Red Blood Cells, Anemia, and Polycythemia (PDF)
Document Details
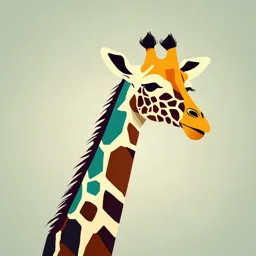
Uploaded by VerifiablePrologue3527
Mary Baldwin University
Tags
Summary
This document provides an overview of red blood cells, including their function, production, and lifespan. It also discusses different types of anemia and their causes. The document is likely a chapter or section from a larger medical textbook.
Full Transcript
G.33 Red Blood Cells, Anemia, and Polycythemia Red Blood Cells (Erythrocytes) The primary function of red blood cells is to transport hemoglobin, which carries oxygen. Red blood cells contain carbonic anhydrase, serving as an excellent acid-base buffer. Normal red blood cell concentrations: Men: 5.2...
G.33 Red Blood Cells, Anemia, and Polycythemia Red Blood Cells (Erythrocytes) The primary function of red blood cells is to transport hemoglobin, which carries oxygen. Red blood cells contain carbonic anhydrase, serving as an excellent acid-base buffer. Normal red blood cell concentrations: Men: 5.2 million per microliter Women: 4.7 million per microliter Hemoglobin concentration maxes out at 34g per 100ml of cells. Normal hemoglobin levels when hematocrit is normal: Men: 15g per 100ml of blood Women: 14g per 100ml of blood When fully saturated, each gram of hemoglobin can bind 1.34ml of oxygen. Red blood cell production: Early embryonic life: Yolk sac Middle trimester: Spleen and lymph nodes Last month of gestation: Bone marrow 5 years and below: Bone marrow of all bones 5-20 years: Marrow of long bones Greater than 20 years: Marrow of membranous bones (vertebrae, sternum, ribs, ilium) All circulating blood cells originate from hematopoietic stem cells in the bone marrow. Growth inducers and differentiation inducers control their life cycle. Interleukin-3 promotes growth and reproduction of all types of committed stem cells. Low oxygen levels cause growth induction and differentiation in red blood cell production. Red blood cell genesis: Pro erythroblast → Reticular site. Reticular sites can pass from bone marrow into blood capillaries and mature into erythrocytes within 1-2 days. Reticular site concentration among red blood cells is normally less than 1%. Red blood cell volume is tightly controlled to ensure sufficient oxygen transport without impeding blood flow. Inadequate oxygen transport and tissue hypoxia stimulate red blood cell production. Erythropoietin is the primary hormone responsible for red blood cell production. 90% is formed in the kidneys, 10% in the liver. Renal hypoxia increases transcription of the erythropoietin gene. Hypoxia in other body parts also stimulates erythropoietin production via norepinephrine, epinephrine, and prostaglandins. Erythropoietin stimulates the production of peripheral blasts from hematopoietic stem cells within minutes to hours of tissue hypoxia. New red blood cells appear in circulation about five days later. With sufficient erythropoietin, iron, and nutrients, red blood cell production can increase to ten times normal. Vitamin B12 and folic acid deficiency impairs DNA, causing failure of nuclear maturation and cell division. Mature red blood cells lack a nucleus, mitochondria, or endoplasmic reticulum. They contain cytoplasmic enzymes for metabolizing glucose and forming ATP. These enzymes maintain cell membrane pliability, membrane transport of ions, and keep iron in the ferrous state. Enzyme activity decreases with cell age, leading to increased fragility. Red blood cell lifespan is 120 days. Many self-destruct in the spleen due to narrow passages (3 micrometers wide). Spleen removal increases the number of old, abnormal red blood cells in circulation. Hemoglobin Hemoglobin A is the most common type in adults, consisting of four hemoglobin chains. Each chain has a heme group containing one iron atom that can bind one oxygen molecule. Variations in amino acid composition can alter the physical characteristics and oxygen-carrying capacity of hemoglobin chains. Hemoglobin binds loosely and reversibly with oxygen. Iron is crucial for oxygen transport, with the body containing an average of 4-5g. It is absorbed in the small intestine, and excess iron is stored in the liver hepatocytes. When red blood cells are destroyed, iron is stored in the ferritin pool for reuse. Men lose about 0.6mg of iron daily in feces. Women lose about 1.3mg daily, accounting for menstruation. Intestinal absorption of iron is low, with only a few milligrams absorbed daily, decreasing further when the body is saturated with iron. Anemia Anemia is categorized into four types: blood loss anemia, aplastic anemia, megaloblastic anemia, and hemolytic anemia. Blood Loss Anemia: Rapid blood loss: Plasma volume is replaced in 1-3 days, but red blood cell concentration takes 3-6 