Unit 3: Semiconducting Devices PDF
Document Details
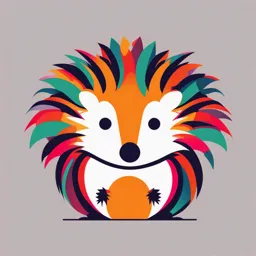
Uploaded by FastestClematis3795
KIET Group of Institutions
Tags
Summary
This document provides an overview of semiconducting devices, focusing on solar cells. It details the photovoltaic effect, construction, working principles, and efficiency of solar cells. It also discusses the materials used, advantages, and disadvantages.
Full Transcript
Unit 3: Semiconducting Devices Solar cell: A solar cell (also known as a photovoltaic cell or PV cell) is defined as an electrical device that converts light energy into electrical energy through the photovoltaic effect. A solar cell is basically a p-n junction diode and are a form of photoelectric...
Unit 3: Semiconducting Devices Solar cell: A solar cell (also known as a photovoltaic cell or PV cell) is defined as an electrical device that converts light energy into electrical energy through the photovoltaic effect. A solar cell is basically a p-n junction diode and are a form of photoelectric cell, defined as a device whose electrical characteristics – such as current, voltage, or resistance – vary when exposed to light. Individual solar cells can be combined to form modules commonly known as solar panels. The common single junction silicon solar cell can produce a maximum ma open-circuit circuit voltage of approximately 0.5 to 0.6 volts. When combined into a large solar panel, considerable amounts of renewable energy can be generated. Photovoltaic effect: The effect due to which light energy is converted to electric energy in certain semiconductor materials is known as photovoltaic effect. This directly converts light energy to electricity without any intermediate process. Construction of Solar Cell: A solar cell functions similarly to a junction diode, but its construction differs slightly from typical p-n junction diodes. A very thin layer of p-type semiconductor is grown on a thicker n- type semiconductor. Then a few finer electrodes on the top of the p-type semiconductor layer applied. Just below the p-type layer there is a p-n junction. A current collecting electrode is applied at the bottom of the n-type layer. The entire assembly is encapsulated by thin glass to protect the solar cell from any mechanical shock. Working Principle of Solar Cell: When light photons reach the p-n junction through the thin p-type layer, they supply enough energy to create multiple electron-hole pairs, initiating the conversion process. The incident light breaks the thermal equilibrium condition of the junction. The free electrons in the depletion region can quickly come to the n-type side of the junction. Similarly, the holes in the depletion can quickly come to the p-type side of the junction. Once, the newly created free electrons come to the n-type side, cannot further cross the junction because of barrier potential of the junction. Once the newly created holes reach the p-type side, they cannot cross back over the junction due to the barrier potential. This separation of electrons and holes across the p-n junction allows it to function like a small battery cell. A voltage is set up which is known as photo voltage. If we connect a small load across the junction, there will be a tiny current flowing through it. I-V Characteristics of a Solar Cell: Solar Cell Efficiency: The efficiency is the most commonly used parameter to compare the performance of one solar cell to another. Efficiency is defined as the ratio of energy output from the solar cell to input energy from the sun. In addition to reflecting the performance of the solar cell itself, the efficiency depends on the spectrum and intensity of the incident sunlight and the temperature of the solar cell. The efficiency of a solar cell is determined as the fraction of incident power which is converted to electricity and is defined as: = = Where: Voc is the open-circuit voltage; is the short-circuit current; FF is the fill factor and η is the efficiency. Fill Factor: The short-circuit current and the open-circuit voltage are the maximum current and voltage respectively from a solar cell. However, at both of these operating points, the power from the solar cell is zero. The "fill factor", more commonly known by its abbreviation "FF", is a parameter which, in conjunction with Voc and Isc, determines the maximum power from a solar cell. The FF is defined as the ratio of the maximum power from the solar cell to the product of Voc and Isc so that: = × Materials Used in Solar Cell: Materials used in solar cells must possess a band gap close to 1.5 ev to optimize light absorption and electrical efficiency. Commonly used materials are: Silicon, GaAs, CdTe, CuInSe2. Criteria for materials to be used in solar cell: 1. Must have band gap from 1ev to 1.8ev. 2. It must have high optical absorption. 3. It must have high electrical conductivity. 4. The raw material must be available in abundance and the cost of the material must be low. Advantages of Solar Cell 1. No pollution associated with it. 2. It must last for a long time. 3. No maintenance cost. Disadvantages of Solar Cell 1. It has high cost of installation. 2. It has low efficiency. 