UTT Aviation Materials and Hardware 1 PDF
Document Details
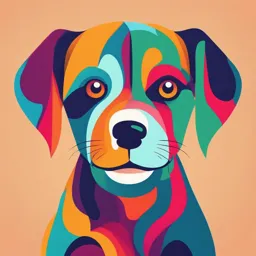
Uploaded by GrandGallium
The University of Trinidad and Tobago
UTT
Raymond Small
Tags
Summary
This presentation covers aircraft materials, focusing on ferrous materials. It details their properties, uses in aircraft, and testing methods. The presentation is from the University of Trinidad and Tobago.
Full Transcript
UTT AVIA 1056 Aircraft Materials and Hardware 1 Instructor Raymond Small Aircraft Materials & Hardware I 1. Aircraft Materials - Ferrous 2. Aircraft Materials - Non Ferrous 3.Composite and Non - Metallic 4. Corrosion Aircraft Material and Hardware I Aircraft Material...
UTT AVIA 1056 Aircraft Materials and Hardware 1 Instructor Raymond Small Aircraft Materials & Hardware I 1. Aircraft Materials - Ferrous 2. Aircraft Materials - Non Ferrous 3.Composite and Non - Metallic 4. Corrosion Aircraft Material and Hardware I Aircraft Materials - Ferrous Aircraft Materials & Hardware I Aircraft Materials - Ferrous 1.Recognize characteristics, properties and identify common alloy steels used in aircraft 2.Test ferrous materials for hardness and tensile strength. 3.Test ferrous materials for fatigue strength and impact resistance. Reference Books Introduction Introduction Knowledge and understanding of the uses, strengths, limitations, and other characteristics of structural metals is vital to properly construct and maintain any equipment, especially airframes. Introduction In aircraft maintenance and repair, even a slight deviation from design specification, or the substitution of inferior materials, may result in the loss of both lives and equipment. The use of unsuitable materials can readily erase the finest craftsmanship. The selection of the correct material for a specific repair job demands familiarity with the most common physical properties of various metals. Introduction The development of new “space age” materials for both aircraft structure and engines has made the vast progress of aviation possible. The basic structure of most of the earliest flying machines were made of strips of spruce or bamboo, and the lifting surfaces were covered with cotton or linen cloth. Introduction After World War I, welded steel tubing replaced the wooden truss used for the fuselage structure of most of the new airplanes. And it was during the 1920s that formed plywood became used for the primary structure of some of the most streamlined and high-strength aircraft of the time. Introduction The technological developments made during World War II allowed the all-metal, stressed-skin, semi-monocoque structure to replace the fabric- covered truss structure in the vast majority of aircraft. This technology was standard until the last few decades when resins reinforced with various types of composite materials have allowed us to build structural shapes that are superior to metals in strength, weight, and rigidity. Introduction Modern aircraft are manufactured from many different types of materials Those most commonly used are aluminum alloy, titanium alloy, Monel, ( a nickel alloy), stainless steel, and Chrome-molybdenum steel. Civilian aircraft are constructed primarily from heat-treated aluminum alloy, while military aircraft (fighters) are constructed primarily from titanium and stainless steel. More and more primary parts are being made of composite. Metals The basic classification of metals for aircraft are ferrous and nonferrous. A ferrous metal is one that contains iron, and in this classification are the various types of steel. Non-ferrous metals are classified into ‘lightweight” and “heavyweight” and include aluminum, magnesium, titanium, and monel. In the aviation industry, titanium with its alloys is considered the heaviest light weight material. Metals Properties Of Metals Of primary concern in aircraft maintenance are such general properties of metals and their alloys as: 1. hardness, 7. brittleness, 2. malleability, 8. fusibility, 9. conductivity 3. ductility, 10.contraction 4. elasticity, 11.expansion, 5. toughness, 12.strength 6. density, Properties Of Metals HARDNESS Hardness refers to the ability of a material to resist abrasion, penetration, cutting action, or permanent distortion. Hardness may be increased by cold working the metal and, in the case of steel and certain aluminum alloys, by heat treatment. Structural parts are often formed from metals in their soft state and are then heat treated to harden them so that the finished shape will be retained. Hardness and strength are closely associated properties of metals. Properties Of Metals STRENGTH One of the most important properties of a material is strength. Strength is the ability of a material to resist deformation. Strength is also the ability of a material to resist stress without breaking. The type of load or stress on the material affects the strength it exhibits. Properties Of Metals DENSITY Density is the weight of a unit volume of a material. In aircraft work, the specified weight of a material per cubic inch is preferred since this figure can be used in determining the weight of a part before actual manufacture. Density is an important consideration when choosing a material to be used in the design of a part in order to maintain the proper weight and balance of the aircraft. Properties Of Metals MALLEABILITY A metal which can be hammered, rolled, or pressed into various shapes without cracking, breaking, or leaving some other detrimental effect, is said to be malleable. This property is necessary in sheet metal that is worked into curved shapes, such as cowling's, fairings, or wingtips. Copper is an example of a malleable metal. Properties Of Metals DUCTILITY Ductility is the property of a metal which permits it to be permanently drawn, bent, or twisted into various shapes without breaking. This property is essential for metals used in making wire and tubing. Ductile metals are greatly preferred for aircraft use because of their ease of forming and resistance to failure under shock loads. Properties Of Metals DUCTILITY For this reason, aluminum alloys are used for cowl rings, fuselage and wing skin, and formed or extruded parts, such as ribs, spars, and bulkheads. Chrome molybdenum steel is also easily formed into desired shapes. Ductility is similar to malleability. Properties Of Metals ELASTICITY Elasticity is that property that enables a metal to return to its original size and shape when the force which causes the change of shape