NUFS 372/373 Protein Lectures - Updated Jan 29 2025 - PDF
Document Details
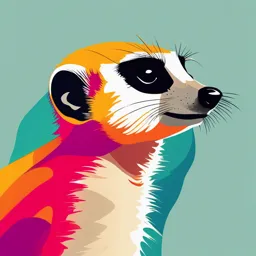
Uploaded by InspirationalOcarina
University of Alberta
2025
NUFS
Rickey Yada
Tags
Summary
These are updated lecture notes for NUFS 372/373 covering the structure, functions, and roles of proteins and amino acids. The document includes important concepts on different aspects of protein functions and touches on their various uses in food systems.
Full Transcript
NUFS 372/373 Amino acids, Peptides and Proteins Rickey Yada [email protected] Outline of topics Amino acid structure and classification Nutritional factors Role of amino acids in foods Reactions of amino acids Classification and structure of proteins Pr...
NUFS 372/373 Amino acids, Peptides and Proteins Rickey Yada [email protected] Outline of topics Amino acid structure and classification Nutritional factors Role of amino acids in foods Reactions of amino acids Classification and structure of proteins Protein-protein interactions Denaturation Functional properties Example: Milk protein: structure and properties Amino acids: α-amino acids are the basic structural units of protein. Consist of an α-carbon atom covalently attached to a hydrogen atom, an amino group, a carboxyl group and a side chain R group Amino acids differ only in the chemical nature of the side chain of the R group. https://www.imgt.org/IMGTeducation/Aide- memoire/_UK/aminoacids/formuleAA/ i. Amino acid structure The alpha carbon is an asymmetric carbon atom in all amino acids (except for glycine), thus amino acids exist in two optically active forms: L- and D- isomers. Alpha-carbon Bitter taste Sweet taste ii) Solubility of amino acids Differences in solubility properties of amino acids is due to the type of side chain. Dependent on the dissociation properties of the amino acid, known as dissociation constant (pK). pK1 pK2 + H3N–R–COOH +H3N–R–COO¯ H2N–R–COO¯ H+ – H+ Acidic Neutral Basic (pI) https://socratic.org/questions/what-is-the- titration-curve-of-glycine iii) Nutritional Quality-Essential Amino Acids Essential amino acids, also known Isoleucine (Ile) as indispensable amino acids, are Leucine (Leu) amino acids that humans and Lysine (Lys) – wheat, corn other vertebrates cannot synthesize from metabolic Methionine (Met) – soy intermediates. These amino acids Phenylalanine (Phe) must be supplied from an Threonine (Thr) exogenous diet because the Tryptophan (Trp) – corn human body lacks the metabolic Valine (Val) pathways required to synthesize these amino acids.– growth and Histidine (His) health. https://pubmed.ncbi.nlm.nih.gov/32496725/#:~:text= Essential%20amino%20acids%2C%20also%20kno wn,to%20synthesize%20these%20amino%20acids. There are 9 essential amino acids for human nutrition. Nutritional factors Limiting amino acids Quality of proteins depends on the richness of essential amino acids (limiting amino acids) and the digestibility of the protein. “The term "limiting amino acid" is used to describe the essential amino acid present in the lowest quantity in a food protein relative to a reference food protein like egg whites. The term "limiting amino acid" may also refer to an essential amino acid that does not meet the minimal requirements for humans.” (e.g. gelatin an animal protein, lacks 4 eAA). https://pubmed.ncbi.nlm.nih.gov/32496725/#:~:text=Essential%20amino%20acids%2C%20also%20known,to%20syn thesize%20these%20amino%20acids Limiting amino acids affect the net protein utilization or biological value. Role of amino acids in foods iv). Structural attributes Different amino acids provides different physico- chemical properties to peptide chains or protein molecules. For example: the nonpolar side chains of valine, isoleucine, leucine are branched and thus restricts their internal flexibility and rise to surface hydrophobicity. https://www.imgt.org/IMGTeducation/Aide- memoire/_UK/aminoacids/formuleAA/ Role of amino acids in foods Structural attributes cont’d Polar side chains form hydrogen bonds. For