weeks to return to normal. Chronic blood loss: Results in microcytic hypochromic anemia due to insufficient iron absorption. Aplastic Anemia: Caused by bone marrow dysfunction. Potential causes: High-dose radiation, chemotherapy, toxic chemicals (e.g., insecticides, benzene), autoimmune disorders (e.g., lupus). Idiopathic aplastic anemia occurs in about 50% of cases. Megaloblastic Anemia: Red blood cells grow large with odd shapes (megaloblasts). Caused by deficiency of vitamin B12, folic acid, or intrinsic factor from the stomach mucosa. Megaloblastic cells rupture easily, leading to anemia. Pernicious anemia results from a failure to produce normal gastric secretions [2, 3]. Parietal cells secrete intrinsic factor, which binds to vitamin B12 to protect it from digestion and facilitate absorption in the ileum. Lack of intrinsic factor reduces B12 availability. Hemolytic Anemia: Red blood cells are fragile and rupture easily, especially in the spleen. Red blood cell production may be normal or higher, but lifespan is significantly reduced. Types include hereditary spherocytosis, sickle cell anemia, and erythroblastosis fetalis. Hereditary spherocytosis: Red blood cells are small and spherical, making them unable to withstand compressive forces in the spleen. Sickle cell anemia: Faulty beta chains in the hemoglobin molecule cause precipitation into long crystals inside red blood cells when exposed to low oxygen concentrations. This makes the cells fragile. Low oxygen tension leads to sickling, causing rupture and further decreasing oxygen tension, creating a progressive cycle. Erythroblastosis fetalis: RH-positive fetal red blood cells are attacked by antibodies from an RH-negative mother, causing them to become fragile and rupture, leading to anemia in the newborn. Circulatory Effects of Anemia: Reduced blood viscosity (as low as 1.5 times that of water, compared to the normal three times). Decreased resistance, leading to increased blood flow. Increased cardiac output. Hypoxia-induced dilation of peripheral blood vessels further increases cardiac output and workload on the heart. Increased cardiac output may compensate for reduced oxygen-carrying capacity, but further increases in demand can lead to cardiac failure. Polycythemia Secondary polycythemia occurs when tissues become hypoxic, leading to increased red blood cell production. This can be caused by high altitudes or failed oxygen delivery, such as in cardiac failure. Polycythemia vera is caused by a genetic aberration in hemoblastic cells, resulting in uncontrolled red blood cell production. Total blood volume increases to almost twice normal. Blood viscosity increases to almost ten times that of water. Individuals typically have a ruby complexion due to increased blood in the skin venous plexus, with a bluish tint caused by cyanosis of the hemoglobin. Sluggish blood flow leads to increased deoxygenation of hemoglobin, causing cyanosis. Circulatory Effects of Polycythemia: Sluggish blood flow in peripheral blood vessels reduces venous return to the heart. Increased blood volume increases venous return. Cardiac output is nearly normal due to the balance of these effects. Compensatory mechanisms remain intact, with only one-third of individuals experiencing hypertension. G.36 Blood Types and Transfusions Antigenicity and Immune Reactions: Blood transfusions can lead to agglutination and hemolysis of red blood cells (RBCs) if the blood types of the donor and recipient are not compatible. This is due to the presence of antigens on the surface of RBCs that can react with antibodies in the plasma of another blood type. O-A-B Blood Types: The O-A-B system is one of the two main types of antigens that can cause transfusion reactions. Antigens (Agglutinogens): Type A and type B antigens are found on the surfaces of RBCs. These antigens, also called agglutinogens, can cause RBC agglutination. People may have neither, one, or both of these antigens. Agglutinogens and Blood Types: The presence or absence of A and B agglutinogens determines blood type. ○ Type O: Neither A nor B agglutinogen is present. ○ Type A: Only A agglutinogen is present. ○ Type B: Only B agglutinogen is present. ○ Type AB: Both A and B agglutinogens are present. Genetic Determination: The ABO blood group genetic locus has three alleles: IA, IB, and IO (commonly referred to as A, B, and O). ○ The O allele is functionless, resulting in no significant type O agglutinogen on the cells. ○ The A and B alleles cause strong agglutinogens on the cells. ○ The O allele is recessive, while A and B alleles are co-dominant. Genotypes: There are six possible combinations of alleles: OO, OA, OB, AA, BB, and AB. ○ Genotype OO: Blood type O (no agglutinogens). ○ Genotype OA or AA: Blood type A (A agglutinogens). ○ Genotype