V07 Surface Tension Lecture Notes WS 23 PDF
Document Details
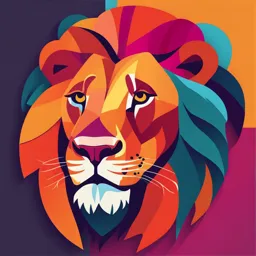
Uploaded by DauntlessLotus
RWTH Aachen University
Uwe Schnakenberg
Tags
Summary
These lecture notes cover surface tension in microfluidics, specifically related to bio-MEMS. The document discusses topics including contact angles, introduction to surface tension, manipulation of surface tension (passive and active), and terminology. Various illustrations and equations are included.
Full Transcript
V07 V 07 Surface Tension Lecture „Microfluidic Systems - Bio-MEMS“ – Surface Tension Prof. Dr.-Ing. Uwe Schnakenberg | Institute of Materials in Electrical Engineering 1 | WS 23 1 Contents and Learning Targets V07 Contents and Learning Targets 7.1 Introduction 7.2 Contact angle 7.2.1 Young‘s Equatio...
V07 V 07 Surface Tension Lecture „Microfluidic Systems - Bio-MEMS“ – Surface Tension Prof. Dr.-Ing. Uwe Schnakenberg | Institute of Materials in Electrical Engineering 1 | WS 23 1 Contents and Learning Targets V07 Contents and Learning Targets 7.1 Introduction 7.2 Contact angle 7.2.1 Young‘s Equation 7.2.2 Dynamic Contact Angle 7.3 Manipulation of Surface Tension 7.3.1 Passive: Surface Conditioning 7.3.2 Active: Electrowetting Lecture „Microfluidic Systems - Bio-MEMS“ – Surface Tension Prof. Dr.-Ing. Uwe Schnakenberg | Institute of Materials in Electrical Engineering 1 | WS 23 2 V07 7.1 Introduction Lecture „Microfluidic Systems - Bio-MEMS“ – Surface Tension Prof. Dr.-Ing. Uwe Schnakenberg | Institute of Materials in Electrical Engineering 1 | WS 23 3 7.1 Introduction V07 4 Surface Tension is Dominant in Small Dimensions Droplets formation en.wikipedia.org Filling of small capillaries Staying of insects on liquid surfaces Membrane properties Lead for electronic components …. hyperphysics.phy-astr.gsu.edu computerbild.de Lecture „Microfluidic Systems - Bio-MEMS“ – Surface Tension Prof. Dr.-Ing. Uwe Schnakenberg | Institute of Materials in Electrical Engineering 1 | WS 23 gore-tex.de en.wikipedia.org 7.1 Introduction V07 5 Surface Tension Property of fluids to reduce their surface area to minimum Results from the greater attraction of liquid molecules of the bulk to each other (due to cohesion) than to the molecules of the other phase These inward force on the surface molecules caused the liquid to contract Tangential force per unit length parallel to the surface is referred to as surface tension hyperphysics.phy-astr.gsu.edu https://en.wikipedia.org/wiki/Surface_tension Lecture „Microfluidic Systems - Bio-MEMS“ – Surface Tension Prof. Dr.-Ing. Uwe Schnakenberg | Institute of Materials in Electrical Engineering 1 | WS 23 www.wikipedia.com 7.1 Introduction V07 6 Surface Tension σ Often also defined as „γ“ Surface (tensile) force F Tangential force F per wetted perimeter l* that tends F to minimize the surface area of an interface F N , l m https://en.wikipedia.org/wiki/Surface_tension Surface energy Work W for increasing the surface divided by the area A which is generated W A J , 2 m Lecture „Microfluidic Systems - Bio-MEMS“ – Surface Tension Prof. Dr.-Ing. Uwe Schnakenberg | Institute of Materials in Electrical Engineering 1 | WS 23 * For a cylindrical capillary l = 2π·r, with r the capillary radius, see V16 7.1 Introduction V07 Terminology Surface tension Between a liquid and a gas Interface tension Between two immiscible liquids (e.g. oil and water) or liquid and a solid surface Lecture „Microfluidic Systems - Bio-MEMS“ – Surface Tension Prof. Dr.