce movement Maintain posture & body position Support Soft Tissues Guard entrance / exits Maintain body temperature Store nutrient reserves Skeletal Muscle Structure Skeletal Muscle Fiber Sarcomere Z line Z line Sarcomere Relaxed Sarcomere Partially Contracted Sarcomere Completely Contracted Neuromuscular Junction Single Fiber Tension The all–or–none principle Length–tension relationship As a whole, a muscle fiber is -Number of pivoting cross- either contracted or relaxed bridges depends on: Tension of a Single Muscle amount of overlap between Fiber thick and thin fibers Depends on -Optimum overlap produces The number of pivoting cross- greatest amount of tension: bridges The fiber’s resting length at too much or too little reduces the time of stimulation efficiency The frequency of stimulation -Normal resting sarcomere length: is 75% to 130% of optimal length Muscle Contraction Types Isotonic contraction Isometric contraction Muscle Contraction Types Isotonic contraction Isometric contraction Muscle Contraction Types Isotonic contraction Isometric contraction ATP as Energy Source Creatine Molecule capable of storing ATP energy Creatine + ATP Creatine phosphate + ADP ADP + Creatine phosphate ATP + Creatine Metabolism Aerobic metabolism – 95% of cell demand – Kreb’s cycle – 1 pyruvic acid molecule ➔ 17 ATP Anaerobic metabolism – Glycolysis ➔ 2 pyruvic acids + 2 ATP – Provides substrates for aerobic metabolism – As pyruvic acid builds converted to lactic acid Muscle Fatigue Muscle Fatigue – When muscles can no longer perform a required activity, they are fatigued Results of Muscle Fatigue – Depletion of metabolic reserves – Damage to sarcolemma and sarcoplasmic reticulum – Low pH (lactic acid) – Muscle exhaustion and pain Muscle Hypertrophy Muscle growth from heavy training Increases diameter of muscle fibers Increases number of myofibrils Increases mitochondria, glycogen reserves Muscle Atrophy – Lack of muscle activity Reduces muscle size, tone, and power Steroid Hormones Stimulate muscle growth and hypertrophy – Growth hormone – Testosterone – Thyroid hormones – Epinephrine Muscle Tonus Tightness of a muscle Some fibers always contracted Tetany Sustained contraction of a muscle Result of a rapid succession of nerve impulses Tetanus Refractory Period Brief period of time in which muscle cells will not respond to a stimulus Refractory Refractory Periods Skeletal Muscle Cardiac Muscle THE NERVE IMPULSE © 2016 Paul Billiet ODWS Cells and membrane potentials All animal cells generate a small voltage across their membranes There are a large amount of small organic molecules in the cytoplasm (e.g. amino acids) To balance this, animal cells pump Na+ out of the cells This regulates osmosis but it leaves a large number of organic molecules These organic molecules are overall negatively charged (anions) in the cytoplasm Thus the cell has a potential difference (voltage) across its membrane. © 2016 Paul Billiet ODWS Experiments on the neuron of a giant squid Concentration /mmol kg-1 water Ion Axoplasm Blood Sea water (the cytoplasm plasma in an axon) K+ 400 20 10 Na+ 50 440 460 Cl- 120 560 540 Organic anions 360 - - (-ve ions) © 2016 Paul Billiet ODWS The neuron www.biologymad.com/.../nervoussystemintro.htm © 2016 Paul Billiet ODWS www.lab.anhb.uwa.edu.au/.../Nervous/Nervous.htm The neuron Nodes of Ranvier Dendrites Schwann cell Nucleus of Schwann cell Myelin sheath Axon Terminal dendrites Cell body © 2016 Paul Billiet ODWS Neurons Neurons, like other cells, are more negatively charged inside than outside This results in a membrane potential of about – 70 milliVolts This is called the resting potential of the neuron. © 2016 Paul Billiet ODWS Potassium & Sodium Ions The two important ions: K+ and Na+ Both are positively charged ions Na+ ions move more slowly across the membrane than K+ or Cl- ions The Na+ ion is smaller than the K+ ion (Na+ has a larger coating of water molecules giving it a bigger diameter) This makes the plasma membrane 25 times more permeable to K+ than Na+. © 2016 Paul Billiet ODWS Potassium & Sodium Ions K+ ions leak out a little from K+ ion pores cell is negative inside pulling K+ in but there is a very high concentration of K+ inside pulling K+ out K+ has to be actively pumped inwards a bit The resting potential of the neuron is almost at the equilibrium for K+ ions K+ leak out a bit and need pumping in Na+ ions, however, are actively pumped out and kept out. © 2016 Paul Billiet ODWS A coupled Na+-K+ pump plasma Cytoplasm membrane ECF K+ K+ coupled ion pump Na+ Na+ © 2016 Paul Billiet ODWS Getting excited! The neuron’s membrane at rest is more negative inside than outside The neuron is said to be polarised Neurons are excitable cells Neurons are excited when their membranes become depolarised. © 2016 Paul Billiet ODWS Depolarisation Depolarising membranes may be achieved by: a stimulus arriving at a receptor cell (e.g. vibration of a hair cell in the ear) a chemical fitting into a receptor site (e.g. a neurotransmitter) a nerve impulse travelling down a neuron. © 2016 Paul Billiet ODWS Nerve impulses Nerve impulses are self-propagating like a trail of gunpowder Localised currents in the ions occur just ahead of the impulse causing localised depolarisation Nerve impulses are not like electrical signals travelling down a wire. © 2016 Paul Billiet ODWS The action potential The action potential is the state of the neuron membrane when a nerve impulse passes by. © 2016 Paul Billiet ODWS The action potential Localised currents cause Na+ channels to flip open Voltage-gated Na+ channels As Na+ moves into the cell, more and more Na+ channels open A small change in the membrane permeability to Na+ results in a big change in membrane potential The volume of the axon is minute compared to the volume of the extracellular fluid. © 2016 Paul Billiet ODWS +35 0 More Na+ channels open mV Na+ floods into neuron Na+ voltage- gated channels open -55 Threshold -70 Time Resting potential Action potential © 2016 Paul Billiet ODWS All-or-nothing Na+s move in, the cell it will become more positive Ion pumps resist the change in the membrane potential If it rises by 15mV and the pumps cannot restore the equilibrium Na+ floods in and neuron is depolarised Nerve impulses all look the same, there are not big ones and little ones This is the all-or-nothing law. © 2016 Paul Billiet ODWS The threshold –55mV represents the threshold potential Beyond this we get a full action poten