example: serine and threonine have hydroxyl groups that form hydrogen bonds, and cysteine has an important role in forming sulfydryl (S-S) (cystine) bridges between different parts of the peptide chain. https://www.imgt.org/IMGTeducation/Aide- memoire/_UK/aminoacids/formuleAA/ Role of amino acids in foods v). Taste and taste-enhancing ability Example: L-glutamate also suppresses certain “undesirable” flavors, such as: - the sharpness of onion flavor. - rawness in many vegetables - earthiness of potatoes. Glutamate activity to modulate taste in foods is noticeable between pH 3.5 to 7.2, being more intense at lower pH. Role of amino acids in foods Sweetness perception of D-amino acids Sweet - glycine, alanine, glutamine, and threonine – hydrogen group Bitter - arginine, histidine, isoleucine, leucine, methionine, phenylalanine, tyrosine, and valine – hydrophobic group Salty - both acid and basic groups, e.g., lysine, glutamic acid, aspartic acid, arginine Sour - Glutamic and aspartic acid - acids Unami (savory or meaty taste of foods) – Glutamate (mono–sodium salt of glutamic acid) https://www.imgt.org/IMGTeducation/Aide- memoire/_UK/aminoacids/formuleAA/ Reactions of amino acids Carbonyl–Amine reactions Maillard reaction has the greatest impact on protein sensory and nutritional properties. Occurs both in food and biological systems. Amino component: proteins and amino acids. Carbonyl component: reducing sugars, ascorbic acid, and carbonyl compounds. Maillard Reaction Hodge, J.E. (1953) Dehydrated Foods, Chemistry of Browning Reactions in Model Systems. Journal of Agricultural and Food Chemistry, 1, 928-943. https://doi.org/10.1021/jf60015a004 vi). Carbonyl–Amine reactions: Strecker degradation Part of the Maillard Amino acids are reaction. degraded to aldehydes, pyrazines, sugar fragments, ammonia, and The oxidation of amino carbon dioxide. acids by an α-dicarbonyl or other conjugated dicarbonyl compound that Strecker degradation of is produced on the each amino acid breakdown of Amadori produces a specific compounds. volatile product and provide unique aromas and flavors. Carbonyl–Amine reactions Strecker degradation Amadori Compounds https://www.biosyn.com/tew/The-Maillard-reaction-and-Amadori- rearrangement.aspx Carbonyl–Amine reactions Strecker degradation Distinctive aroma Amino acid Heated at 100˚C Heated at 180˚C Valine Rye bread Chocolate Leucine Sweet, chocolate Burnt cheese Proline Burnt Pleasant bakery odor Arginine Popcorn Burnt sugar Reactions of amino acids vii). Enzymatic reactions: tyrosine oxidation The presence of oxygen and the enzyme tyrosinase (often polyphenol oxidase) are essential for the oxidation of tyrosine to dihydroxy-phenyalanine (DOPA). Copper is an essential co-factor for the tyrosinase activity. This is often termed “Enzymatic Browning” https://www.linkedin.com/pulse/why-do- some-fruits-vegetables-turn-brown-when- exposed-mukherjee/ Enzymatic reactions (polyphenol oxidase): tyrosine oxidation Reactions of amino acids cont’d. Enzymatic reactions: tyrosine oxidation The reaction requires copper as a catalyst. Optimal pH ranges from 6.7 – 7.2 Sequestering copper agents (e.g., organic acids) and decreasing pH result in the inhibition of the tyrosinase enzyme. The reaction is also reduced by: Reduction of oxygen concentration (e.g., vacuum packaging) Use of reducing agents (ascorbic acid, sulfites). https://pmc.ncbi.nlm.nih.gov/articles/PMC8 839884/figure/molecules-27-01101-f001/ Reactions of amino acids Decarboxylation reactions: Biogenic amines Amino acid decarboxylation reactions result in the formation of biogenic amines (e.g., vasoactive amines) Decarboxylation of amino acids: Tyrosine to tyramine Histidine to histamine, Phenylalanine to phenylethyl- amine, Arginine to putrescine. Bioactive amines are result of the action of bacterial enzyme: L-amino acid decarboxylase. Reactions of amino acids Decarboxylation reactions: tyramine Tyramine is naturally present in small amounts in bananas, pineapples, and it