UV-VIS Spectroscopy PDF
Document Details
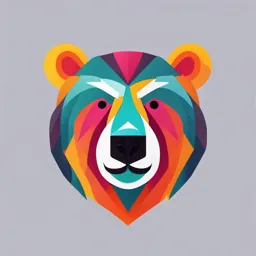
Uploaded by TrustworthyYellow5490
Tags
Summary
These notes provide an overview of UV-Vis Spectroscopy, covering its principles, mechanisms, and applications in organic chemistry. The document discusses the electronic transitions that lead to absorption and the interpretation of UV-Vis spectra. It also details how to use UV-Vis spectrometry for the analysis of organic compounds and explores Beers Law and how to calculate concentration.
Full Transcript
W I L L I A M S UV/VIS SPECTROSCOPY Definition: UV-VIS Spectroscopy is a technique that measures the absorption of ultraviolet (200–400 nm) and visible (400–800 nm) light by a sample. The absorption corresponds to electronic transitions in the mo...
W I L L I A M S UV/VIS SPECTROSCOPY Definition: UV-VIS Spectroscopy is a technique that measures the absorption of ultraviolet (200–400 nm) and visible (400–800 nm) light by a sample. The absorption corresponds to electronic transitions in the molecules, providing valuable information about their structure and composition. Importance: This technique is widely used in analytical chemistry for its high accuracy, speed, and affordability. It is essential for analyzing complex mixtures, determining W H AT I S concentrations, and characterizing chemical compounds in various fields, including pharmaceuticals, environmental UV/VIS? analysis, and biochemistry. Graph: The typical output of a UV-VIS spectrum is a graph that plots the intensity of absorbed light (or transmittance) on the y-axis against the wavelength of light on the x-axis. This spectrum provides peaks that correspond to specific wavelengths where the sample absorbs light, allowing for the identification and quantification of compounds. MECHANISM OF UV/VIS SPECTROSCOPY Absorption in UV-VIS spectroscopy occurs when photons of light are absorbed by a sample, causing electrons within the sample to transition from a lower energy state to a higher one. The energy of the absorbed light corresponds to the energy gap between the electron’s initial state and its excited state. This process is highly dependent on the molecular structure and the electronic configuration of the substance being studied. Absorption of uv/vis in organic compounds causes the electrons to transition from ground state orbitals to higher energy states. These orbitals include: σ (sigma): Found in single bonds (like between two hydrogen atoms). π (pi): Found in double or triple bonds (like in alkenes or alkynes). n (non-bonding): Found in lone pairs of electrons (like on oxygen or nitrogen atoms). Antibonding Orbitals: The higher energy states the electrons jump to are called antibonding orbitals. These are unstable compared to the original orbitals where the electrons started. TYPICAL ELECTRONIC TRANSITIONS σ → σ* transitions need a lot of energy, which is found in the vacuum UV range. This range is hard to study with regular equipment. n → σ* and n → π* transitions need less energy and occur in the near-UV range. Transitions involving π orbitals (like π → π*) are the most useful for analysis because they provide meaningful information and occur in the UV-VIS range. POSSIBLE ELECTRON TRANSITION IN M O L E C U L A R O R B I TA L S Of all the possible transitions only π → π *, n → π* and n → σ* normally produce absorption in the UV and visible region, the others require much more energy. In ethene (C=C bond), the π → π* transition refers to the excitation of an electron from a bonding π orbital to an antibonding π* orbital when it absorbs UV light. This requires high-energy light with a wavelength of around 170 nm, which falls in the vacuum UV region, making it challenging to study with standard UV-VIS spectroscopy instruments. However, in molecules with conjugated double bonds (e.g., alternating single and double bonds like in butadiene or benzene), the π and π* orbitals interact. This interaction reduces the energy difference (ΔE) between these orbitals. As a result:The energy required to promote an electron is less. The molecule absorbs light at a longer wavelength (lower energy) that falls within the near-UV or visible region, making it more accessible for analysis. Importantly, as the number of conjugated double bonds increases, the energy gap between π and π* orbitals becomes even smaller. This means:The molecule absorbs light at progressively longer wavelengths, often moving from the UV region into the visible spectrum. UV SPECTRUM OF