OB or BB: Blood type B (B agglutinogens). ○ Genotype AB: Blood type AB (A and B agglutinogens). Agglutinins: When type A agglutinogen is not present, anti-A agglutinins develop in the plasma. When type B agglutinogen is not present, anti-B agglutinins develop. ○ Type O blood: Contains both anti-A and anti-B agglutinins. ○ Type A blood: Contains type A agglutinogens and anti-B agglutinins. ○ Type B blood: Contains type B agglutinogens and anti-A agglutinins. ○ Type AB blood: Contains both A and B agglutinogens but no agglutinins. Agglutination Process: Mismatched bloods result in anti-A or anti-B plasma agglutinins mixing with RBCs containing A or B agglutinogens, causing agglutination. Agglutinins bind to two or more RBCs, clumping the cells together. These clumps plug small blood vessels. Physical distortion or phagocytic white blood cells destroy the membranes of agglutinated cells, causing hemolysis. Origin of Agglutinins: Agglutinins are gamma globulins produced by bone marrow and lymph gland cells. Rh Blood Types: The Rh blood type system is also important in transfusions. Rh Antigens: There are six common types of Rh antigens (C, D, E, c, d, and e). Rh Positive/Negative: The presence of the type D antigen determines if a person is Rh positive; its absence means the person is Rh negative. Rh Immune Response: Unlike the O-A-B system, spontaneous agglutinins almost never occur in the Rh system. A person must be exposed to the Rh antigen to develop agglutinins. Formation of Anti-Rh Agglutinins: Rh-negative individuals develop anti-Rh agglutinins upon exposure to Rh-positive blood. Characteristics of Rh Transfusion Reactions: A first transfusion of Rh-positive blood into an Rh-negative person may not cause an immediate reaction, but subsequent transfusions can cause severe reactions. Blood Typing: Blood typing is necessary to match donor and recipient blood before a transfusion. RBCs are separated from plasma and mixed with anti-A and anti-B agglutinins. Agglutination indicates an antibody-antigen reaction. Type O RBCs do not react with anti-A or anti-B agglutinins, while Type A, B, and AB blood will agglutinate with the corresponding antisera [5, 7]. Transfusion Reactions: Transfusion reactions occur when mismatched blood types are mixed, leading to agglutination and hemolysis of RBCs [3, 8]. Acute Hemolysis: Immediate hemolysis of RBCs can occur due to mismatched blood, where antibodies cause lysis by activating the complement system. Effects of Transfusion Reactions: Reactions can cause immediate or delayed hemolysis, resulting in the release of hemoglobin into the plasma and potential kidney failure. Acute Kidney Failure: Kidney failure can result from renal vasoconstriction, circulatory shock, and renal tubular blockage caused by hemoglobin precipitation. Erythroblastosis Fetalis (Hemolytic Disease of the Newborn): This condition occurs when the mother is Rh negative and the fetus is Rh positive. The mother develops anti-Rh agglutinins that cross the placenta and attack the fetus's RBCs. Incidence: First pregnancies usually do not cause harm, but subsequent pregnancies can lead to the disease. Effects on the Fetus: Agglutination and hemolysis of fetal RBCs, leading to anemia, jaundice, and potential brain damage (kernicterus) [6, 8]. Treatment: Replacing the neonate's blood with Rh-negative blood can help prevent kernicterus. Prevention: Rh immunoglobulin can be administered to the mother to prevent sensitization to the D antigen. Transplantation of Tissues and Organs: Foreign cells transplanted into a recipient can produce an immune reaction. Graft Types: ○ Autograft: Transplant from one part of the body to another in the same individual. ○ Isograft: Transplant between identical twins. ○ Allograft: Transplant between individuals of the same species. ○ Xenograft: Transplant from one species to another. Tissue Typing: Matching human leukocyte antigens (HLA) is crucial for graft acceptance. Prevention of Graft Rejection: Immunosuppression: Suppressing the immune system can prevent graft rejection. Therapeutic Agents: Glucocorticoid hormones, azathioprine, cyclosporine, tacrolimus, and antilymphocyte antibodies [12, 13]. G.37 Hemostasis and Blood Coagulation: A Comprehensive Overview Detailed Note on Hemostasis and Blood Coagulation Hemostasis, the prevention of blood loss, is achieved through several mechanisms. These include vascular constriction, platelet plug formation, blood clot formation via coagulation, and eventual fibrous tissue growth into the clot to permanently close the vessel. Vascular Constriction When a blood vessel is severed or ruptured, the vessel wall undergoes smooth muscle contraction, reducing blood flow. This contraction results from local myogenic spasm, local autacoid factors from