Photosynthesis Overview PDF
Document Details
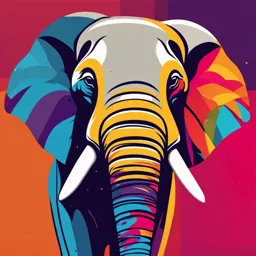
Uploaded by VirtuousAsh
Tags
Summary
This document provides an overview of photosynthesis, covering light-dependent and light-independent reactions, and the roles of photosystems and ATP production. It details the process of converting light energy into chemical energy and the significance of these reactions in the overall process of photosynthesis.
Full Transcript
Photosynthesis Overview: Photosynthesis is a two-step process: Light-dependent reactions: Convert light energy into chemical energy (ATP) and split water to release electrons and hydrogen atoms. Light-independent reactions: Use ATP and NADPH from the light-dependent reactions to fix carbon (CO₂) and...
Photosynthesis Overview: Photosynthesis is a two-step process: Light-dependent reactions: Convert light energy into chemical energy (ATP) and split water to release electrons and hydrogen atoms. Light-independent reactions: Use ATP and NADPH from the light-dependent reactions to fix carbon (CO₂) and synthesize organic molecules. 2. Interdependence of Reactions: The light-dependent reactions produce ATP and NADPH (energy carriers) needed for the light-independent reactions (Calvin cycle). The light-independent reactions require ATP for energy and NADPH to reduce carbon dioxide and form organic molecules. NADP+ (unloaded) is crucial in the light-dependent stage, and it is converted to NADPH in the light-independent stage. Lack of CO₂ in the light-independent reaction can prevent the production of NADPH in Photosystem II. 3. Photosystem Functionality: Photosystems are complexes of pigments (e.g., chlorophyll) that capture light energy and generate high-energy electrons. There are two main photosystems: Photosystem II (PSII): Absorbs light (~680 nm) and splits water, donating excited electrons to the electron transport chain, generating ATP. Photosystem I (PSI): Absorbs light (~700 nm) and passes excited electrons to NADP+, producing NADPH. It can also recycle electrons in cyclic photophosphorylation to generate more ATP. 4. Pigment Structure & Function: Photosynthetic organisms use a variety of pigments (not just chlorophyll) to absorb different wavelengths of light, optimizing energy capture. These pigments are organized into photosystems that maximize light absorption, facilitating efficient energy transfer to the reaction center. 5. Z Scheme (Electron Flow): The Z scheme represents the flow of electrons through the photosystems: Photosystem II: Light energizes electrons, which are then passed through an electron transport chain to produce ATP. Photosystem I: Receives de-energized electrons, energizes them with light, and uses them to reduce NADP+ to NADPH. Summary: - Light-dependent reactions generate ATP and NADPH, which are required by the light-independent reactions (Calvin cycle) to produce organic compounds. - The two photosystems (PSII and PSI) work together to capture light energy, split water, generate ATP, and produce NADPH. - The interdependence of these reactions ensures that both ATP and NADPH are available when needed to convert CO₂ into organic molecules in the light-independent reactions. 1. Thylakoid Structure & Function: The light-dependent reactions occur within thylakoids, which are specialized membrane-bound structures within chloroplasts. In plants, thylakoids are arranged into stacks called grana. In cyanobacteria and single-celled algae, they exist as individual floating thylakoids. Thylakoids house the photosystems, complexes of pigments that absorb light energy and convert it into chemical energy. 2. Role of Photosystems: Photosystems (organized in thylakoid membranes) use light energy to excite electrons in pigments. The energized electrons are passed to an electron transport chain (ETC) in the thylakoid membrane, initiating ATP production and electron transfer processes. 3. ATP Production (Chemiosmosis & Photophosphorylation): As electrons travel through the electron transport chain, they lose energy, which is used to pump protons (H⁺)from the stroma into the thylakoid space, creating a proton gradient. The protons flow back into the stroma through ATP synthase, which uses this flow (chemiosmosis) to produce ATP from ADP and inorganic phosphate (Pi). This ATP production is known as photophosphorylation because light energy is the initial source. 4. Reduction of NADP+ (Non-Cyclic Photophosphorylation): Non-cyclic photophosphorylation involves both Photosystem II (PS II) and Photosystem I (PS I) to generate both ATP and NADPH. In PS II, light energizes electrons, which are passed down the electron transport chain and eventually used to produce ATP. In PS I, light energizes electrons, which are used to reduce NADP+ to NADPH (a coenzyme that carries energy for the light-independent reactions). Water molecules are split (photolysis) in PS II to replace electrons lost during light absorption, making this process non-cyclic. 