is produced by streptococci action in cheddar cheese. Tyramine poisoning can occur as low as 40mg. Tyramine causes hypertension, intense headache. Reactions of amino acids Decarboxylation reactions: histamine Histamine is formed from the enzymatic action of gram- negative bacteria (Proteus morganii; Morganella morganii). Mainly found in fish (e.g., mackerel, tuna) Poisoning symptoms include headache, nausea, hypertension, and vomiting. Reactions of amino acids Decarboxylation reactions: histamine Histamine production can also be synthesized by the normal flora of the colon. Intake of foods rich is histidine may favor endogenous production of histamine. Histidine decarboxylase is also present in tissue, mast cells and blood cell basophils, thus typical signs of inflammation can be due to the release of this enzyme. https://pubs.rsc.org/en/content/chapterhtml/2019/bk9781788014366-00001?isbn=978-1-78801-436- 6#:~:text=Biogenic%20amines%20(BAs)%20are%20important,microbial%20decarboxylation%20of%20amino%20acids. Reactions of amino acids Decarboxylation reactions: putrescine and tryptamine (A) (B) Biogenic amines in foods Amino oxidation products Protein in different food matrices like beef patties, chicken meat, raw pork and different types of fish can be oxidized during frozen storage The usage of sodium nitrite, in cured meat, is another cause of protein oxidation Specific amino acids are involved: Amino acid oxidation: lysine (alpha-aminoadipic acid), tyrosine (dityrosine, kynurenine) tryptophan (kynurenic acid) Consequences? Protein functionality & human health Reactions of amino acids Reactions with secondary amines Nitrites are used for preservation against C. botulinum. Nitrite is reduced to nitrous anhydride, which reacts with amines containing ionizable groups, forming nitrosamines (risk of cancer). Nitrosamides are derivatives of substituted amides. Nitrosamine formation https://www.google.ca/search?q=nitrosamine+formation+mechanism+in+foods+images&sca_esv=3cb0aa99076b7154&ei=AiKaZ6zzNc7D0PEPjpLFqAo&oq=n itrosamine+formation+mechanism+in+foods+image&gs_lp=Egxnd3Mtd2l6LXNlcnAiLm5pdHJvc2FtaW5lIGZvcm1hdGlvbiBtZWNoYW5pc20gaW4gZm9vZHMga W1hZ2UqAggAMgUQIRigATIFECEYoAEyBRAhGKABSOw2UKEIWJkicAF4AJABAJgBggGgAaQGqgEDNy4yuAEByAEA- AEBmAIJoALzBcICChAAGLADGNYEGEfCAgcQIRigARgKwgIEECEYFcICBRAhGJ8FmAMAiAYBkAYIkgcDNy4yoAeLNQ&sclient=gws-wiz- serp#imgrc=_7AsUAzsBYjbhM&imgdii=484luLYpFRZkSM Reactions of amino acids Reactions with secondary amines α-amino acids contain ionizable groups are: proline, tryptophan, tyrosine, cysteine, arginine, histidine. Nitrosation of proline Reactions of amino acids Reactions with secondary amines Nitrosamines are produced under conditions of low pH (stomach environment) and at high temperatures (e.g., frying, roasting). Nitrosamines are only carcinogenic when metabolic activated by the hosts oxidative enzymes. https://www.sciencedirect.com/referencework/9780123786135/encyclopedia- of-food-safety II. Proteins Organic compounds formed by 100–500 amino acids linked together. Proteins are also necessary in animals' diets, since animals cannot synthesize all the amino acids they need and must obtain essential amino acids from food. Amino acids are built from a central carbon bonded to four different groups. hydrogen (–H), amino group (–NH2), carboxyl group (–COOH), and side chain symbolized by “R”. Classification of proteins (next to water, protein in the most plentiful substance in body). Simple Proteins Yield amino acids on hydrolysis: Albumins: egg, albumin, lactalbumin, serum albumin - soluble in water Globulins: serum globulin, β-lactoglobulin, myosin, actin, glycinin - soluble in neutral salt solutions Glutelins: glutenin and oryzenin - soluble in dilute acid or alkali Prolamins: proteins found in cereals, rich in lysine and arginine - soluble in 70% alcohol Classification of proteins Conjugated Proteins These proteins contain an amino acid combined with a non-protein constituent: Phosphoproteins: phosphate groups linked to hydroxyl groups of serine or threonine; e.g. casein in milk and phosphoprotein in yolk. Lipoproteins: combination of lipids and protein with excellent emulsifying properties. Nucleoproteins: combination of nucleic acid with protein. Found in cell nuclei. Glycoproteins: with carbohydrate (8-20%). Has strong allergenic properties, e.g. ovomucin in egg white. Chromoproteins: have prosthetic groups, e.g., hemoglobin, chlorophyll, myoglobin, flavoproteins Classification of proteins Derived/By-products Proteins Derived proteins/by-products are obtained by chemical or enzymatic methods. 