-Ing. Uwe Schnakenberg | Institute of Materials in Electrical Engineering 1 | WS 23 7 7.1 Introduction V07 8 Why can an Insect Stay on Water? Bond number Bo* Characteristic number Bo Gravitational force Fg Surfacetension Fst m g lc Wilfrid Noel Bond (1897 – 1937) When object is heavy, gravity wins and object sinks m… g …. σ …. lc..... Mass Gravitational constant Surface tension Characteristic length Bad news: Surface tension holds the object in place so it can hardly move https://en.wikipedia.org/ Lecture „Microfluidic Systems - Bio-MEMS“ – Surface Tension Prof. Dr.-Ing. Uwe Schnakenberg | Institute of Materials in Electrical Engineering 1 | WS 23 * Also called Eötvös number Eo 7.1 Introduction V07 Surface Tensions Fluid Acetone Ethanol Glycerin N-Hexane Iso-Propylalcohol Methanol Water Water Water Water Temperature [°C] 20 20 20 20 20 20 0 25 50 100 Lecture „Microfluidic Systems - Bio-MEMS“ – Surface Tension Prof. Dr.-Ing. Uwe Schnakenberg | Institute of Materials in Electrical Engineering 1 | WS 23 Surface Tension [mN/m] 23.70 22.27 63.00 18.40 21.70 22.60 75.64 71.97 67.91 58.85 9 7.1 Introduction de.wikipedia.com V07 10 Surface Tension Depends on Temperature Eötvös‘ Rule Loránd Eötvös (1848 – 1919) Surface tension σ of a pure fluid depends k 2 Vm 3 (Tc 6 K T ) Vm … Molar volume Tc …. Critical temperature (unit in [K]) at which σ = 0 k ….. 2.1*10-7 J/K*mol-2/3 for all fluids Lecture „Microfluidic Systems - Bio-MEMS“ – Surface Tension Prof. Dr.-Ing. Uwe Schnakenberg | Institute of Materials in Electrical Engineering 1 | WS 23 Benzene Temperature / [K] https://de.wikipedia.org/ Surface tension / [mN/m] linearly on temperature V07 7.2 Contact Angle Lecture „Microfluidic Systems - Bio-MEMS“ – Surface Tension Prof. Dr.-Ing. Uwe Schnakenberg | Institute of Materials in Electrical Engineering 1 | WS 23 11 7.2 Contact Angle V07 12 Contact Angle Θc Is the angle, conventionally measured through the liquid, where a liquid-vapor interface meets a solid surface (triple point) A given system of solid, liquid and vapor has an unique equilibrium contact angle at a given temperature and pressure γSG.. solid-gas interfacial tension γSL … solid-liquid interfacial tension γLG... liquid-gas surface tension http://de.wikipedia.org/wiki/Kontaktwinkel Lecture „Microfluidic Systems - Bio-MEMS“ – Surface Tension Prof. Dr.-Ing. Uwe Schnakenberg | Institute of Materials in Electrical Engineering 1 | WS 23 7.2 Contact Angle V07 13 hydrophilic hydrophobic Θ < 90° Θ > 90° www.rheinfaktor.de Lecture „Microfluidic Systems - Bio-MEMS“ – Surface Tension Prof. Dr.-Ing. Uwe Schnakenberg | Institute of Materials in Electrical Engineering 1 | WS 23 7.2 Contact Angle V07 14 C 90 C 90 chem1.com Lecture „Microfluidic Systems - Bio-MEMS“ – Surface Tension Prof. Dr.-Ing. Uwe Schnakenberg | Institute of Materials in Electrical Engineering 1 | WS 23 V07 15 7.2.1 Young’s Equation Lecture „Microfluidic Systems - Bio-MEMS“ – Surface Tension Prof. Dr.-Ing. Uwe Schnakenberg | Institute of Materials in Electrical Engineering 1 | WS 23 7.2.1 Young’s equation Equilibrium of forces SG SL cos C LG Young‘s equation de.wikipedia.org at triple point V07 16 Thomas Young (1773 – 1829) http://archiv.aktuelle-wochenschau.de/2011/w12/woche12.html T. Young: Phil. Trans. R. Soc. London 95 65-87 (1805) Lecture „Microfluidic Systems - Bio-MEMS“ – Surface Tension Prof. Dr.-Ing. Uwe Schnakenberg | Institute of Materials in Electrical Engineering 1 | WS 23 V07 17 7.2.2 Dynamic Contact Angle Lecture „Microfluidic Systems - Bio-MEMS“ – Surface Tension Prof. Dr.