Sensory Information and Neurodevelopment PDF
Document Details
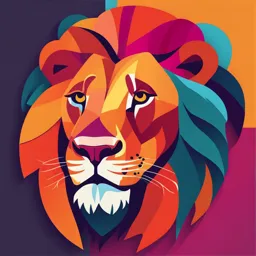
Uploaded by SofterPointillism
Tags
Summary
This document provides an overview of sensory information processing in the brain, covering visual, auditory, and somatosensory systems. It explores the functions of different cortical areas such as the primary visual cortex, Wernicke's area, and the somatosensory cortex. Also discussed are mirror neurons, glial cells plus the five phases of early neurodevelopment.
Full Transcript
Couple of sub regions that process sensory information Visual Information Primary Visual Cortex (V1) Located in the occipital lobe, posterior brain region. First cortical area to process visual stimuli received from the retina via the later...
Couple of sub regions that process sensory information Visual Information Primary Visual Cortex (V1) Located in the occipital lobe, posterior brain region. First cortical area to process visual stimuli received from the retina via the lateral geniculate nucleus (LGN) of the thalamus. (Hubel & Wiesel, 1968): ○ Identified a critical period during early life, essential for normal visual development. ○ Demonstrated that visual deprivation during this period permanently impairs neural development in V1. ○ Influenced clinical practice; congenital cataracts are now treated surgically in infancy to avoid lifelong visual impairment. Visual Association Areas (V2, V3, V4, V5/MT) Further process visual information, specialising in: ○ Colour (V4) ○ Form and depth perception (V2, V3) ○ Motion detection (V5/MT) (Foss-Feig et al., 2013): ○ Deficits in area MT/V5 linked to abnormal motion perception in autism and schizophrenia. ○ Impaired motion processing in MT may contribute to difficulties interpreting biological motion, affecting social interactions. Auditory Information Primary Auditory Cortex (A1) Located in the superior temporal gyrus. Processes basic auditory signals, including pitch and loudness. Auditory Association Cortex (Wernicke’s Area) Located in the posterior section of the superior temporal gyrus, typically in the left hemisphere. Interprets complex sounds, speech comprehension, and semantic meaning. Named after neurologist Carl Wernicke (1874), who discovered its crucial role in language comprehension. Somatosensory Information Primary Somatosensory Cortex (S1) Processes essential tactile sensations: ○ Touch: Pressure, vibration, texture, fine detail. ○ Proprioception: Body position, limb orientation, and movement awareness. ○ Pain and Temperature: Detection and interpretation of noxious (harmful) stimuli and thermal sensations. Somatosensory Association Cortex (Posterior Parietal Cortex) Integrates sensory inputs from S1 to create meaningful perceptions. Key functions include: ○ Stereognosis: Ability to identify objects by touch alone. ○ Spatial Awareness: Recognising body position relative to the environment. ○ Multisensory Integration: Coordinating visual, auditory, and tactile inputs for comprehensive spatial understanding. (Vallar & Bolognini, 2014): ○ Damage (e.g., stroke, trauma) can cause neglect syndrome, leading patients to ignore sensory information on one side of their body or environment. Mirror neurons Mirror neurons are specialised neurons initially discovered in the premotor cortex and inferior parietal lobule of monkeys (Rizzolatti et al., 1996). They activate both when an individual performs an action and when they observe someone else performing the same or a similar action. Some researchers suggest that mirror neurons may have supported the evolution of language by providing a bridge from gestural communication to spoken language, due to their involvement in Broca’s area (Rizzolatti & Arbib, 1998). The mirror neuron system has also been associated with emotional mirroring. Observing others in pain activates affective brain regions such as the anterior insula and anterior cingulate cortex (Singer et al., 2004). There are two main types of mirror neurons: Strictly congruent – respond only to specific, matching actions. Broadly congruent – respond to a range of goal-related actions. Heyes (2010): Argues that mirror neurons may not be innate. Suggests they are formed through sensorimotor associative learning. Supports a learning-based view. Liszka & Saxe (2009): Explain the methodological limitations of studying mirror neurons in humans (e.g., lack of single-neuron recordings). Note that theory of mind abilities are preserved even when mirror neuron regions are damaged. five phases of early neurodevelopment 1️⃣Induction of the Neural Plate Begins around week 3 of gestation. The ectoderm thickens to form the neural plate, a precursor to the nervous system, due to signals from the underlying mesodermal organiser. This leads to formation of the neural groove and eventually the neural tube, which will become the brain and spinal cord. Defects in this stage can result in neural tube defects like spina bifida. Cowan (1979) Outlines how mesodermal signals create neural development in the ectoderm. He introduced the idea of the organiser region as crucial in directing neural fate. 2️⃣Neural Proliferation Once the neural tube is closed, neural stem cells begin to divide rapidly, especially in the ventricular zone. This proliferation is asymmetrical, meaning daughter cells become either new stem cells or differentiate into neurons/glia. 