5. Cyclic Photophosphorylation: Cyclic photophosphorylation involves only Photosystem I (PS I). The electrons are excited and passed through the electron transport chain but are recycled back to PS I rather than being transferred to NADP+. This process generates ATP but does not produce NADPH or contribute to organic molecule synthesis. It serves as a mechanism to balance ATP and NADPH production when needed. Key Processes: Photophosphorylation (ATP synthesis via light energy) can occur in two ways: cyclic (only ATP) and non-cyclic(ATP + NADPH). Chemiosmosis generates ATP through the movement of protons across the thylakoid membrane via ATP synthase. Summary: Thylakoids in chloroplasts are crucial for the light-dependent reactions, where light energy is captured by photosystems and used to produce ATP and NADPH. Non-cyclic photophosphorylation uses both photosystems (PS II and PS I) to generate ATP and NADPH, while cyclic photophosphorylation uses only PS I to produce ATP. The production of ATP via chemiosmosis is a key feature of the thylakoid membrane’s role in photosynthesis. 1. Calvin Cycle Overview: The light-independent reactions (Calvin cycle) occur in the stroma of the chloroplast and use ATP and NADPH from the light-dependent reactions to convert CO₂ into organic molecules. 2. Step 1: Carbon Fixation (Rubisco): The Calvin cycle begins with a 5-carbon compound called ribulose bisphosphate (RuBP). The enzyme Rubisco catalyzes the attachment of CO₂ to RuBP, forming an unstable 6-carbon compound that quickly breaks down into two molecules of glycerate-3-phosphate (GP), each containing 3 carbon atoms. For each cycle, 3 molecules of CO₂ combine with 3 molecules of RuBP, producing 6 molecules of GP. 3. Step 2: Reduction of GP to Triose Phosphate (TP): GP is converted into triose phosphate (TP) using NADPH and ATP, both produced during the light-dependent reactions. NADPH provides electrons (hydrogen atoms), and ATP provides energy for this conversion. Six molecules of GP are converted into six molecules of TP per cycle. 4. Step 3: Regeneration of RuBP: Out of the six TP molecules produced, one TP may be used to form a sugar molecule (such as glucose), but two cycles are required to form one glucose monomer. The remaining five TP molecules are used to regenerate RuBP (3 × 5-carbon molecules from 5 × 3-carbon molecules of TP), which requires energy from the hydrolysis of ATP. 5. Carbon Compounds Produced by the Calvin Cycle: The Calvin cycle ultimately fixes carbon from CO₂ into organic compounds, primarily in the form of triose phosphates (TP). These TP molecules can be used to form a variety of essential organic compounds, including carbohydrates, lipids, proteins, and nucleic acids. The synthesis of these molecules may require the incorporation of mineral nutrients (e.g., nitrogen for proteins, phosphorus for nucleic acids and phospholipids). 6. Linking Carbon Fixation with Organic Molecule Synthesis: The TP produced in the Calvin cycle can be converted into sugars like glucose, which may be stored as starch or used in various metabolic pathways to form proteins, lipids, and nucleic acids. These organic compounds are crucial for the structure, function, and energy storage in the cell. Summary: The Calvin cycle fixes CO₂ into organic molecules using ATP and NADPH. The cycle involves three main steps: carbon fixation (by Rubisco), reduction of GP to TP, and regeneration of RuBP. The triose phosphate (TP) produced can be used to form important biomolecules (carbs, proteins, lipids, nucleic acids) with the help of mineral nutrients. 1. Chloroplast Function: Chloroplasts are the cellular structures responsible for converting light energy into chemical energy during photosynthesis. They produce ATP in the light-dependent reactions and organic compounds (like glucose) in the light-independent reactions (Calvin cycle). 2. Structure and Origin: Chloroplasts are thought to have evolved from prokaryotes (likely cyanobacteria) through a process called endosymbiosis, which is why they have their own DNA (circular, like prokaryotic DNA) and ribosomes (70S, like those in bacteria). They have a double membrane structure due to the process of internalization during endosymbiosis. 3. Thylakoids and Grana: Thylakoids are flattened discs that form the internal membrane system where the light-dependent reactions occur. They have a small internal volume to maximize the proton gradient necessary for ATP synthesis. Thylakoids are organized into stacks called grana to increase the surface area to volume ratio (SA:Vol), enhancing the efficiency of light absorption and energy production. 4. Photosystems and Pigment Arrangement: Within the thylakoid membrane, photosystems are clusters of pigments (like chlorophyll) that absorb light energy. This arrangement maximizes light absorption, crucial for the initial stage of photosynthesis. 5. Stroma and Enzyme Environment: The stroma is the fluid-filled cavity surrounding the thylakoids. It contains the enzymes and has the appropriate pHto support the Calvin cycle, where carbon fixation occurs to form organic compounds. 6. Lamellae: Lamellae are membrane structures that connect and separate the grana stacks of thylakoids. They help organize the thylakoid system, maximizing the efficiency of photosynthesis. Summary: Chloroplasts are specialized for photosynthesis, with structures such as thylakoids (for light reactions), grana (to increase surface area), photosystems (to capture light), and stroma (for the Calvin cycle). Their evolutionary origin as endosymbiotic prokaryotes is reflected in their DNA and ribosomes, which are similar to those of bacteria. These adaptations collectively maximize the efficiency of photosynthesis.