-Ing. Uwe Schnakenberg | Institute of Materials in Electrical Engineering 1 | WS 23 V07 18 Dynamic Contact Angle For liquid moving over a surface, the contact angle is altered from its value at rest https://www.cscscientific.com 7.2.2 Dynamic Contact Angle https://perminc.com/ Droplets try to withstand the movement, because of adhesion forces to the bottom plate Advancing contact angle θa increases Receding contact angle θr decreases During droplet movement, θa and θr depend on Droplet speed Viscosity and surface tension of droplet Hydrophobicity of substrate Lecture „Microfluidic Systems - Bio-MEMS“ – Surface Tension Prof. Dr.-Ing. Uwe Schnakenberg | Institute of Materials in Electrical Engineering 1 | WS 23 θs …Static contact angle θa … Advancing contact angle θr … Receding contact angle 7.2.2 Dynamic Contact Angle V07 19 Hysteresis of Dynamic Contact Angle https://www.youtube.com/watch?v=Qxe2gp3e1u8 Y. Wei, D. Beck: doi: 10.1117/2.1200705.0758 Lecture „Microfluidic Systems - Bio-MEMS“ – Surface Tension Prof. Dr.-Ing. Uwe Schnakenberg | Institute of Materials in Electrical Engineering 1 | WS 23 V07 20 7.3 Manipulation of Surface Tension Lecture „Microfluidic Systems - Bio-MEMS“ – Surface Tension Prof. Dr.-Ing. Uwe Schnakenberg | Institute of Materials in Electrical Engineering 1 | WS 23 7.3 Manipulation of Surface (Interface) Tension V07 21 Manipulation of Surface (Interface) Tension Passive Surfaces Hydrophobization Active Surfaces Electrically (electrowetting) Hydrophilization Fluid Wetting agents Lecture „Microfluidic Systems - Bio-MEMS“ – Surface Tension Prof. Dr.-Ing. Uwe Schnakenberg | Institute of Materials in Electrical Engineering 1 | WS 23 V07 22 7.3.1 Passive Manipulation of Surface Tension: Surface Conditioning Lecture „Microfluidic Systems - Bio-MEMS“ – Surface Tension Prof. Dr.-Ing. Uwe Schnakenberg | Institute of Materials in Electrical Engineering 1 | WS 23 7.3.1 Passive Manipulation of Surface Tension: Surface Conditioning Polar Molecules Molecules with, e.g., -OH, -COOH, or -NH2 groups are water-soluble Make hydrogen bonds with water - hydrophilic molecules Cysteamine Non-polar Molecules Molecules with, e.g., -CH3 or lipids, are not water-soluble Do not make hydrogen bonds with water - hydrophobic molecules Methyl group Lecture „Microfluidic Systems - Bio-MEMS“ – Surface Tension Prof. Dr.-Ing. Uwe Schnakenberg | Institute of Materials in Electrical Engineering 1 | WS 23 V07 23 7.3.1 Passive Manipulation of Surface Tension: Surface Conditioning Hydrophobization V07 24 To prevent wetting with water Generation of non-polar groups at surfaces Covering the surface with self-assembling monolayer (SAM) Long-chain CH- oder FC-molecules High contact angle ( > 100°) Methyl 3-mercaptopropionate www2.mic.dtu.dk Lecture „Microfluidic Systems - Bio-MEMS“ – Surface Tension Prof. Dr.-Ing. Uwe Schnakenberg | Institute of Materials in Electrical Engineering 1 | WS 23 7.3.1 Passive Manipulation of Surface Tension: Surface Conditioning Hydrophilization V07 25 Improvement of wetting with water Generation of polar groups at surfaces Mercaptoethanol Coverage with self-assembling monolayer (SAM) On metals, metal oxides, 11-Mercaptoundecanoic acid oxides, hydroxides Small contact angle (ΘC < 80°) Polyethylenglycol Lecture „Microfluidic Systems - Bio-MEMS“ – Surface Tension Prof. Dr.