1) Primary-protein derivatives: Limited hydrolysis of proteins (kappa–casein to para – kappa casein + glycomacropeptide) – resulting in coagulated proteins, e.g., rennet coagulated casein/cheese curd. 2.) Secondary derivatives: Peptides: a combination of two or more amino acids. Examples are the breakdown products formed during processing of milk products: the peptides formed during the ripening of cheese.- bitterness i). Protein structure Four levels of protein structure: Primary Secondary Tertiary Quaternary https://www.khanacademy.org/science/biology/macromolecules/p roteins-and-amino-acids/a/orders-of-protein-structure https://www.medschoolcoach.com/levels- of-protein-structure-mcat-biochemistry/ Proteins Primary structure Primary structural properties of proteins (sequence of the amino acids) are associated with the properties of individual amino acids comprising the protein. Amino acids are linked by covalent bonds through amide bonds, also known as peptide bonds. https://byjus.com/jee/peptide-bond/ Proteins- Secondary Structure: Refers to the periodic spatial arrangement of amino acid residues at certain segments of the polypeptide chain. The regularly repeating local structures are stabilized by hydrogen bonding; notable examples are the alpha helix, beta strand (structures), beta turns and random coil. Two forms of periodic secondary structures are found in proteins: helical and sheet structures. Because secondary structures are local, many regions of different secondary structure can be present in the same protein molecule. Proteins α-helix secondary structure From the helical structures the major form in proteins is the α-helix, which is the most stable. Each backbone N-H group is hydrogen bonded to the C=O group of the fourth preceding residue. Can exist in either right or left-handed orientation. The right-handed α-helix is the more stable. The helix makes a complete turn every 3.6 amino acids. α-helix secondary structure Proteins β-strands secondary structure In this form, the C=O and N-H groups are oriented perpendicular to the direction of the chain. Hydrogen bonding is possible between segments and not within a segment. β-sheet structure is comprised of individual β-strands. β-strands are usually 5-15 amino acids residues long. Proteins β-strand (sheet) secondary structure In a protein, 2 β-strands of the same molecule interact by hydrogen bonds, forming a sheet-like structure known as β-pleated sheet. β-strands often have a hydrophilic and a hydrophobic side. β-sheet is generally more stable than the α-helix. β-sheet structure are generally fibrous in nature and insoluble in aqueous solvents. Examples: β-lactoglobulin, soy globulin. β-structure secondary structure https://chemistry.stackexchange.com/questions/143189/why-are- hydrogen-bonds-in-an-antiparallel-beta-sheet-stronger-than-those- in-para β-Turns Polypeptide turns back on itself– 180o https://www.sciencedirect.com/refere ncework/9780128032015/comprehe nsive-medicinal-chemistry-iii Proteins Tertiary structure Refers to the spatial arrangement attained when a linear protein chain with defined (α-helix, β-sheets) or non-defined (random coil) secondary structure segments folds further into a compact 3-dimensional form. Amino acid chain linkages fold over into compact structures stabilized by hydrogen bonds, hydrophobic, electrostatic, disulphide bridges and van der Waals forces. Proteins Quaternary