3. During cloudy day, the energy cannot be produced and also at night we will not get solar energy. Applications of solar cells: Solar cells power devices from small calculators and wristwatches to large-scale applications in spacecraft, highlighting their versatility and growing importance in renewable energy systems. Photodetectors Photodetectors are devices used for the detection of light. Photodetectors usually deliver an electronic output signal – for example, a voltage or electric current which is proportional to the incident optical power, thus they belongs to the area of optoelectronics. Types of Photodetectors: There are many types of photodetectors described as below: Photodiodes are semiconductor devices with a p–n junction or p–i–n structure (i = intrinsic material) (→ p–i–n photodiodes), where light is absorbed in a depletion region and generates a photocurrent. Such devices can be very compact, fast, highly linear, and exhibit a high quantum efficiency (i.e., generate nearly one electron per incident photon) and a high dynamic range, provided that they are operated in combination with suitable electronics. A particularly sensitive type is that of avalanche photodiodes, which are sometimes used even for photon counting. Metal–semiconductor–metal (MSM) photodetectors contain two Schottky contacts instead of a p–n junction. They are potentially faster than photodiodes, with bandwidths up to hundreds of GHz Phototransistors are similar to photodiodes, but exploit internal amplification of the photocurrent. Photoconductive detectors are based on certain semiconductors, e.g. cadmium sulfide (CdS). They are cheaper than photodiodes, but they are fairly slow, are not very sensitive, and exhibit a nonlinear response. Phototubes are vacuum tubes or gas-filled tubes where the photoelectric effect is exploited (→ photoemissive detectors). Photomultipliers are a special kind of phototubes, exploiting electron multiplication processes for obtaining a much increased responsivity. They can also have a high speed and large active area. Some of them are based on multichannel plates; they can be substantially more compact than traditional photomultipliers. Research is performed on novel photodetectors based on carbon nanotubes (CNT) or graphene, which can offer a very broad wavelength range and a very fast response. Ways for integrating such devices into optoelectronic chips are explored. These devices are all based on the internal or external photoelectric effect; photoemissive detectors belong to the latter category. Various kinds of photodetectors can be integrated into devices like power meters and optical power monitors. Others can be made in the form of large two-dimensional arrays, e.g. for imaging applications. Construction and working of photodiode and PIN diode: Photodiodes are frequently used photodetectors, which have largely replaced the formerly used vacuum phototubes. They are semiconductor devices which contain a p–n junction, and often an intrinsic (undoped) layer between n and p layers. Devices with an intrinsic layer are called p–i–n or PIN photodiodes. Light absorbed in the depletion region or the intrinsic region generates electron–hole pairs, most of which contribute to a photocurrent. The photocurrent can be quite precisely proportional to the absorbed (or incident) light intensity over a wide range of optical powers. Figure 1: Schematic drawing of a p–i–n photodiode. The green layer is an anti-reflection coating. Figure 1 schematically shows the typical design of the photodiode on p–i–n type. Here, one has an intrinsic region between an n-doped and a p-doped region, where most of the electric carriers are generated. Through the electrical contacts (anode and cathode), the generated photocurrent can be obtained. The anode may have a ring shape, so that the light can be injected through the hole. A large active area can be obtained with a correspondingly large ring, but that tends to increase the capacitance, thus reducing the detection bandwidth, and increases the dark current; also, the efficiency may drop if carriers are generated too far from the electrodes. Photodiodes can be operated in two very different modes: Photovoltaic mode: like a solar cell, the illuminated photodiode generates a voltage which can be measured. However, the dependence of this voltage on the light power is nonlinear and the dynamic range is fairly small. Also, the maximum speed is not achieved. Figure 2: Current-voltage characteristics of a photodiode in photovoltaic mode for different load resistors. Photoconductive mode: here, a reverse voltage is applied to the diode and measures the resulting photocurrent. The simplest solution for that reversed-biased mode is based on a voltage source and a load resistor, as shown in Figure 3. The dependence of the photocurrent on the light power can be very linear over six or more orders of magnitude of the light power, e.g. in a range from a few nanowatts to tens of milliwatts for a silicon p–i–n photodiode with an active area of a few mm2. The magnitude of the reverse voltage has nearly no influence on the photocurrent and some influence on the dark current. A higher reverse voltage tends to make the response faster but also increases the heating of the device, which can be a problem for high photocurrents. Figure 3: A simple electronic circuit for a photodetector based on a photodiode. Figure 4: Current-voltage characteristics of a photodiode for different optical powers. Applications of Photodetectors: In radiometry and photometry: can be used for measuring properties like optical power, luminous flux, optical intensity and irradiance, in conjunction with additional means also for properties like the radiance. They are used to measure optical powers e.g. in spectrometers, light barriers, optical data storage devices, beam profilers, fluorescence microscopes, interferometers and various types of optical sensors. Particularly sensitive photodetectors are required for laser rangefinders, LIDAR, quantum optics experiments and night vision devices. Fast photodetectors are used for optical fiber communications, optical frequency metrology and for the characterization of pulsed lasers or laser noise. Two-dimensional arrays containing many identical photodetectors are used as focal plane arrays, mostly for imaging applications. For example, most cameras contain such devices as image sensors.