-Ing. Uwe Schnakenberg | Institute of Materials in Electrical Engineering 1 | WS 23 7.3.1 Passive Manipulation of Surface Tension: Surface Conditioning Manipulation of Wetting with Surfactants in Fluids SURFACTANT (SURFace ACTing AgeNT) Surfactants Molecules have hydrophilic group at one end hydrophobic group at the other end Hydrophilic group in water phase Hydrophobic group in oil-based phase Form a thin interface layer between the two phases Decrease of surface tension → Interface is getting more stretchable No change of fluid viscosity or density Lecture „Microfluidic Systems - Bio-MEMS“ – Surface Tension Prof. Dr.-Ing. Uwe Schnakenberg | Institute of Materials in Electrical Engineering 1 | WS 23 V07 26 7.3.1 Passive Manipulation of Surface Tension: Surface Conditioning https://www.youtube.com/watch?v=uuwmYltg-KY V07 27 https://www.youtube.com/watch?v=w9bXMInpwLE Lecture „Microfluidic Systems - Bio-MEMS“ – Surface Tension Prof. Dr.-Ing. Uwe Schnakenberg | Institute of Materials in Electrical Engineering 1 | WS 23 7.3.2 Active Manipulation of Surface Tension: Electrowetting V07 28 7.3.2 Active Manipulation of Surface Tension: Electrowetting (EW) Electrowetting on Dielectrics (EWOD) Lecture „Microfluidic Systems - Bio-MEMS“ – Surface Tension Prof. Dr.-Ing. Uwe Schnakenberg | Institute of Materials in Electrical Engineering 1 | WS 23 7.3.2 Active Manipulation of Surface Tension: Electrowetting V07 29 Electrowetting (EW) Electrowetting on Dielectrics (EWOD) Electrostatic modification of surface tension between droplet and hydrophobic surface Hydrophobic surface Lecture „Microfluidic Systems - Bio-MEMS“ – Surface Tension Prof. Dr.-Ing. Uwe Schnakenberg | Institute of Materials in Electrical Engineering 1 | WS 23 d www.nature.com Decrease of contact angle through an applied electric field 7.3.2 Active Manipulation of Surface Tension: Electrowetting V07 30 Electrowetting By applying a voltage U, an electric double layer builds up spontaneously at the solid-liquid interface consisting of charges on the metal electrode and a cloud of oppositely charged counter-ions on the liquid side of the interface Charge leads to a reduction of the effective interfacial tension (like surfactants) The fringing field at the corners of the droplet tends to pull the droplet down onto the electrode, lowering the macroscopic contact angle and increasing the droplet contact area F. Krogmann et al: JMEMS 17 (6) 1501-1512 (2008) Lecture „Microfluidic Systems - Bio-MEMS“ – Surface Tension Prof. Dr.-Ing. Uwe Schnakenberg | Institute of Materials in Electrical Engineering 1 | WS 23 7.3.2 Active Manipulation of Surface Tension: Electrowetting V07 31 Electrowetting Contact angle is determined by surface energy Energy which is stored in the capacitor, the application of a voltage decreases the surface energy increases wettability Contact angle is a function of applied voltage Hydrophobic surface is necessary because contact angle can only decrease 0.1-1 mm d Hydrophobic surface Isolation layer Electrode F. Mugele: Soft Matter 5 3377-3384 (2009) Lecture „Microfluidic Systems - Bio-MEMS“ – Surface Tension Prof. Dr.-Ing. Uwe Schnakenberg | Institute of Materials in Electrical Engineering 1 | WS 23 www. uni-ulm.de 7.3.2 Active Manipulation of Surface Tension: Electrowetting SG SL LG Young‘s equation www.aip.org cos C V07 32 By application of a voltage V r 0 V2 cos cos C LG d 2 Young-Lippmann equation (1875) d …. Thickness of isolator Lecture „Microfluidic Systems - Bio-MEMS“ – Surface Tension Prof. Dr.