is removed. This property is extremely valuable because it would be highly undesirable to have a part permanently distorted after an applied load was removed. Properties Of Metals ELASTICITY Each metal has a point known as the elastic limit, beyond which it cannot be loaded without causing permanent distortion. In aircraft construction, members and parts are so designed that the maximum loads to which they are subjected will not stress them beyond their elastic limits. This desirable property is present in spring steel. Properties Of Metals TOUGHNESS A material which possesses toughness will withstand tearing or shearing and may be stretched or otherwise deformed without breaking. Toughness is a desirable property in aircraft metals. Properties Of Metals BRITTLENESS Brittleness is the property of a metal which allows little bending or deformation without shattering. A brittle metal is apt to break or crack without change of shape. Because structural metals are often subjected to shock loads, brittleness is not a very desirable property. Cast iron, cast aluminum, and very hard steel are examples of brittle metals. Properties Of Metals FUSIBILITY Fusibility is the ability of a metal to become liquid by the application of heat. Metals are fused in welding. Steels fuse around 2,600 °F and aluminum alloys at approximately 1,100°F. Properties Of Metals CONDUCTIVITY Conductivity is the property which enables a metal to carry heat or electricity. The heat conductivity of a metal is especially important in welding because it governs the amount of heat that will be required for proper fusion. Conductivity of the metal, to a certain extent, determines the type of jig to be used to control expansion and contraction. In aircraft, electrical conductivity must also be considered in conjunction with bonding, to eliminate radio interference. Properties Of Metals THERMAL EXPANSION Thermal expansion refers to contraction and expansion that are reactions produced in metals as the result of heating or cooling. Heat applied to a metal will cause it to expand or become larger. Cooling and heating affect the design of welding jigs, castings, and tolerances necessary for hot rolled material. Ferrous Aircraft Metals Any metal that contains iron as its principal constituent is called a ferrous metal. In aircraft structure we are chiefly concerned with steel, which is iron with a controlled amount of carbon added. Example of Steel Usage for Aircraft Ferrous Aircraft Metals IRON If carbon is added to iron, in percentages ranging up to approximately 1 percent, the product is vastly superior to iron alone and is classified as carbon steel. Carbon steel forms the base of those alloy steels produced by combining carbon steel with other elements known to improve the properties of steel. Ferrous Aircraft Metals IRON A base metal (such as iron) to which small quantities of other metals have been added is called an alloy. The addition of other metals changes or improves the chemical or physical properties of the base metal for a particular use. Steel Manufacturing IRON Iron is, in its pure form, a fairly soft, malleable, and ductile metal that is easy to form or shape. It is silvery white in color and is quite heavy, having a density of 7.9 g/cm cube. Iron easily combines with oxygen to form iron oxide, which is more commonly known as rust. Cast-iron is seldom used in aircraft construction because of its low strength-to- weight ratio. Steel Manufacturing IRON When the iron is moved from the furnace, after it has been taken out of ore, it is in a form known as pig iron. It may be re-melted and cast into cast-iron components, or it may be taken to some of the steel-making furnaces, or converters, to convert the iron into steel Steel Manufacturing UNALLOYED and LOW-ALLOYED STEELS Pig iron from the blast furnace is re-melted in a special furnace where oxygen is forced through the molten metal so that oxygen can unite with the carbon and burn it out. When the carbon is burnt out, a controlled amount of carbon is put back in and, along with other elements that are needed to get the particular characteristics wanted from steel. Steel Manufacturing IRON Iron Ore heated in a furness : cooled = Pig Iron Pig Iron reheated in a furness: cast into a mold = Cast Iron Or Pig Iron remelted carbon burnt off: controlled amounts of Carbon Added = Steel Steel melted and other metals added: steel alloys Steel Manufacturing HIGH ALLOYED STEEL If steel alloy contains more than 5% of an alloying agent, it is considered as a high alloyed steel. High alloyed steel is the kind of steel we use in aircraft construction. it is made in electric furnaces, which allows much better control of the alloying agents than the gas fired furnace Ferrous Aircraft Metals STEEL AND STEEL ALLOYS To facilitate the discussion of steels, some familiarity with their nomenclature is desirable. A numerical index, sponsored by the Society of Automotive Engineers (SAE) and the American Iron and Steel Institute (AISI), is used to identify the chemical compositions of the structural steels. In this system, a four-numeral series is used to designate the plain carbon and alloy steels; five numerals are used to designate certain types of alloy steels. Ferrous Aircraft Metals STEEL AND STEEL ALLOYS The first two digits indicate the type of steel, the second digit also generally (but not always) gives the approximate amount of the major alloying element, and the last two (or three) digits are intended to indicate the approximate middle of the carbon range. However, a deviation from the rule of indicating the carbon range is sometimes necessary. Basic Alloying Elements SAE Notations: For example SAE 4130 steel is Molybdenum steel that contains approximately 1% MO and 0.30% carbon. (Base material is Iron) Ferrous Aircraft Metals STEEL AND STEEL ALLOYS The first two digits indicate the type of steel, the second digit also generally (but not always) gives the approximate amount of the major alloying element, and the last two (or three) digits are intended to indicate the approximate middle of the carbon range. However, a deviation from the rule of indicating the carbon range is sometimes necessary. Ferrous Aircraft Metals STEEL AND STEEL ALLOYS Small quantities of certain elements are present in alloy steels that are not specified as required. These elements are considered as incidental and may be present to the maximum amounts as follows: copper, 0.35 percent; nickel, 0.25 percent; chromium, 