structure Quaternary structure refers to the spatial arrangement of a protein when it contains more than one polypeptide. Formation of oligomeric structures is the result of specific protein-protein interactions. Typical-11S-soy-protein https://www.researchgate.net/figure/Protein-structure-of-a- soybean_fig2_282946577 ii). Protein-protein interactions Protein-protein interactions Protein-protein interactions Summary of protein-protein interactions Interactions that determine secondary and tertiary structure of proteins: (A) Hydrogen bond; (B) van der Waals; (C) Hydrophobic interactions; (D) Disulfide links; (E) Ionic interactions. Courtesy FNH 301 Food Chemistry Land and Food Systems UBC Protein-protein interactions Hydrogen bonds Interaction of a hydrogen atom that is covalently attached to an electronegative atom (N, O, S) with another electronegative atom. Courtesy FNH 301 Food Chemistry Land and Food Systems UBC Protein-protein interactions Hydrophobic interactions Protein stability depends on maintenance of an apolar environment. Hydrophobic interactions are the major forces to drive protein folding and stability. Hydrophobic interaction between nonpolar side chains of amino acid residues is the major reason by which proteins fold into unique tertiary structures. Protein-protein interactions Disulfide bonds Disulfide bonds occur between two cysteine (Cys) residues by oxidation of the sulfhydryl groups by molecular oxygen to form cystine Once formed, disulfide bonds help stabilize the folded structure of protein. iii). Protein denaturation Protein denaturation is known as changes in the secondary, tertiary and quaternary structures of a protein without cleavage of backbone peptide bonds. https://www.google.ca/url?sa=i&url=http%3A%2F%2Fib.bioninja.com.au%2Fstandard-level%2Ftopic-2-molecular-biology%2F24- proteins%2Fdenaturation.html&psig=AOvVaw1H_- FmFS65LFR1hRntzfrZ&ust=1630238004457000&source=images&cd=vfe&ved=2ahUKEwipncH609PyAhWLrJ4KHbZQC2YQr4kDegUIAR DKAQ Protein denaturation Causes of protein denaturation include: Acids Alkaline conditions Strong salts solutions Radiation Heat Protein denaturation is usually reversible (in the absence of aggregation) when the denaturant is removed (except egg white). Thermal denaturation temperatures (Td) and hydrophobicity of protein. Courtesy FNH 301 Food Chemistry Land and Food Systems UBC Changes with protein denaturation Many biologically active proteins lose their activity upon denaturation. Denaturation may result in: a) Loss of solubility b) Increase intrinsic viscosity c) Altered water holding capacity d) Increase digestibility e) Increase susceptibility to protease attack f) Improves foaming and emulsifying properties Changes with protein denaturation Primary structure, such as the sequence of amino acids held together by covalent peptide bonds, is not disrupted by denaturation. In secondary structure denaturation, proteins lose all regular repeating patterns such as α-helices and β-pleated sheets, and adopt a random coil configuration. Changes with protein denaturation .Tertiary structure denaturation involves the disruption of: a) Covalent interactions (such as disulfide bonds) – no breakage. b) Non-covalent interactions between polar amino acid side chains and the surrounding solvent. c) Van der Waals interactions between nonpolar amino acid side chains. In Quaternary structure denaturation, protein sub-units are dissociated and/or the spatial arrangement of protein subunits is disrupted https://foodtechpathshala.com/science-of- protein-denaturation-by-heat/ iv. Functional properties of proteins Functionality of food proteins is defined as “those physical and chemical properties which affect the behavior of proteins in food systems during processing, storage, preparation and consumption”. (Kinsella, 1976). Functional roles of food proteins in food systems are: solubility, viscosity, water binding, gelation, cohesion- adhesion, elasticity, emulsification, foaming, fat and flavor binding. Some