-Ing. Uwe Schnakenberg | Institute of Materials in Electrical Engineering 1 | WS 23 Gabriel Lippmann (1845 – 1921) 7.3.2 Active Manipulation of Surface Tension: Electrowetting V07 33 Young-Lippmann Equation Curve progression symmetric to y-axis (→ V2-dependency) www.physicaplus.org.il r 0 V 2 cos cos 0 lg d 2 Electrowetting number Describes the relationship between electrostatic force and surface tension σlg can be determined with these measurements Lecture „Microfluidic Systems - Bio-MEMS“ – Surface Tension Prof. Dr.-Ing. Uwe Schnakenberg | Institute of Materials in Electrical Engineering 1 | WS 23 7.3.2 Active Manipulation of Surface Tension: Electrowetting V07 34 Several Explanations of Contact Angle Saturation Several rather disconnected hypotheses have been published Charge trapping in the insulation/dielectric layer H.J.J. Verheijen et al.: https://doi.org/10.1021/la990548n Gas ionization in the vicinity of the contact line and contact line instability M. Vallet et al.: https://doi.org/10.1007/s100510051186 Droplet resistance is shape dependent and therefore dependent on contact angle B. Shapiro et al.: https://doi.org/10.1063/1.1563828 Zero interfacial tension criterion V. Peykov et al.: https://doi.org/10.1007/s003960000333 A. Quinn et al.: https://doi.org/10.1021/jp040478 Lecture „Microfluidic Systems - Bio-MEMS“ – Surface Tension Prof. Dr.-Ing. Uwe Schnakenberg | Institute of Materials in Electrical Engineering 1 | WS 23 See annex 7.3.2 Active Manipulation of Surface Tension: Electrowetting SG SL cos C LG V07 35 Young‘s equation The contact angle dependence on the applied voltage is entirely ascribed to changes in the solid/liquid interface tension γSL The other two tensions are assumed to be independent on applied voltage γSL decreased when voltage is increased until it becomes zero, the lowest possible value A. Quinn et al.: J. Phys. Chem. B 109 6268-6275 (2005) Lecture „Microfluidic Systems - Bio-MEMS“ – Surface Tension Prof. Dr.-Ing. Uwe Schnakenberg | Institute of Materials in Electrical Engineering 1 | WS 23 7.3.2 Active Manipulation of Surface Tension: Electrowetting V07 36 Young-Lippmann Equation r 0 V 2 cos cos 0 lg d 2 700 nl droplet (ca. 1,5 mm Φ) → video on URL microfluidics.ee.duke.edu/ Lecture „Microfluidic Systems - Bio-MEMS“ – Surface Tension Prof. Dr.-Ing. Uwe Schnakenberg | Institute of Materials in Electrical Engineering 1 | WS 23 7.3.2 Active Manipulation of Surface Tension: Electrowetting Θ1 Electrode A V07 37 Θ2 Electrode B U http://www.utdallas.edu By application of a voltage to adjacent electrode, charges are induced along the surface → decrease the interfacial energy between the solid surface and the electrolyte Decrease in interfacial energy results a decrease in contact angle → Movement of droplet to the gated electrode F. Mugele: Soft Matter 5 3377-3384 (2009) Lecture „Microfluidic Systems - Bio-MEMS“ – Surface Tension Prof. Dr.-Ing. Uwe Schnakenberg | Institute of Materials in Electrical Engineering 1 | WS 23 7.3.2 Active Manipulation of Surface Tension: Electrowetting http://www.youtube.com/watch?v=fTjy39ptaR4 Lecture „Microfluidic Systems - Bio-MEMS“ – Surface Tension Prof. Dr.-Ing. Uwe Schnakenberg | Institute of Materials in Electrical Engineering 1 | WS 23 V07 38 7.3.2 Active Manipulation of Surface Tension: Electrowetting Droplet M.G. Pollack et al.: Lab Chip 2 96-101 (2002) Lecture „Microfluidic Systems - Bio-MEMS“ – Surface Tension Prof. Dr.-Ing. Uwe Schnakenberg | Institute of Materials in Electrical Engineering 1 | WS 23 V07 39 7.3.2 Active Manipulation of Surface Tension: Electrowetting → video on URL microfluidics.ee.duke.edu/ → video on URL microfluidics.ee.duke.edu/ M.G. Pollack et al.: Lab Chip 2 96-101 (2002) V07 40 6 nl droplets (~0.3 mm Φ), 200 Hz Lecture „Microfluidic Systems - Bio-MEMS“ – Surface Tension Prof. Dr.