0.20 percent; molybdenum, 0.06 percent. Ferrous Aircraft Metals STEEL AND STEEL ALLOYS The list of standard steels Basic Alloying Elements SAE Notations: For example SAE 4130 steel is Molybdenum steel that contains approximately 1% MO and 0.30% carbon. (Base material is Iron) Types, Character, and Uses of Alloyed Steel CARBON STEEL Steel containing carbon in percentages ranging from 0.10 to 0.30 percent is classed as low carbon steel. The equivalent SAE numbers range from 1010 to 1030. Steels of this grade are used for making such items as safety wire, certain nuts, cable bushings, or threaded rod ends. This steel in sheet form is used for secondary structural parts and clamps, and in tubular form for moderately stressed structural parts. Types, Character, and Uses of Alloyed Steel CARBON STEEL Steel containing carbon in percentages ranging from 0.30 to 0.50 percent is classed as medium carbon steel. This steel is especially adaptable for machining or forging, and where surface hardness is desirable. Certain rod ends and light forgings are made from SAE 1035 steel. Types, Character, and Uses of Alloyed Steel CARBON STEEL Steel containing carbon in percentages ranging from 0.50 to 1.05 percent is classed as high carbon steel. The addition of other elements in varying quantities adds to the hardness of this steel. In the fully heat-treated condition it is very hard, will withstand high shear and wear, and will have little deformation. It has limited use in aircraft. SAE 1095 in sheet form is used for making flat springs and in wire form for making coil springs. Ferrous Aircraft Metals NICKLE STEEL The various nickel steels are produced by combining nickel with carbon steel. Steels containing from 3 to 3.75 percent nickel are commonly used. Nickel increases the hardness, tensile strength, and elastic limit of steel without appreciably decreasing the ductility. It also intensifies the hardening effect of heat treatment. SAE 2330 steel is used extensively for aircraft parts, such as bolts, terminals, keys, clevises, and pins. Basic Alloying Elements SAE Notations: For example SAE 4130 steel is Molybdenum steel that contains approximately 1% MO and 0.30% carbon. (Base material is Iron) Ferrous Aircraft Metals NICKEL-CHROMIUM STEEL Nickel gives toughness to steel and chromium hardens it. Nickel chromium steels such as SAE 3130 and 3150 are used for forged and machined parts where high strength, ductility, toughness and shock resistance are needed Ferrous Aircraft Metals CHROME - MOLYBDENUM STEEL Most aircraft structural steel is a chrome-molybdenum alloy that combines toughness and high strength with ease of welding and machining. SAE 4130 steel is one of the most popular alloys, and it is used extensively for steel wilded structures such as fuselage frames, landing gear, and engine mounts. Engine cylinders and other high stressed parts are often made of SAE 4130 steel. Ferrous Aircraft Metals CHROME - MOLYBDENUM STEEL Heat-treated SAE 4130 steel has an ultimate tensile strength of about four times that of SAE 1025 steel, which make it an ideal choice for landing gear structures and engine mounts. Ferrous Aircraft Metals CHROME - VANADIUM STEEL Chrome-vanadium steels are used extensively for wrenches and other hand tools where extremely high strength and toughness are essential. Ferrous Aircraft Metals INCONEL Inconel is a nickel-chromium-iron alloy closely resembling stainless steel (corrosion resistant steel, CRES) in appearance. Aircraft exhaust systems use both alloys interchangeably. Because the two alloys look very much alike, a distinguishing test is often necessary. Ferrous Aircraft Metals END Properties Of Metals Of primary concern in aircraft maintenance are such general properties of metals and their alloys as: 1. hardness, 7. brittleness, 2. malleability, 8. fusibility, 3. ductility, 9. conductivity 4. elasticity, 10.contraction 5. toughness, 11.expansion, 6. density, Basic Alloying Elements SAE Notations: For example SAE 4130 steel is Molybdenum steel that contains approximately 1% MO and 0.30% carbon. (Base material is Iron) Alloying Agents CARBON Carbon is the most important element found in steel, mixing with the iron to form compounds of iron carbides called cementite. It is the carbon in the steel that allows it to be heat-treated to obtain varying degrees of hardness, strength and toughness. The greater the carbon content the better the ability to heat treat and therefor the higher the tensile strength and hardness Alloying Agents CARBON Increasing the carbon content decreases the malleability and weldability of the steel. A heigh-carbon content, up to about 0.953%, may be used where extreme hardness is needed and malleability is not of primary concern. High- carbon steel is used in the manufacture of cutting tools and springs. Alloying Agents CARBON Low-carbon steel that contains about 0.203% carbon is used where a great deal of forming or drawing is needed, and where strength is of minor concern. Medium-carbon steel, is one having between 0.253 and 0.503% carbon, it is used for applications where both strength and ductility, or form ability, are required. Alloying Agents MANGANESE Manganese is alloyed with steel to eliminate some of the oxides and sulphur from steel, making it a clean, tough and uniform metal. Manganese also improves the forging characteristic of the steel by making it less brittle at the rolling and forging temperatures Alloying Agents SILICON When this non-metallic element is alloyed with steel, it acts as a harder. When it is used in small quantities, it improves the ductility of the steel. Alloying Agents PHOSPHOROUS This element raises the yield strength of steel, which improves the resistance of low-carbon steel to atmospheric corrosion. No more that 0.05% phosphorous is normally used in steel, as more of it will cause the metal to become brittle when it is cold. Alloying Agents CHROMIUM The hard high-melting point element is alloyed with steel to increase its strength and wear resistance, as well as its resistance to corrosion. It also gives steel a good measure of hardness. Chromium is usually used in conjunction with other elements, such as molybdenum, nickel, and vanadium, to give the steel the special qualities that are needed in an aircraft structure. Alloying Agents CHROMIUM Chrome-vanadium alloy is used when extreme tough steel is needed for applications such as wrenches and ball bearings Chrome-molybdenum steel has the strength, toughness and lightweight