ISOPROPENE ABSORPTION BANDS When molecules absorb light in the UV or visible region, electrons are excited from the ground state to higher electronic energy levels. Each electronic energy level is not isolated but associated with a series of vibrational and rotational energy levels. These energy sub-levels create a multitude of possible transitions.The result is not a single absorption line but a group of closely spaced lines. These lines collectively form an absorption band. These humps show than the molecule is absorbing radiation over a band of wavelengths A N A LY S I N G G R A P H S The wavelength at which the sample shows maximum absorption is denoted by λmax , this is the tallest part of the peak for an absorption band. Organic functional groups can be identified by their typical λmax values while the concentration of a solution can be determined from the amount of light absorbed by a solution.(Beer-Lamberts Law) Some organic molecules have structural features that can cause ORGANIC the absorption of uv and vis wavelengths. These structural features are called CHROMOPHORES. COMPOUND : UV/VIS The term CHROMOPHORE describes a molecular feature that is responsible for the absorption of light of any wavelength. Visible light that hits the chromophore can be absorbed by exciting an electron from its ground state into an excited state. Analysis of samples by UV-VIS spectroscopy entails accurate measurement of the wavelength of maximum absorption (λmax) and of the intensity of absorption. S O LV E N T Absorption of UV-visible radiation is determined using USE very dilute solutions, so the sample to be analyzed must be dissolved in a solvent. The solvent must be one which does not absorb light in the same wavelength as the sample. E.g 95% ethanol, water and hexane. When white light interacts with a substance which has an absorption in the region of the spectrum, a characteristic portion of the mixed wavelengths is absorbed. The remaining wavelengths are transmitted, and the substance will assume the complementary color of the wavelength/s absorbed. Complementary colors are pairs of colors that, when COLORS combined, cancel each other out (produce white or SHOWN gray in light). If a substance absorbs light of a specific color, the color we perceive is its complementary color. ABS SEEN Violet (400–450 nm) Yellow-GreenBlue (450–495 nm) YellowGreen (495–570 nm) RedYellow (570–590 nm) An ultraviolet-visible spectrophotometer or colorimeter is used to quantitatively measure the amount of light absorbed by a solution. THE SPECTROPHOTOMETER THE SPECTROPHOTOMETER LAMP, the light source is a deuterium or tungsten lamp which supplies a constant amount of light to the sample. MONOCHROMATOR selects the required wavelength (λ) to be absorbed by the sample. BEAN SPLITTER divides the light into two equally intense beams. DETECTOR compares the two beans and records it. THE U V-V I S I B L E A B S O R P T I O N SPECTROMETER The procedure to determine absorbance is: Set the wavelength of light required. Place pure solvent in the cell (water, ethanol…) Adjust the meter reading to 0 absorbance or 100% transmittance. Put the sample in another identical cell and place this in the path of the light. Record the meter reading ( A or T). Repeat at other selected wavelengths. A calibration curve using standard solutions can be used to relate the Absorbance to the concentration of the substance present. THE SPECTROPHOTOMETER Over a period of time the spectrophotometer can scan all the component wavelengths from ultraviolet region (200nm – 400 nm) to the visible region (400nm – 800nm) and plot of absorbance (A) versus wavelength (λ) is generated. This plot is called a UV-Vis or electronic absorbance spectrum. By conversion, in UV-Vis spectrometry, we use λ in nanometers (nm = 10-9 m) R E L AT I O N S H I P B E T W E E N L I G H T A B S O R P T I O N AND COLOR OBSERVED When ALL visible light is absorbed, a substance appears black, if light between 400 and 800 nm is not absorbed, the substance appears colourless. The intensity of the colour (how dark the solution appears) is directly related to the concentration of the absorbing species. This is the basis for the quantitative determination of the unknown analyte by UV-Vis spectroscopy. R E L AT I O N S H I P B E T W E E N L I G H T A B S O R P T I O N AND COLOR OBSERVED Seeing Color The sensors in our eyes detect only those wavelengths in the visible portion of the electromagnetic spectrum. When the wavelengths of one