traumatized tissues, vascular endothelium, and blood platelets, as well as nervous reflexes initiated by pain or sensory impulses from the traumatized vessel or nearby tissues. Myogenic contraction is likely caused by direct damage to the vascular wall. Smaller vessels constrict due to thromboxane A2 released by platelets. The severity of trauma correlates with the degree of vascular spasm, which can last for minutes or hours, allowing platelet plugging and blood coagulation to occur. Platelet Plug Formation Small cuts in blood vessels are often sealed by a platelet plug. Platelets, or thrombocytes, are small discs without nuclei, with a normal concentration of 150,000 to 450,000/µl in the blood. Platelet Characteristics: Platelets contain actin and myosin for contraction, endoplasmic reticulum and Golgi apparatus residuals for enzyme synthesis and calcium storage, mitochondria and enzyme systems for ATP and ADP formation, enzyme systems for prostaglandin synthesis, fibrin-stabilizing factor, and a growth factor for vascular endothelial cells. Platelet membranes have glycoproteins that prevent adherence to normal endothelium but promote adherence to injured areas, especially exposed collagen. They also contain phospholipids that activate blood-clotting stages. Platelet Activation: Upon contact with a damaged vascular surface, especially collagen fibers, platelets swell, develop pseudopods, contract, and release granules containing active factors, becoming sticky and adhering to collagen and von Willebrand factor (vWF). Platelet Secretions: Platelets secrete ADP and platelet-activating factor (PAF), and their enzymes form thromboxane A2, which activate nearby platelets. This leads to increased platelet stickiness and the formation of a platelet plug. Platelet Plug Stability: The initial plug is loose but is reinforced by fibrin threads during blood coagulation. The platelet-plugging mechanism is crucial for closing minute ruptures in small blood vessels. A deficiency in platelets can lead to numerous small hemorrhagic areas under the skin, known as petechiae. Blood Coagulation Blood clot formation is the third hemostatic mechanism. It begins within 15 to 20 seconds if the vascular trauma is severe or within 1 to 2 minutes if minor. Activator substances from the traumatized vascular wall, platelets, and blood proteins initiate the process. The process involves a cascade of chemical reactions involving over 12 blood coagulation factors, resulting in the formation of prothrombin activator. Prothrombin activator catalyzes the conversion of prothrombin into thrombin. Thrombin then acts as an enzyme to convert fibrinogen into fibrin fibers, which enmesh platelets, blood cells, and plasma to form the clot. Clotting Factors: More than 50 substances affect blood coagulation, including procoagulants and anticoagulants. Clotting depends on the balance between these. Prothrombin to Thrombin: Prothrombin activator forms due to blood vessel rupture or damage to blood substances. In the presence of calcium ions, prothrombin activator converts prothrombin to thrombin. Fibrinogen to Fibrin: Thrombin polymerizes fibrinogen into fibrin fibers. The rate-limiting factor in blood coagulation is usually the formation of prothrombin activator. Conversion of Fibrinogen to Fibrin Fibrinogen, a high-molecular-weight protein (≈340,000), is produced in the liver at a concentration of 100 to 700 mg/dl. Liver disease can reduce fibrinogen concentration. Thrombin acts on fibrinogen to form fibrin monomer molecules, which polymerize into long fibrin fibers. Fibrin stabilizing factor, activated by thrombin, forms covalent bonds between fibrin monomer molecules and cross-linkages between adjacent fibrin fibers, strengthening the fibrin meshwork. The blood clot consists of fibrin fibers, blood cells, platelets, and plasma, adhering to damaged vessel surfaces. Clot Retraction and Serum Expression Clot retraction occurs within minutes after clot formation, expressing fluid called serum. Serum lacks fibrinogen and most clotting factors. Platelets attach to fibrin fibers and release procoagulant substances, including fibrin stabilizing factor. Platelet thrombosthenin, actin, and myosin molecules cause the platelet spicules attached to fibrin to contract, compressing the meshwork. This contraction pulls the edges of the broken vessel together. Positive Feedback of Clot Formation Thrombin's proteolytic action promotes further clotting by acting on other blood-clotting factors and prothrombin. This positive feedback accelerates the actions of factors VIII, IX, X, XI, and XII, and aggregates platelets. Initiation of Coagulation: Formation of Prothrombin Activator Prothrombin activator formation is initiated by trauma to the vascular wall and adjacent tissues, trauma