needed to make it the primary steel for aircraft structure and for high- strength engine components such as cylinders. Alloying Agents CHROMIUM Nickel-chrome steel is the primary alloy used in the manufacture of aircraft hard-ware, and because of its strength and hardness at elevated temperatures, tungsten-chrome steel is used for high-speed cutting tools. In addition to its important function as an alloying element in the manufacture of steel, chromium may be electrolytically deposited on cylinder walls and bearing journals to provide a hard, wear resistant surface Alloying Agents MOLYBDENUM One of the most widely used alloying elements for aircraft structural steel is molybdenum. It reduces the grain size of the steel and increases both its impact strength and its elastic limit. Molybdenum steels have some of the best wear resistance and fatigue strength of any of the alloys. This accounts for their popularity for high strength structural members and for engine cylinder barrels. Chrome-molybdenum steel responds very well to heat-treatment, machines easily, and is readily worked. Alloying Agents VANADIUM When extreme high strength is required of steel along with toughness and good ductility, chrome-vanadium steel is used. Vanadium used in amounts up to about 0.20% improves the grain structure and increases both the ultimate tensile strength and toughness. Most wrenches are made of chrome-vanadium steel. Alloying Agents TUNGSTEN Tungsten, which has an extremely high melting point, brings some of this characteristic into steel with which it alloys. Tungsten alloys are used for breaker contacts in magnetos and for cutting tools. Tungsten steels retain their hardness even when operated red-hot. Corrosion-Resistant (Stainless) Steel The development of stainless steel has made possible many of the outstanding advances in aviation, gas-turbine engines and rockets. The most important characteristics of stainless steel are corrosion resistance, strength, toughness, and resistance too high temperatures. Stainless steel can be divided into three general groups based on their structure: austenitic, ferrets martensitic and Precipitation hardenable. Corrosion-Resistant (Stainless) Steel Stainless Steel also designated Corrosion Resistant Steel (CRES) Corrosion-Resistant (Stainless) Steel AUSTENITIC The Austenitic steels are chromium (Cr), nickel (Ni) and chromium-nickel- manganese alloys. They can be hardened only by cold working, and heat treatment serves only to anneal them. They are nonmagnetic in the annealed condition, although some may be slightly magnetic after cold working. Corrosion-Resistant (Stainless) Steel AUSTENITIC Steels are formed by heating the steel mixture above the critical range and holding to form a structure called austenite. A controlled period of partial cooling is allowed followed by rapid quenching just above the critical range. This kind of CRES is widely used in aviation. Corrosion-Resistant (Stainless) Steel FERRITIC Ferritic Steel Contains no carbon; they do not respond to heat treatment. They contain a substantial amount of chromium and many have a small amount of aluminum. They are always magnetic. This kind of CRES is not used in aviation Corrosion-Resistant (Stainless) Steel MARTENSITIC Martensitic steel are straight chromium alloy that harden intensely if they are allowed to cool rapidly from high temperatures. They differ from the 2 preceding groups because they can be hardened by heat treatment. The most widely used stainless steels for general use are those in the 300 series, called 18-8 because they contain approximately 18 percent chromium and 8 percent nickel. (SAE 31188) Corrosion-Resistant (Stainless) Steel PRECIPITATION HARDENABLE The increase of flight speed increased friction with the air and greater heat is developed. Therefor, the need arose for materials that have good corrosion resistance and great strength at room temperature and at moderately high temperature to approximately 400ºC. This led to the development of this kind of steel. They are alloyed with elements such as copper and aluminum. These elements make precipitation hardening possible. Corrosion-Resistant (Stainless) Steel HANDLING PECULIARITIES OF CRES Although stainless steel have many advantages, they are certain disadvantages that must be faced by the fabricator and designer. Stainless steels are more difficult to cut and form than many materials. Stainless steel have a much greater expansion coefficient than other steels, and they conduct heat at a low rate; this makes welding more difficult. Corrosion-Resistant (Stainless) Steel HANDLING PECULIARITIES OF CRES Many of the stainless steels loose their corrosion resistance under high temperature. In welding CRES, inert-gas welding is preferred, because this process causes less deformation due to heat expansion of the metal and it prevents oxidation. Corrosion-Resistant (Stainless) Steel HANDLING PECULIARITIES OF CRES Because of its toughness, stainless steel is more difficult to cut, form, shear machine or drill than ordinary steel. For this reason the technician who is to work with this material successfully must be experienced in the necessary processes or must be directed by an experienced technician. HEAT TREATMENT Of STEEL BEHAVIOR OF STEEL DURING HEATING AND COOLING Changing the internal structure of a ferrous metal is accomplished by heating to a temperature above its upper critical point, holding it at that temperature for a time sufficient to permit certain internal changes to occur, and then cooling to atmospheric temperature under predetermined, controlled conditions. HEAT TREATMENT Of STEEL HEAT TREATMENT Heat treatment is a series of operations involving the heating and cooling of metals in the solid state. Its purpose is to change a mechanical property or combination of mechanical properties so that the metal will be more useful, serviceable, and safe for a definite purpose. By heat treating, a metal can be made harder, stronger, and more resistant to impact. HEAT TREATMENT Of STEEL HEAT TREATMENT Of STEEL Heat treating can also make a metal softer and more ductile. No one heat treating operation can produce all of these characteristics. In fact, some properties are often improved at the expense of others. In being hardened, for example, a metal may become brittle. HEAT TREATMENT Of STEEL STEEL RESPONSE DURING HEAT TREATING When steel is heat treated its temperature increases steadily until it is momentarily checked at the