🧾 Götz & Huttner (2005) Established that radial glial cells are not just support structures, they are actually stem cells. These cells can turn into many different types of brain cells, not just help other cells move. 3️⃣Migration and Aggregation New neurons migrate to their final destinations via: Radial migration (outward from ventricular zone) Tangential migration (parallel to tube wall) Migration is guided by: Somal translocation: neuron pulls itself along its process Radial-glia-mediated migration: neuron climbs the glial scaffold Aggregation forms layered brain structures, driven by: Cell adhesion molecules (CAMs) Gap junctions Neuron-glia interactions 🧾 Hatten (2002) Hatten expanded on how radial glial cells act as both guides and signals; they also send out chemical signals that help control how and where those neurons move. 4️⃣Axon Growth and Synapse Formation Axons reach targets using: Chemoaffinity Hypothesis (Sperry, 1963): each target cell releases specific chemical cues that attract the correct axon. Topographic Gradient Hypothesis: position-based chemical gradients guide axons to precise regions. Synaptogenesis: requires coordinated activity between neurons, and recent evidence shows astrocytes play a key regulatory role. Sperry (1963) In his most famous experiment, he surgically removed a frog’s eye, rotated it 180 degrees, and then reattached the optic nerve. Even after healing, the frog saw everything upside down and backwards. Shows that the optic nerve fibres grew back to their original locations in the brain. 🧾 Sanes & Yamagata (2009) – Reviewed molules like cadherins, ephrins, and neurexins, which fine-tune synaptic targeting and specificity, particularly in brain regions like the retina and cerebellum. This review showed that brain wiring is not just about growing toward a target—it’s also about recognising and selecting the right synaptic partner. These molecular cues are vital for building accurate and efficient neural networks during development. 5️⃣Neuron Death and Synapse Rearrangement Apoptosis (programmed cell death) occurs in waves during development to refine neural circuits. Cells that fail to receive neurotrophic support (e.g., NGF, BDNF) undergo apoptosis. Debris is cleared by microglia, preventing inflammation. Neuroplasticity Degeneration: Clearing the way for reorganisation Degeneration is the breakdown of neural connections after injury. Transneuronal degeneration spreads damage to connected neurons. Degeneration is not passive — it’s regulated and can influence recovery (Coleman & Perry, 2002). This pruning removes damaged or unnecessary connections, making space for future rewiring. Coleman & Perry (2002) In normal mice, axons degenerate rapidly after being cut. But in Wld^s mutant mice, axons survive much longer after injury. == gene mutation can slow or block degeneration, proving it's an active biological process, not inevitable. Regeneration: Limited potential, mostly in the PNS Schwann cells in the PNS guide axons and secrete growth factors. Collateral sprouting: healthy neurons send out new axon branches to replace lost connections Navarro et al. (2007) - why CNS can not regenerate Oligodendrocytes, which do not support regeneration. Myelin-associated inhibitors (e.g. Nogo-A, MAG, OMgp) that block axonal growth. Formation of glial scars after injury, which physically and chemically inhibit regrowth. Reorganisation: The brain reroutes itself The cortex can remap functions to undamaged areas: Mechanisms include: ○ Unmasking of latent synapses ○ Synaptogenesis (new connections form) ○ Strengthening of underused pathways Elbert et al. (1995) - Violin players vs non-instrument playing people Used magnetoencephalography (MEG) In musicians, the representation of the left-hand fingers was significantly larger than in non-musicians. The amount of cortical expansion correlated with the age at which musical training began — the earlier the start, the larger the effect. Recovery: Adapting function over time ○ Restitution: reactivation of damaged circuits ○ Compensation: use of alternative strategies Short-term improvements may reflect reduced inflammation (oedema). (Burns et al., 2014) -BCI Stimulates the brain in order to promote neuroplasticity, aiding cortical reorganisation used for several other conditions that cause asynchrony with brain signals, such as Alzheimer's and ADHD.. Damage to the Somatosensory System How damage can occur Ischemic Stroke Definition & Cause: Caused by an obstruction in cerebral blood flow, often due to a thrombus or embolus. Accounts for ~87% of all strokes (Benjamin et al., 2018). Biological Damage Mechanisms: Neuronal Ischemia: Deprivation of oxygen and glucose leads to ATP failure → neuronal depolarization → glutamate excitotoxicity → intracellular calcium overload → cell death (Dirnagl et al., 1999). Hemorrhagic Stroke Definition & Cause: ○ Occurs due to rupture of a cerebral blood vessel → intracerebral (within brain tissue) or subarachnoid (around the brain) bleeding. ○ Commonly caused by hypertension, aneurysms, or arteriovenous malformations. Global Cerebral Hypoxia Energy Depletion: Neurons depend on aerobic metabolism; oxygen deprivation → ATP depletion → ion pump failure → neuronal depolarisation (Choi, 1990).. Glial Cells: Glial cells are non-neuronal support cells found throughout the nervous system. Oligodendrocytes (CNS): Function: Form myelin sheaths around CNS axons to increase conduction velocity and support neuronal metabolism. Maintain long-term axonal health and energy supply. Damage Effects: Associated with diseases like multiple sclerosis, where impaired conduction affects sensory processing (Nave & Werner, 2014). Schwann Cells (PNS): Function: Myelinate peripheral axons, ensuring fast signal conduction. Promote axonal regeneration and guide reinnervation following injury. Damage Effects: Schwann cell abnormalities have been linked to conditions like Charcot-Marie-Tooth disease (Murakami & Sunda , 2019). Microglia (CNS): Function: Serve as the brain’s resident immune cells, detecting damage or pathogens. Regulate inflammation, synaptic pruning, and neuroplasticity. Damage Effects: - Has been linked to alzheimer's There is mounting evidence that microglia protect against the incidence of AD, as impaired microglial activities and altered microglial responses to β-amyloid are associated with increased AD risk.(Hansen et al., 2018) Smith et al. (2018) Impact of genetic mutations on glial cells and their role in altering pain perception, particularly in neuropathic conditions. Glial cells, such as astrocytes and microglia, are crucial for maintaining neural health and function. The research highlighted that certain genetic mutations affecting these glial cells can lead to dysregulated immune responses and heightened sensitization of pain pathways in the nervous system.