functional properties of proteins in food systems Functional properties of proteins M. A. H. Ismond, E. D. Murray, and S. D. Amtfield, The role of non-covalent forces in micelle formation by vicilin from Vicia faba. 11. The effect of stabilizing and destabilizing anions on protein interactions, Food Chem. 21:27 (1986). Functional properties of proteins a). Water binding capacity Water molecules bind to different groups in proteins: Charged groups: ion-dipole interaction Backbone peptide groups Amide groups of Asn and Gln Hydroxyl groups of Ser, Thr, and Tyr residues Non-polar residues: dipole-induce dipole interaction and hydrophobic hydration. Functional properties of proteins a). Water binding capacity Defined as grams of water bound per gram of protein when a dry protein is equilibrated with water vapor at 90- 95% relative humidity. Water binding capacity (WBC) is also known as hydration capacity. Functional properties of proteins Factors affecting water binding capacity (WBC) (1) pH and isoelectric point (pI): At isoelectric point (pI), proteins exhibit the least hydration, but increased protein-protein interaction. WBC increases above and below the isoelectric pH. Functional properties of proteins Factors affecting water binding capacity (2) Presence of Salts: At low concentrations (to surface of oil droplet; HL amino acid side chains remain in aqueous solution. Result: water-oil interface. Protein cannot be used for water in oil (w/o) emulsions because of insolubility in oil. Functional properties of proteins Factors affecting protein emulsification (1) Solubility of protein Optimal at 25-80% solubility. (2) pH Proteins with high solubility have maximal emulsifying capacity. (3) Salt Salts may increase protein solubility thus increasing emulsification. Functional properties of proteins Factors affecting protein emulsification (4) Temperature Small increase in temperature will influence the rate of diffusion and the rate of adsorption and protein unfolding, thus increasing emulsification. (5) Denaturation With partial denaturation, proteins start to unfold – “coat” oil droplet Stabilize emulsion by coating and steric hinderance (proteins act like “spikes”) Functional properties of proteins d). Foaming A foam consists of an aqueous continuous phase and a gaseous (air) dispersed phase. The foaming property of protein refers to its ability to form a thin at gas-liquid interfaces so that large quantities of gas bubbles can be incorporated and stabilized. Protein-stabilized foams are formed by bubbling, whipping, or shaking a protein solution. Functional properties of proteins e). Flavor binding Proteins themselves are odorless. Flavors come mainly from aldehydes, ketones, and alcohols. Noncovalent interactions are normally the mechanism of flavor binding to proteins. Noncovalently bound fraction can contribute to aroma and taste of the protein food. Functional properties of proteins Factors affecting flavor binding (1) Protein denaturation Thermally denatured proteins exhibit increased ability to bind flavors. Ability to bind flavors is dependent on non-polar groups exposed (2) Salts “Salting-in” and “Salting-out” will affect binding – dependent on non-polar groups exposed Functional properties of proteins Factors affecting flavor binding (3) pH Flavor binding is usually enhanced at alkaline pH. (4) Disulfide bonds Breakage of disulfide bonds causes unfolding of proteins thus increasing flavor binding. (5) Proteolysis Proteolysis usually produces peptides of bitter taste exposure of hydrophobic amino acids. Functional properties of proteins f). Gelation Protein gelation refers to transformation of a protein from a solid state to a “gel-like” state. Proteins form two types of gels: coagulum gels and translucent gels. nPN=native nPD=denatured n= number Courtesy FNH 301 Food Chemistry Land and Food Systems UBC Functional properties of proteins Types of protein gels (1) Coagulum gel (opaque gel) Original protein have large amounts of non-polar