critical point. At this point the metal absorbed heat and changes occur in the structure of the metal, without temperature rise. After this period has passed the temperature continues to rise as before. HEAT TREATMENT Of STEEL STEEL RESPONSE DURING HEAT TREATING If steel having different carbon contents are heated in this way and the critical point plotted on a graph, and if all these points are joined an Iron/Carbon Equilibrium Diagram is produced. As shown. Relationship of Temperature and C-content of Steel 1536ºC = delta 1392ºC = gamma 911ºC = alpha 769ºC HEAT TREATMENT Of STEEL PROCESS OF HEAT TREATING STEEL Most of the heat treatment that are carried out on carbon steels relate to the temperatures on the Iron/Carbon Equilibrium Diagram. HEAT TREATMENT Of STEEL COOLING Iron is an allotropic metal, which means that it can exist in more than one type of lattice structure, depending on the temperature. Molten pure iron will begin to solidify at 1536ºC. The structure at this point is known as the delta form. if cooling continues to 1392ºC the atoms will rearrange themselves into the gamma austenitic form. Iron in this form is nonmagnetic. Relationship of Temperature and C-content of Steel 1536ºC = delta 1392ºC = gamma 911ºC = alpha 769ºC HEAT TREATMENT Of STEEL COOLING When the nonmagnetic austenitic gamma iron is cooled to 911ºC another change occurs. The iron is transformed into a nonmagnetic form of the alpha ferritic structure. if cooling continues to 769ºC the material becomes magnetic within no further changes in the lattice structure ……………………………….. HEAT TREATMENT Of STEEL HEATING Steel (iron + carbon) exist in two basic forms, ferrite and austenite. Ferrite is alpha solid solution of iron into which some carbon has dissolved. It exists at temperatures below the lower critical temperature. Above this lower critical temperature, the steel begins to turn into austenite, which consists of austenitic gamma iron into which carbon has been dissolved. Relationship of Temperature and C-content of Steel 1536ºC = Delta 1392ºC = gamma 911ºC = alpha 769ºC HEAT TREATMENT Of STEEL Heat Treatment Temperatures of Steel Steel HEAT TREATMENT Of STEEL HEATING As the temperature is increased, this transformation of ferrite into austenite continues until the upper critical temperature is reached. Above the upper critical temperature, the entire structure consists of austenite. Below its lower critical temperature the carbon, which exist in the steel in the form of cement (iron carbide Fe3C), is scattered throughout the iron matrix, or its modular structure, as a physical mixture. HEAT TREATMENT Of STEEL HEATING When the steel is heated to its upper critical temperature, this carbon dissolves into the matrix and becomes a solid solution, rather than a physical mixture. The steel has now become austenite, and the iron is in its gamma form. HEAT TREATMENT Of STEEL When the temperature of the steel drops below this critical value, the carbide particles precipitate out of the solution. If the steel is cooled slowly, the particles will be quite large and the steel will be soft, but if it is cooled very rapidly by quenching, the carbon particles will be extremely fine and will effectively bind the molecular structure of the steel together, making it hard and strong. HEAT TREATMENT Of STEEL The critical temperature and the ultimate strength steel will develop, will vary with the alloying agent, but most important factor is the amount of carbon Low-carbon steel will not heat-treat satisfactorily because of the small amount of carbon, but as the amount of carbon is increased the steel gains the ability to be hardened and strengthened by heat treatment. This occurs to about 0.80% carbon. Beyond this, the hardness does not increase, but its wear resistance improves with an increase in the amount of carbon. Relationship of Temperature and C-content of Steel 1536ºC = Delta 1392ºC = gamma 911ºC = alpha 769ºC HEAT TREATMENT PROCESSES TEMPERING Tempering reduces the brittleness imparted by hardening and produces definite physical properties within the steel. Tempering always follows, never precedes, the hardening operation. In addition to reducing brittleness, tempering softens the steel. Tempering is always conducted at temperatures below the low critical point of the steel. In this respect, tempering differs from annealing, normalizing, or hardening, all of which require temperatures above the upper critical point. HEAT TREATMENT Of STEEL Heat Treatment Temperatures of Steel HEAT TREATMENT PROCESSES TEMPERING The hardened steel may be tempered, or have some of the hardness drawn out, by heating it to a temperature considerably below its critical temperature and holding it there until it has reached the temperature throughout. It is then allowed to cool to room temperature in still air. Tempering not only reduces hardness, it will also relieve stress and improve the ductility and toughness of the steel. HEAT TREATMENT PROCESSES ANNEALING Annealing of steel produces a fine grained, soft, ductile metal without internal stresses or strains. In the annealed state, steel has its lowest strength. In general, annealing is the opposite of hardening. Annealing of steel is accomplished by heating the metal to just above the upper critical point (871°C) soaking at that temperature, and cooling very slowly in the furnace. HEAT TREATMENT PROCESSES ANNEALING To produce maximum softness in steel, the metal must be cooled very slowly. Slow cooling is obtained by shutting off the heat and allowing the furnace and metal to cool together to 480°C or lower, then removing the metal from the furnace and cooling in still air. Another method is to bury the heated steel in ashes, sand, or other substance that does not conduct heat readily. HEAT TREATMENT PROCESSES NORMALISING The normalizing of steel removes the internal stresses set up by heat treating, welding, casting, forming, or machining. Stress, if not controlled, will lead to failure. Because of the better physical properties, aircraft steels are often used in the normalized state, but seldom, if ever, in the annealed state. HEAT TREATMENT PROCESSES NORMALISING The Steel is heated to about 40 °C above its upper critical temperature and held until this temperature is uniform throughout. Then it is removed from the Furnace and allowed to cool in still air. The particles of carbon that precipitate out are not as large as those formed when the steel is annealed, but the molecular stresses are