to the blood, or contact of blood with damaged endothelial cells or collagen. This leads to prothrombin conversion to thrombin. Extrinsic Pathway: Triggered by trauma to the vascular wall or extravascular tissues. ◦Release of tissue factor: Traumatized tissue releases tissue factor (phospholipids and lipoprotein complex). ◦Activation of factor X: Tissue factor complexes with factor VII and calcium ions to activate factor X (Xa). ◦Prothrombin activator formation: Activated factor X combines with tissue phospholipids and factor V to form prothrombin activator. Intrinsic Pathway: Triggered by trauma to the blood or exposure to collagen. ◦Activation of factor XII and release of platelet phospholipids: Blood trauma activates factor XII and releases platelet phospholipids (platelet factor 3). ◦Activation of factor XI: Activated factor XII enzymatically activates factor XI, requiring high-molecular-weight kininogen and prekallikrein. ◦Activation of factor IX: Activated factor XI activates factor IX. ◦Activation of factor X: Activated factor IX, with activated factor VIII and platelet phospholipids, activates factor X. ◦Prothrombin activator formation: Activated factor X combines with factor V and platelet phospholipids to form prothrombin activator. Calcium ions are required for most blood-clotting reactions, except the first two steps in the intrinsic pathway. Intravascular Anticoagulants These prevent blood clotting in the normal vascular system. Endothelial Surface Factors: Smoothness of the endothelial cell surface, glycocalyx layer, and thrombomodulin. Thrombomodulin: Binds thrombin, slowing clotting and activating protein C, which inactivates factors V and VIII. Damaged Endothelial Wall: Loss of smoothness and glycocalyx-thrombomodulin layer activates factor XII and platelets. Intact Endothelial Cells: Produce prostacyclin and nitric oxide (NO) that inhibit platelet aggregation. Antithrombin Action: Fibrin fibers adsorb thrombin, preventing its spread. Antithrombin III combines with thrombin, blocking its effect on fibrinogen and inactivating it. Heparin: Combines with antithrombin III, enhancing its effectiveness in removing thrombin. It also removes activated factors IX through XII. Plasmin and Lysis of Blood Clots Plasminogen in plasma is activated to plasmin (fibrinolysin), which digests fibrin fibers and other protein coagulants. Activation of Plasminogen: Tissue plasminogen activator (t-PA) is released by injured tissues and vascular endothelium, activating plasminogen to plasmin. Conditions Causing Excessive Bleeding Excessive bleeding can result from a deficiency of blood-clotting factors. Vitamin K Deficiency: Decreased prothrombin, factor VII, factor IX, and factor X. Vitamin K is needed for liver carboxylation of glutamic acid residues on clotting factors. Deficiency can result from liver disease or poor fat absorption. Hemophilia: Almost exclusively in males. Hemophilia A (classic) is caused by factor VIII deficiency, while hemophilia B is caused by factor IX deficiency. Thrombocytopenia: Low platelet count, leading to small punctate hemorrhages (petechiae). Causes include decreased production, peripheral destruction, sequestration in the spleen, consumption in thrombi, and dilution from fluid resuscitation. Thromboembolic Conditions Thrombi and Emboli: An abnormal clot in a vessel is a thrombus, and a freely flowing clot is an embolus. Emboli can plug arteries in the brain, kidneys, or lungs. Causes: Roughened endothelial surface or slow blood flow. Tissue Plasminogen Activator (t-PA): Can dissolve intravascular clots if delivered promptly. Femoral Venous Thrombosis and Massive Pulmonary Embolism: Immobility and blood stasis can cause clots in leg veins, leading to pulmonary embolism. Disseminated Intravascular Coagulation (DIC): Widespread activation of clotting mechanisms, often due to large amounts of traumatized tissue or septicemia. Anticoagulants for Clinical Use Heparin: Increases blood-clotting time. Coumarins (e.g., Warfarin): Inhibit vitamin K epoxide reductase complex 1 (VKORC1), reducing active vitamin K and decreasing the production of active coagulation factors. Prevention of Blood Coagulation Outside the Body Silicone-coated Containers: Prevent contact activation of platelets and factor XII. Calcium Ion Reduction: Oxalate or citrate compounds can be used to precipitate or deionize calcium, preventing coagulation. Blood Coagulation Tests Bleeding Time: Indicates how long bleeding lasts after a puncture. Prolonged by clotting factor deficiencies or lack of platelets. Clotting Time: Time for blood to clot in a glass tube. Varies widely and is less commonly used. Prothrombin Time: Indicates prothrombin concentration. International Normalized Ratio (INR): Standardizes prothrombin time measurements.