-Ing. Uwe Schnakenberg | Institute of Materials in Electrical Engineering 1 | WS 23 7.3.2 Active Manipulation of Surface Tension: Electrowetting Hysteresis Effect of Dynamic Contact Angle M.G. Pollack et al.: Lab Chip 2 96-101 (2002) Lecture „Microfluidic Systems - Bio-MEMS“ – Surface Tension Prof. Dr.-Ing. Uwe Schnakenberg | Institute of Materials in Electrical Engineering 1 | WS 23 V07 41 7.3.2 Active Manipulation of Surface Tension: Electrowetting V07 42 microfluidics.ee.duke.edu/ Droplet Separation and Merging http://www.youtube.com/watch?v=Tjp9qxob9pw S.K. Cho et al.: J. Microelectromech. Sys. 12 70-80 (2003) M.G. Pollack et al.: Lab Chip 2 96-101 (2002) P. Paik et al.: Lab Chip 3 253-259 (2003) Lecture „Microfluidic Systems - Bio-MEMS“ – Surface Tension Prof. Dr.-Ing. Uwe Schnakenberg | Institute of Materials in Electrical Engineering 1 | WS 23 → Mixing by repetition 7.3.2 Active Manipulation of Surface Tension: Electrowetting Mixing of Droplets by Shaking P. Paik et al.: Lab Chip 3 28-33 (2003) Lecture „Microfluidic Systems - Bio-MEMS“ – Surface Tension Prof. Dr.-Ing. Uwe Schnakenberg | Institute of Materials in Electrical Engineering 1 | WS 23 V07 43 7.3.2 Active Manipulation of Surface Tension: Electrowetting Different Regimes for Mixing of Droplets P. Paik et al.: Lab Chip 3 253-259 (2003) Lecture „Microfluidic Systems - Bio-MEMS“ – Surface Tension Prof. Dr.-Ing. Uwe Schnakenberg | Institute of Materials in Electrical Engineering 1 | WS 23 V07 44 7.3.2 Active Manipulation of Surface Tension: Electrowetting at 16 Hz P. Paik et al.: Lab Chip 3 253-259 (2003) J. Fowler et al: IEEE 15th Int. Conf. MEMS, Las Vegas, NV, January 20-24, 2002, pp 97-100 Lecture „Microfluidic Systems - Bio-MEMS“ – Surface Tension Prof. Dr.-Ing. Uwe Schnakenberg | Institute of Materials in Electrical Engineering 1 | WS 23 V07 45 PortaDrop Digital microfluidic platform Jan Wagner - December 8, 2023 V07 47 Contents Introduction and Motivation Technology EWOD Platform Experiments Challenges Development Goals 47 PortaDrop – Digital microfluidic platform Jan Wagner | IWE1 | [email protected] 24.05.2023 V07 48 Introduction and Motivation Project: “Catalytic Microgel Reactions at Interface of Immiscible Droplets” Collaboration with DWI (Prof. Pich) Microgel synthesis and characterization Microgels 48 Soft, colliodal polymer networks Topology and properties tuneable during synthesis Possible functionalization @ Phase boundary (w/o): concentration at interface PortaDrop – Digital microfluidic platform Jan Wagner | IWE1 | [email protected] 24.05.2023 Oliver Fiukowski, DWI V07 49 Introduction and Motivation Catalytically active microgel systems Organic synthesis: reactions at w/o interfaces Functionalized microgels as catalysts Influences on catalytic performance? Size? Concentration? Softness? Solvent? H2O2 Localization of catalytic units (core/shell)? → Systematic analysis necessary → Use of digital microfluidics 49 PortaDrop – Digital microfluidic platform Jan Wagner | IWE1 | [email protected] 24.05.2023 H2O Oliver Fiukowski, DWI V07 50 Introduction and Motivation Why to use a DMF system? Alternatives Bulk reactions in dispersions Channel-based microfluidics DMF platform has unique advantages Very small amounts of analytes (nl) needed Precise control of reaction times and interface area → qualitative analysis of reaction kinetics Separation of droplets without residues Reuse of catalyst possible → Systematic analysis of reaction