relieved. HEAT TREATMENT PROCESSES HARDENING If the steel is to be hardened, it is heated above its critical temperature so the carbon can enter into uniform solution with the iron matrix and is then cooled rapidly by quenching in water or oil or brine. The speed of the quenching is determined by the quenching medium. Oil provides the slowest quenching and brine the most rapid. HEAT TREATMENT PROCESSES HARDENING If the squelch is quick enough, insufficient time is allowed for the carbon to precipitate out, and it becomes trapped in solution. The resultant structure is known as martensite, and is a supersaturated solid solution of carbon in an iron matrix. Although martensite, is the hardest possible structure, it is not the desired result of heat-treatment. Martensite is far too hard and brittle for most applications, and the final product must be obtained by tempering. Ferrous Aircraft Metals END HEAT TREATMENT PROCESSES CASE HARDENING Case hardening produces a hard wear-resistant surface or case over a strong, tough core. Case hardening is ideal for parts which require a wear- resistant surface and, at the same time, must be tough enough internally to withstand the applied loads. There are two ways that case hardening is done. The first is a process known as carburizing and the second is nitriding. HEAT TREATMENT PROCESSES CARBURIZING Carburizing is a case hardening process in which carbon is added to the surface of low carbon steel. Thus, a carburized steel has a high carbon surface and a low carbon interior. When the carburized steel is heat treated, the case is hardened while the core remains soft and tough. There three methods that can be used to increase the carbon content of the surface of a low-carbon steel. HEAT TREATMENT PROCESSES CARBURIZING Pack Carburizing is done by enclosing the part in a fire-clay container and packing it with a carbon-rich material such as charcoal. The container is sealed and placed in a furnace where it is heated to 927°C. Carbon monoxide gas forms from the heated charcoal, and since it cannot escape, its carbon combines with the gamma iron in the surface of the steel. The depth to which this carbon penetrates depends upon the soak time HEAT TREATMENT PROCESSES CARBURIZING Gas Carburization is similar to pack carburization, except that the carbon monoxide is produced by a gas rather than a solid material. In this process, as with pack carburizing, the carbon from carbon monoxide combines with the gamma iron and forms a high-carbon surface. HEAT TREATMENT PROCESSES CARBURIZING Liquid Carburization produces the high-carbon surface when the part is heated in a molten bath of sodium cyanide or barium cyanide, which supplies the needed carbon. Any portion of the part that should not be case hardened may be copper- plated. The carbon will not infuse into the copper-plated surface HEAT TREATMENT PROCESSES NITRIDING Nitriding differs from carburizing in that the part to be hardened is first heat-treated to give it the characteristics we want that is, it is hardened, tempered, and then ground to the proper dimensions. It is then placed in a special retort and heated to a temperature of approximately 538°C, and is surrounded by ammonia gas (CNH3) HEAT TREATMENT PROCESSES NITRIDING The heigh temperature breaks the ammonia down into nitrogen and hydrogen. The nitrogen reacts with the steel and with any of the nitride forms in the steel such as aluminum, chromium, molybdenum or vanadium, to form the appropriate nitrides. HEAT TREATMENT PROCESSES NITRIDING The nitride surface is extremely thin, with its thickness depending on the length of time it is exposed to the ammonia gas. The hardness of the surface gradually decreases with depth until it reaches that of the core. Aircraft engine crankshafts and cylinder walls are commonly nitrided for increased wear resistance. But these surfaces have one serious problem: they are highly susceptible to pitting corrosion and must be protected from air with a coating of oil. Production Methods FORGING Forging is the process of forming a product by hammering or pressing. When the material is forged below the recrystallization temperature, it is called cold forged. When worked above the recrystallization temperature, it is referred to as hot forged. Production Methods FORGING Drop forging is a hammering process that uses a hot ingot that is placed between a pair of formed dies in a machine called a drop hammer and a weight of several tons is dropped on the upper die. This results in the hot metal being forced to take the form of the dies. Because the process is very rapid, the grain structure of the metal is altered, resulting in a significant increase in the strength of the finished part. Production Methods CASTING Casting is formed by melting the metal and pouring it into a mold of the desired shape. Since plastic deformation of the metal does not occur, no alteration of the grain shape or orientation is possible. Both iron and aluminum alloys are cast for aircraft uses Production Methods EXTRUDING The extrusion process involves the forcing of metal through an opening in a die, thus causing the metal to take the shape of the die opening. The shape of the die will be the cross section of an angle, channel, tube, or some other shape. Aluminum is the most extruded metal used in aircraft. Production Methods COLD WORKING/HARDENING Cold working applies to mechanical working performed at temperatures below the critical range. It results in a strain hardening of the metal. In fact, the metal often becomes so hard that it is difficult to continue the forming process without softening the metal by annealing. Production Methods COLD WORKING/HARDENING While there are several cold working processes, the two with which the aviation mechanic will be principally concerned are cold rolling and cold drawing. These processes give the metals desirable qualities which cannot be obtained by hot working. Production Methods COLD ROLLING Cold rolling usually refers to the working of metal at room temperature. In this operation, the materials that have been rolled to approximate sizes are pickled to remove the scale, after which they are passed through chilled finishing rolls. This gives a smooth surface and also brings the pieces to accurate dimensions. The principal forms of cold rolled stocks are sheets, bars, and rods. Production Methods COLD DRAWING Cold drawing is used in making seamless tubing, wire, streamlined tie rods, and other forms of stock. Wire is made