residues that undergo hydrophobic aggregation, or insoluble aggregates that randomly associate. Irreversible gel. Surimi https://stock.adobe.com/ca/search/images ?k=surimi&asset_id=26286297 Functional properties of proteins Types of protein gels (2) Translucent gel Main linkages are H-bonds and electrostatic forces. Formed by proteins with relatively small amounts of non-polar residues and high amounts of polar amino acid residues. Reversible soluble complex after denaturation, e.g., “jello” Ordered aggregation Functional properties of proteins Types of protein gels Gel network is mainly formed by hydrogen bonding and hydrophobic and electrostatic interactions – more structure Gels are highly hydrated systems, up to 98% water. Translucent gels hold more water and are less prone to syneresis than coagulum-type gels, due to hydrogen bonding interactions. Functional properties of proteins Factors affecting protein gelation (1) Type of interaction Gels formed by noncovalent interactions, mainly hydrogen bonding, are thermally reversible, e.g., “jello”. Gels formed by hydrophobic interactions are irreversible (i.e., egg white gel). Proteins can undergo polymerization via sulfhydryl- disulfide interactions, are usually thermally irreversible (i.e., ovalbumin, β-lactoglobulin, whey gels) Functional properties of proteins Factors affecting protein gelation (2) Protein concentration Minimum protein concentration, least concentration endpoint (LCE) is required. Soy protein = 8%; egg albumin = 3%; gelatin = 0.6%. (3) Humectants (sugars or salts) Humectants bind to free water causing high protein- protein interaction and low protein-water interaction. This results in strong gels formation with greater heat stability. Functional properties of proteins Factors affecting protein gelation (4) pH At or near pI, proteins usually form a coagulum-type gel. Extreme pH, weak gels are formed because of strong electrostatic repulsion. Optimum pH = 7 – 8 for most proteins. Functional properties of proteins Factors affecting protein gelation (5) Proteolysis Limited proteolysis facilitates gel formation. Addition of chymosin (rennin) to casein in milk results in formation of a coagulum-type gel (cheese curds) – hydrophobic interactions. (6) Cross-linking Enzymatic cross-linking at room temperature can result in gelation, e.g., Transglutaminase is used to form highly elastic and irreversible gels, even at low protein concentration. Transglutaminase (TG) Gelatin gel https://chembam.com/resources-for-students/the-chemistry-of/gelatin/ Let’s look at Milk protein Bovine milk contains 30–36 g/L (%) of total protein. Milk proteins: casein (80%) and whey (20%). https://www.researchgate.net/figure/Composition-of-milk-8-9_tbl1_280318425 Casein Mainly α-, β-, and κ- caseins. Calcium sensitive α- and β- caseins, while κ-caseins are calcium insensitive. Caseins are amphipathic, have both polar and hydrophobic residues. In solution, caseins self-associate to form large spherical complexes known as micelles. Casein micelle Various models (below is just one example) - composed of sub-micelles Casein Adding Ca+2 to α- and β- caseins will precipitate the protein. Adding Ca+2 to κ-casein will not affect the protein and it will remain soluble. Rennin (chymosin) enzyme cleaves κ-casein at Phe105 – Met106 into: para-κ-casein and a glycopeptide. para-κ-casein micelles form small aggregates, which then assemble into a gel – hydrophobic interactions. Renneting (chymosin) of Milk – Coagulation (cheese curds) https://www.thecourtyarddairy.co.uk/blog/cheese-musings-and- tips/rennet-in-cheese-the-science-how-rennet-works/ Properties of casein (1) Hydrophobicity αs2- < αs1- < β- (2) Calcium binding capacity The higher degree of phosphorylation, the higher binding capacity to calcium. αs2- > αs1- > β- > κ- (3) Interactions Micelles are kept together by electrostatic interactions: calcium or calcium phosphate bridges with phosphoserine and glutamic residues. Hydrogen bonds and minimal hydrophobic bonding.