kinetics at single water/oil droplet interfaces 50 PortaDrop – Digital microfluidic platform Jan Wagner | IWE1 | [email protected] 24.05.2023 V07 51 Technology - Devices Device architecture - closed configuration glass counter electrode (ITO) PTFE dielectric glass Bottom Chip Cr/Au path electrodes Dielectric layer Hydrophobic coating Top Chip Transparent counter electrode (ITO) Hydrophobic coating 51 PortaDrop – Digital microfluidic platform Jan Wagner | IWE1 | [email protected] 24.05.2023 path electrodes (Cr/Au) Top chip Parafilm spacer Bottom chip Technology - Electronics Working principle Application of voltage between path electrodes and counter electrode Parameters: 52 DC or AC voltage Amplitude Frequency Activation time PortaDrop – Digital microfluidic platform Jan Wagner | IWE1 | [email protected] 24.05.2023 V07 52 EWOD platform Design and requirements 53 droplet manipulation (electrowetting/dielectrowetting) HV generation: adjustable, up to 250 V AC frequencies up to ~50 kHz addressing of 59 path electrodes operation from single 5 V supply USB/UART interface for control of experiments PortaDrop – Digital microfluidic platform Jan Wagner | IWE1 | [email protected] 24.05.2023 V07 53 V07 54 New EWOD Platform Working principle Based on integrated low voltage serial to high-voltage parallel converter (HV507, Microchip) Up to 300 V 64 channels Push-pull outputs AC signals: switching of counter electrode with inverted signal 54 PortaDrop – Digital microfluidic platform Jan Wagner | IWE1 | [email protected] 24.05.2023 VDC = 200 V f = 100 Hz V07 55 Digital microfluidic platform Hardware Control software experiments controlled from a PC software with graphical user interface “recipe” files to define experiment sequences parameters/protocols can be changed easily VDC = 200 V f = 100 Hz UDC,in = 250 V f = 10 kHz 55 PortaDrop – Digital microfluidic platform Jan Wagner | IWE1 | [email protected] 24.05.2023 V07 56 Experiments – Concept Aqueous phase: microgel solutions Not EWOD-moveable (microgel adsorption on PTFE) Organic phase: 1-octanol Low vapor pressure Low water solubility Sufficient conductivity → Suitable organic solvent for EWOD Idea Microgel solutions remain stationary on chip All droplet operations carried out on octanol droplet Required droplet operations Dispensing Moving Droplet splitting 56 PortaDrop – Digital microfluidic platform Jan Wagner | IWE1 | [email protected] 24.05.2023 Merging Phase separation V07 57 Droplet movement DI-water droplets can be moved at high velocities Movement at up to 191 mm/s without splitting Maximum velocity as a measure of force on droplets Optimal AC frequency liquid-dependent 1-octanol can be also dispensed and moved Droplet movement (1-octanol) Droplet movement (DI-water, 53,6 mm/s) 57 PortaDrop – Digital microfluidic platform Jan Wagner | IWE1 | [email protected] 24.05.2023 Droplet movement (DI-water, 191 mm/s) Droplet dispensing Aim: precise volumes for chemical reactions Repeatability Dependence on reservoir volume Comparison of reservoir designs Conventional design L-junction 58 PortaDrop – Digital microfluidic platform Jan Wagner | IWE1 | [email protected] 24.05.2023 V07 58 Droplet dispensing – 1-octanol Parameters Voltage and frequency Reservoir design Dispensing time Liquid volume in reservoir Results Conventional design: 𝜎𝑣 ≈ 1.51 % L-Junction: 𝜎𝑣 ≈ 0.37 % 59 PortaDrop – Digital microfluidic platform Jan Wagner | IWE1 | [email protected] 24.05.2023 V07 59 V07 60 Droplet merging and separation Aim: Defined contact line Required