from hot rolled rods of various diameters. These rods are pickled in acid to remove scale, dipped in lime water, and then dried in a steam room where they remain until ready for drawing. The lime coating adhering to the metal serves as a lubricant during the drawing operation. Production Methods COLD DRAWING The size of the rod used for drawing depends upon the diameter wanted in the finished wire. To reduce the rod to the desired size, it is drawn cold through a die. One end of the rod is filed or hammered to a point and slipped through the die opening. Here it is gripped by the jaws of the drawing block and pulled through the die. This series of operations is done by a mechanism known as a drawbench. Determining the Temperature of Steel If we need to heat treat steel and we do not have a pyrometer to measure its temperature, we can make a reasonable estimate of its temperature by observing its color. Relationship Between Steel Colour and Temperature HEAT TREATMENT Of STEEL Heat Treatment Temperatures of Steel Tempering Small Tools An example of tempering steel, when we make a screwdriver blade, chisel, or punch, from tool-steel rod. Anneal This steel has a high carbon content and is usually obtained in its normalized condition. After the tool has been shaped it is heated to a cherry red color and quenched in oil. Tempered Then a portion is polished and re-heated until the proper oxide color appears. The steel is again quenched and it should have the proper hardness. Annealing Colour Chart of Tool Steel Oxides Testing of Ferrous Materials HARDNESS TESTING Hardness testing is a method of determining the results of heat treatment as well as the state of a metal prior to heat treatment. Since hardness values can be tied in with tensile strength values and, in part, with wear resistance, hardness tests are a valuable check of heat-treat control and of material properties. Practically all hardness testing equipment now uses the resistance to penetration as a measure of hardness. Testing of Ferrous Materials RELATIONSHIP BETWEEN HARDNESS AND STRENGTH The smaller the carbon particles that are in solution with the matrix of the steel the harder and stronger the steel will be. There is a definite relationship between strength and hardness of steel. This relationship does not necessarily exist, however with metals other than steel. There are two systems of measuring the hardness of steel in common use. 1. the Brinell hardness tester 2. the Rockwell hardness tester Testing of Ferrous Materials THE BRINELL HARDNESS TESTER The Brinell hardness tester uses a hardened spherical ball, which is forced into the surface of the metal. This ball is 10 millimeters (0.3937 inch) in diameter. A pressure of 3,000 kilograms is used for ferrous metals and 500 kilograms for nonferrous metals. The pressure must be maintained at least 10 seconds for ferrous metals and at least 30 seconds for nonferrous metals. Testing of Ferrous Materials THE BRINELL HARDNESS TESTER The load is applied by hydraulic pressure. The hydraulic pressure is built up by a hand pump or an electric motor, depending on the model of tester. A pressure gauge indicates the amount of pressure. There is a release mechanism for relieving the pressure after the test has been made, and a calibrated microscope is provided for measuring the diameter of the impression in millimeters. ss) Steel Brinell Hardness Tester Testing of Ferrous Materials THE BRINELL HARDNESS TESTER To determine the Brinell hardness number for a metal, measure the diameter of the impression, using the calibrated microscope furnished with the tester. Then convert the measurement into the Brinell hardness number on the conversion table furnished with the tester. Conversion of different hardness testers values Testing of Ferrous Materials THE ROCKWELL HARDNESS TESTER The Rockwell hardness tester measures the resistance to penetration, as does the Brinell tester. Instead of measuring the diameter of the impression, the Rockwell tester measures the depth, and the hardness is indicated directly on a dial attached to the machine. Testing of Ferrous Materials THE ROCKWELL HARDNESS TESTER The dial numbers in the outer circle are black, and the inner numbers are red. Rockwell hardness numbers are based on the difference between the depth of penetration at major and minor loads. The greater this difference, the lower the hardness number and the softer the material Testing of Ferrous Materials THE ROCKWELL HARDNESS TESTER Two types of penetrators are used with the Rockwell tester: a diamond cone and a hardened steel ball. The load which forces the penetrator into the metal is called the major load and is measured in kilograms. The results of each penetrator and load combination are reported on separate scales, designated by letters. The penetrator, the major load, and the scale vary with the kind of metal being tested. ROCKWELL HARDNESS TERTER For hardened steels, the diamond penetrator is used Testing of Ferrous Materials STANDARD ROCKWELL HARDNESS SCALE Testing of Ferrous Materials ROCKWELL HARDNESS TERTER For hardened steels, the diamond penetrator is used; the major load is 150 kilograms; and the hardness is read on the “C” scale. When this reading is recorded, the letter “C” must precede the number indicated by the pointer. Testing of Ferrous Materials ROCKWELL HARDNESS TERTER The C-scale setup is used for testing metals ranging in hardness from C-20 to the hardest steel (usually about C-70). If the metal is softer than C-20, the B-scale setup is used. With this setup, the 1⁄16-inch ball is used as a penetrator; the major load is 100 kilograms; and the hardness is read on the B-scale. Testing of Ferrous Materials THE VICKERS HARDNESS TESTER Standard method for measuring the hardness of metal, particularly those with extremely hard surfaces: the surface is subject to a standard pressure, which depends on the material, for a standard length of time by means of a pyramid-shaped diamond. The diagonal of the resulting indention is measured under a microscope and the Vickers hardness value read from a conventional table. Testing of Ferrous Materials TENSILE STRENGTH TEST Materials strength testing is largely done by manufacturers and design engineers long before the field technician ever encounters the aircraft for maintenance. Testing of Ferrous Materials TENSILE STRENGTH TEST The tensile strength of a material is what is commonly thought of as the strength of a material. It is a measurement of