or more colors is absorbed, it is the colors on the opposite side of the color wheel that are transmitted RELATIONSHIP BETWEEN LIGHT ABSORPTION AND COLOR OBSERVED Color of light Wavelength (nm) Color Absorbed Absorbed Observe Violet 400 Yellow Blue 450 Orange Green-blue 500 Red Yellow 550 Violet Orange 600 Blue -Green Red 700 Green Factors such as solvents, substituents and conjugation will affect the position of λmax. COMMON CHROMOPHORES AND THEIR APPROXIMATE ABSORTION MAXIMA. CHROMOPHORE TYPICAL λ MAX TRANSITIONS ALKENE C=C 175 π → π* CONJUGATED ALKENE C=C-C=C 220 π → π* 180 (LARGE EMAX) ALKYNE C≡C 225 (SMALL EMAX) π → π* 185 π → π* CARBONYL C=O 280 n → π* CARBOXYL COOH 205 n → π* AMIDE CONH 215 n → π* AZO N=N 340 n → π* NITRO NO 2 280 n → π* NITRATE NO3- 270 n → π* ALCOHOL OH 180 n → σ* THE ABSORPTION OF LIGHT IN TRANSITION ELEMENT IONS When light passes through a solution containing transition metal complexes, we see those wavelengths of light that are transmitted. The solutions of most octahedral Cu (II) complexes are blue. The visible spectrum for an aqueous solution of Cu (II), [Cu(H2O6]2+, shows that the absorption band spans the red-orange-yellow portion of the spectrum and green, blue and violet are transmitted. S P E C T R A O F I N O R G A N I C ( M E TA L A N D N O N - M E TA L ) I O N S A N D I O N I C C O M P L E X E S Inorganic anions have broad UV absorption bands from non- bonding electrons. Transition metal ions and complexes absorb visible light upon excitation between filled and unfilled d-orbitals. The energy (E) required for d-d transitions is in the visible region of the spectrum. The colour of the complex is a complement of the ABSORPTIO colours absorbed from the visible light. N BY TRANSITIO DETECTION LIMIT is the lowest concentration of and N M E TA L analyte that can be detected at a known confidential COMPLEXES level.. SENSIBILTY is the ability of a method to discriminate between a blank small differences in measurements. Colourless compounds do not absorb visible light and cannot be quantified by direct measurement. In many cases the colourless sample is reacted with a colouring agent to form a highly coloured compound complex which absorbs visible light. This group is called an AUXOCHROME. D E T E R M I N AT I O N O F C O N C E N T R AT I O N U S I N G U V-V I S S P E C T R O S C O P Y First, a series of solutions of known concentrations (standard solutions) must be prepared. The absorbances of these solutions can be measured at the maximum wavelength obtain from absorbance spectrum. It is important that the wavelength is fixed at λmax for the absorbance reading of both standards and sample (unknown). A calibration curve is then prepared by plotting absorbances of the standard solutions versus their concentrations, this plot should be linear ( equation is of the form y = mx ) if holds Beer-Lambert’s law. The sample solution of unknown concentration must be prepared under similar conditions. Calibration curves are used to determine the concentration of the sample. The diagram below shows a beam of monochromatic radiation of radiant power P0 (I0) directed at a sample solution. Absorption takes place and the beam of radiation leaving the sample has radiant power P(I) (Intensity of light). The amount of radiation absorbed may be measured in a number of ways: Transmittance T = I / I0 Absorbance A = log ( I0 / I ) Absorbance is related to transmittance by the equation: A = -log10 T TRANSMITTANCE (T) refers to light passing through a solution. ABSORBANCE (A) refers to light absorbed by a solution. BEER – If all the light passes through a solution LAMBERT’ without any absorption, then absorbance S LAW is 0%, and the transmittance is 100%. If all the light is absorbed, then percent transmittance is 0%, and absorption is 100%. BEER- LAM BERT S LAW Beer-Lambert's law states that: the amount of light absorbed is proportional to the concentration of the solution and the amount of light absorbed is proportional to the distance it travels through the solution (the path length l) OR THE BEER–LAMBERT LAW, states that the degree of absorption at a given wavelength of an absorbing solvent depends on the concentration of compound and the path length of the radiation. BEER- LAM BERT A is the absorbance; E (epsilon) is a constant called molar absorptivity or molar extintion coefficient, and has units M-1 cm-1; It’s a measure of the amount of light absorbed per unit concentration. l is the cell length or path length in cm; C