for determination of interface area No overlapping or emulsion formation On Teflon surface: well defined, almost vertical interface Separation of droplets is possible Challenge: splitting does not occur at liquid-liquid interface 60 PortaDrop – Digital microfluidic platform Jan Wagner | IWE1 | [email protected] 24.05.2023 1-octanol DI-water Droplet merging and separation Concept: Hydrophilic well Structuring of ITO + Teflon layer on top chip exposes glass surface Hydrophilic well confines aqueous droplet to defined position and shape on chip Enables merging with organic solvent droplet and “clean” phase separation 61 PortaDrop – Digital microfluidic platform Jan Wagner | IWE1 | [email protected] 24.05.2023 V07 61 V07 62 Droplet merging and separation With hydrophilic well Defined interface Shape of interface changes due to lower contact angle in the well Droplet separation Improvement using hydrophilic well Separation happens at the interface “clean” and reliable phase separation is possible 62 PortaDrop – Digital microfluidic platform Jan Wagner | IWE1 | [email protected] 24.05.2023 DI-water 1-octanol Droplet splitting Aim: Small droplet (~ 100 nl) for off-line analysis Larger droplet remains on chip for subsequent reactions Asymmetric Y-junction splitter with 3:1 ratio Droplet volume ratio: 3.61 ± 0.37% (n = 10) 63 PortaDrop – Digital microfluidic platform Jan Wagner | IWE1 | [email protected] 24.05.2023 V07 63 V07 64 Thank you for your attention Questions? Electrowetting V07 65 Properties of EWOD Systems Droplet manipulation protocol is software-driven Individual addressing of droplet (free programmable) Platform is reconfigurable Parallel processing possible No mechanical components (e.g. valves, pumps, …) No channels No resistive current, only capacitive current Very low energy consumption: nW / Transfer High droplet velocities (up to 25 cm/s possible) Mobile setups are possible High voltages (depends on dielectric layer and its thickness) 65 PortaDrop – Digital microfluidic platform Jan Wagner | IWE1 | [email protected] 24.05.2023 Conclusion V07 66 Conclusion Surface tension is one dominant effect in microfluidics Contact angle is the parameter to describe surface tension Manipulation of surface tension Passively through hydrophilic/hydrophobic surfaces Actively through electrowetting effect Reconfigurable EWOD platforms are used to enable automated droplet-based assays with nL / fL volumes Lecture „Microfluidic Systems - Bio-MEMS“ – Surface Tension Prof. Dr.-Ing. Uwe Schnakenberg | Institute of Materials in Electrical Engineering 1 | WS 23 V07 67 One Minute Paper 1. What was the most important topic you understood? 2. What was the topic you didn‘t catch? Lecture „Microfluidic Systems - Bio-MEMS“ – Surface Tension Prof. Dr.-Ing. Uwe Schnakenberg | Institute of Materials in Electrical Engineering 1 | WS 23 Annex V07 68 L1 L2 L3 Droplet resistance is shape dependent and therefore dependent on contact angle As Θ decreases, the radius of the drop increases (to keep the volume constant) In addition the liquid edges pull away from the fixed electrode This means that the ions in the liquid have to travel a longer distance to get from the bottom of the top electrode to the triple line (L1 < L2 < L3) Thus the effective resistance of the liquid increases as contact angle decreases B. Shapiro et al.: J. Appl. Phys. 93 5794 (2003) Lecture „Microfluidic Systems - Bio-MEMS“ – Surface Tension Prof. Dr.-Ing. Uwe Schnakenberg | Institute of Materials in Electrical Engineering 1 | WS 23