tension, which is the stress that resists a force that tends to pull a material apart. Testing of Ferrous Materials TENSILE STRENGTH TEST To perform a test of tensile strength, the material is secured in a specially designed electromagnetically or hydraulically powered machine that exerts force on the material to pull it apart. While the force is applied and increased, dimensional measurements are made and recorded. Most materials will elongate before the force becomes so great that there is critical failure. Testing of Ferrous Materials TENSILE STRENGTH TEST Many materials are tested along a single axis since they are isotropic. This means tensile strength is uniform in all directions. Aviation metals are in this category. Aircraft fabrics and composites are anisotropic, meaning that tensile strength varies depending upon which direction the force is applied. Testing of Ferrous Materials TENSILE STRENGTH TEST Testing of Ferrous Materials FATIGUE STRENGTH TEST Fatigue is a weakness in materials, especially metals, caused by repeated variations of stress. Fatigue is of great concern in aviation due to the constantly changing loads experience by nearly all parts of the structure during operation. Testing of Ferrous Materials FATIGUE STRENGTH TEST The critical issue with fatigue is that numerous, repeated applications of over and over a relatively small load can result in failure. A single small load of the size under considerations size would have virtually no effect on the material. But application and release of the small load gradually weakens it. An example of fatigue failure is bending a piece of metal back and force until is weakened and breaks. Testing of Ferrous Materials FATIGUE STRENGTH TEST Fatigue failure is caused by stress cycles, such as reversal or alternating stress. Reversal of direction of bending or torsion, of alternating compression and tensile stress Fluctuating stress, variation of intensity of stress Repeated stress, application and removal of stress Testing of Ferrous Materials FATIGUE Testing of Ferrous Materials FATIGUE STRENGTH TEST This form of failure is encountered very frequently in engineering, and, although much publicity has been given to the fatigue of aircraft structures, this form of failure is very common in non-aviation circumstances. Testing of Ferrous Materials FATIGUE STRENGTH TEST When the fatigue resistance of a material is to be determined, a number of test-pieces are tested. The number of stress cycles that produce failure is recorded. The first test is carried out with a high maximum stress, and each subsequent test employs a lower maximum stress. Testing of Ferrous Materials FATIGUE STRENGTH TEST The object of the series of test is to determine the highest maximum stress that will permit a given “life” in terms of stress cycles. Alternately the fatigue limit may be required. This is the highest maximum stress that will permit the material to undergo an infinite number of stress cycles before failure Testing of Ferrous Materials FATIGUE STRENGTH TEST If fatigue conditions are present, the material will fail at a stress that is much lower than that associated with a static force. The fatigue strength of a material is between 0.35 and 0.65 of the tensile strength, depending upon composition; but the fatigue strength of a component is reduced by poor finish, sharp corners, and corrosion. Testing of Ferrous Materials FATIGUE STRENGTH TEST Metal fatigue can cause micro cracks and other fatigue damage precursors that are sought during periodic inspection. Visual, eddy current, ultra sound, fluorescent penetrant, magnetic particle and even radiography inspection techniques are all employed in various situation to detect metal fatigue. Testing of Ferrous Materials FATIGUE STRENGTH TEST The progression from a micro crack to cracks that join too cause a large section of metal to fail can be rapid. It is critical, especially on aircraft with a high number of operating cycles, to follow all manufacturer's specifications for investigating fatigue. Testing of Ferrous Materials FATIGUE STRENGTH TEST New studies and method of detection are being developed such as electrochemical and light scattering inspection processes. Some country authorities have placed limits on the number of cycles an aircraft may accrue before it must be frequently inspected or retired from service. Testing of Ferrous Materials IMPACT RESISTANCE TEST Impact tests are used to indicate the toughness of a material and most importantly its ability to resist mechanical shock, to ensure that temper brittleness has not been introduced during heat treatments. Toughness is, broadly, a measure of the amount of energy required to cause an item - a test piece or a bridge or a pressure vessel - to fracture and fail. The more energy that is required the tougher the material. Testing of Ferrous Materials IMPACT RESISTANCE TEST There are two types of machine used for testing aircraft materials, both of which use a pendulum weight to fracture the specimen. The energy absorbed by the specimen is measured from the angle through which the pendulum swings after causing the fracture. Testing of Ferrous Materials IMPACT RESISTANCE TEST A metallurgist named IZOD invented an impost-test for determining the suitability of various metals to be used as cutting tools. The test involved a pendulum with a known weight at the end of its arm swinging down and striking the specimens as it stood clamped in a vertical position rials IZOD IMPACT TESTER Testing of Ferrous Materials IMPACT RESISTANCE TEST Another metallurgist named CHARPY modified the test slightly by orienting the specimens in a horizontal fashion Where the test piece must be tested at high or low temperatures the CHARPY test is used. The test is carried out within the 6 seconds of removal of the test piece from the heating or cooling bath. Testing of Ferrous Materials IMPACT RESISTANCE TEST Within recent times it became apparent that higher velocities and impact energies could be achieved by vertical style drop towers and thus the trend shifted. Pendulum machines remained popular with those testing to IZZOD and CHARPY while more high speed, product oriented impact applications became the dominion of the drop tower Testing of Ferrous Materials IMPACT RESISTANCE TEST The Drop Tower allowed the user to use one impact test system to not only test CHARPY and IZOD specimens, but also test components and flat specimens. Impact Testing Machines Testing of Ferrous Materials END