is the concentration of the sample in M. BEER – LAM BERT ’ S LAW The absorbance is also given by the relationship: I0 is intensity of light transmitted through a colorimeter cell containing pure solvent. I is the intensity of the light transmitted through the cell containing the solution under test. BEER – LAM BERT ’ S LAW At low concentrations a At higher concentrations graph of A vs c shows Beer-Lambert’s law is proportionality. It follows not obeyed. Beer-Lambert’s law. CALIBRATION CURVE SHOWINGABSORBANCE VERSUS CONCENTRATION x A at 280 nm 1.0 x 0.5 x 1 2 3 4 5 Concentration (mM) A Slope of Standard Curve = C A = Ecl, if l = 1cm, them a plot of A vs c should be a straight line with intercept 0 and slope E. WORKED EXAMPLE 1 When radiation of wavelength 200nm is passed through solution X in a cell of 1.5 cm path length, its absorption measured on a spectrometer is 0.65 solution X has a molar absortivity of 80. Calculate the concentration of the solution. Rearrange Beer-Lambert’s law in the form A= Elc so that c is the subject: c=A/El Substitute the values: c = 0.65 / 80 x 1.5 c = 5.4 x 10-3 mol, dm-3 WORKED EXAMPLE 2 A solution containing 0.025 mol.dm-3 copper (II) sulphate (solution A) is placed in a spectrometer cell of path length 1cm. The absorbance of this solution is 0.48. Another solution of copper(II) sulphate (solution B) is place in the same cell under the same conditions. The absorbance is 0.18. calculate (i) the concentration of solution B (ii) the molar absorptivity of copper (II) sulphate. Worked example 2. Answer (i) Since absorbance is proportional to concentration if all other factors are constant A of 0.48 → 0.025 moldm-3 CuSO4 A of 0.18 → x 0.025 x 0.18/0.48 = 0.0094 (to 2 s.f) (ii) Rearrange Beer-Lambert’s law in the form A=Elc so the E is the subject: E=A/lc E = 0.48 / 1 x 0.025 = 19.2 QUESTIONS 1. Explain the origin of absorption in UV/VIS spectroscopy 2. Explain why some organic species would absorb light in the ultraviolet region whereas others would not. 3. Outline the basic steps involved in analyzing a sample by UV/VIS spectroscopy 4. a. Define Beer Lambert’s law b. A 1cm3 sample solution of X showed an absorbance of 0.82 at a wavelength of 240nm. Given the absorptivity constant is 12000 the cell path is 1cm, calculate the molar concentration of X in the 1 cm3 sample. c. State the limitations of Beer-Lambert law. 6. Nitrite in water can be determined by its reactions with sulphanilimide and N-(1-naphthyl) ethylenediamine to give a pink colour complex. Five standards solutions of nitrite were prepared, the pink colour was developed and the absorbances were recorded at 550nm as shown in the table below. a. Plot a calibration curve from [Nitrile Absorbanc the data given ]/ ppm e b.Determine by interpolation of the calibration curve, the 2 0.010 nitrile concentration of an 4 0.020 unknown sample which have an absorbance of 0.035. 6 0.031 c. Using Beer-Lambert law, 8 0.041 calculate the molar 10 0.050 absorptivity (E ) assuming that the cell path is 1cm. PPM PPM (parts per million) represents a very small concentration, meaning out of a million. Conversion: 1 ppm = 1 milligram per litre (mg/L) of water. Alternatively, it equals 1 milligram per kilogram (mg/kg) of soil. Relation to Molarity: 1 ppm is equivalent to 1×10𝑀 D I LU T I O N A N D C O N C E N T R AT I O N FA C T O R The dilution factor is calculated using the formula: Example Problem : Calculate the dilution factor when 0.1 mL of a specimen (aliquot) is added to 9.9 mL of diluent Calculate the final volume. Add the aliquot volume to the diluent volume: Final Volume=0.1 mL+9.9 mL=10 ml Calculate the dilution factor. This corresponds to a 102 dilution D I LU T I O N A N D C O N C E N T R AT I O N FA C T O R Determine the Concentration Factor (CF).Concentration factor is the inverse of the dilution factor and is calculated as: This corresponds to a concentration of 10−2. H O W T O P R E PA R E A S O LU T I O N O F A G I V E N D I LU T I O N / C O N C E N T R AT I O N Calculate the Volume of the Aliquot: Use the formula: OR Volume of Aliquot=Concentration Factor × Final Volume Calculate the Volume of the Diluent: Volume of Diluent=Final Volume−Aliquot Prepare the Solution Measure the calculated volume of diluent. Add the calculated volume of aliquot to the diluent. Mix thoroughly. EXAMPLE How would you prepare 20 mL of a 1:50 dilution? Determine required aliquot by dividing final volume by dilution factor: Subtract the aliquot volume from the final volume