Biol 266 - Cell Biology Unit 2 PDF
Document Details
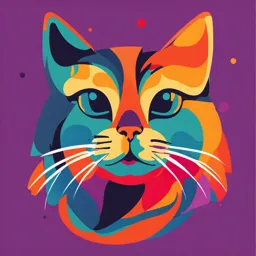
Uploaded by SuppleRosemary9790
Tags
Related
Summary
This document details cell biology unit 2, focusing on the study of organelles, their fractionation, and analysis of their biochemical functions. It covers different solutions affecting cell structure and function as well as techniques for understanding cell machinery including methods like immunoprecipitation, and affinity chromatography.
Full Transcript
Biol 266 – Cell Biology UNIT 2 “How cells are studied - II” To address many of the questions concerning the function of organelles in the cell, it is necessary to isolate organelles of eukaryotic cells in a form that can be used for biochemical studies Subcellular fractionation the separation of...
Biol 266 – Cell Biology UNIT 2 “How cells are studied - II” To address many of the questions concerning the function of organelles in the cell, it is necessary to isolate organelles of eukaryotic cells in a form that can be used for biochemical studies Subcellular fractionation the separation of the cell into its functioning organelles or isolation (purification) of individual organelles from the cell Golgi Plasma membrane Subcellular Lysosomes fractionation (vacuoles) Mitochondria Nucleus Peroxisomes ER Cell Study: 1) protein composition 2) lipid composition 3) metabolism (biochemical reactions) The initial step in subcellular fractionation is the disruption of the plasma membrane and the cell wall (if present) under conditions that do not destroy organelles The cells are suspended in an isotonic solution of appropriate pH (pH ~ 7.5) and salt content cell Isotonic solution has a salt concentration (150 mM) and a water concentration equal to that of the cell interior Water flows across the plasma membrane from a solution of high water (low salt) to one of low water (high salt) concentration until the water concentration on both sides is equal When cells are placed in a hypotonic solution (i.e. one with a lower salt concentration than that of the cell interior), water flows into the cells until the ion concentration inside and outside the cell is the same. Thus, the cells swell (the organelles also swell). Water Salt concentration Salt concentration inside decreases, inside = 150 mM i.e. < 150 mM (=100 mM) cell Hypotonic solution: Becoming an Isotonic solution: Salt concentration = 50 mM Salt concentration = 100 mM When cells are placed in a hypertonic solution (i.e. one with a higher salt concentration than that of the cell interior), water flows out of cells until the ion concentration inside and outside the cell is the same. Thus, the cells shrink (the organelles also shrink). Water Salt concentration Salt concentration inside inside = 150 mM increases, i.e. >150 mM (=200 mM) cell Hypertonic solution: Becoming an Isotonic solution: Salt concentration = 250 mM Salt concentration = 200 mM When cells are placed in an isotonic solution, there is no net movement of water in and out of cells. Thus, the cells and organelles do not swell or shrink For this reason, an isotonic solution is best for preserving the normal structure and function of organelles cell Isotonic solution has a salt concentration (150 mM) and a water concentration equal to that of the cell interior The initial step in subcellular fractionation is the disruption of the plasma membrane and the cell wall (if present) under conditions that do not destroy organelles 1. Conditions − Isotonic solution Homogenizer: The cells ( ) are forced through a very − On ice (0°C) to preserve organelles, proteins narrow space between the and other constituents plunger and the vessel wall 2. Methods vessel − Stirring the cell suspension in a high-speed wall blender − Sonication (exposure to high-frequency sound) − Grinding in a mechanical homogenizer plunger narrow space The cell disruption procedures: 1. Break the plasma membrane and the membrane of the ER into small fragments that immediately reseal to form small closed vesicles (the vesicles derived from the ER are called microsomes) 2. Leave other organelles (nuclei, mitochondria, Golgi, chloroplasts, lysosomes and peroxisomes) intact Cell Cell disruption in an isotonic Golgi Plasma solution on ice membrane Lysosomes (vacuoles) Mitochondria Nucleus Plasma ER Peroxisomes Microsomes (ER) membrane Salts: KCl, NaCl etc. The resulting suspension of broken cells is called a homogenate, extract or lysate Most subcellular fractionation procedures begin with differential centrifugation at increasingly high speeds The centrifugal force causes organelles, microsomes and plasma membrane vesicles to move toward the bottom of the centrifuge tube and form a pellet (sedimentation) at a rate that depends on their size and density. The largest and the heaviest organelles and vesicles sediment most rapidly. Nucleus (largest Golgi Plasma and most membrane dense Lysosomes (vacuoles) Golgi, lysosomes, Mitochondria Nucleus mitochondria and peroxisomes (intermediate sizes Peroxisomes Microsomes (ER) and densities Microsomes (ER) and plasma membrane vesicles (smallest and least dense) Ultracentrifuge 1. Consists of a rotor housed in a refrigerated chamber 2. The rotor is rotated at high speed by an electric motor 3. The rotor holds tubes that contain homogenates for fractionation 4. The ultracentrifuge is equipped with a vacuum system to reduce friction between the rotor and air. The vacuum prevents heating of the rotor and allows the refrigeration system to maintain the sample at 4oC 5. Some ultracentrifuges reach speeds over 200,000 rpm, subjecting samples to forces up to 500,000 times greater than gravity (g) 6. The centrifugal force causes organelles to move toward the bottom of the centrifuge tube and form a pellet (a process called sedimentation) Only nuclei sediment during centrifugation for 10 min at 1,000 x g, as they are the largest and the most dense organelles All other organelles remain in the supernatant (the so- called post- nuclear supernatant) 1,000 x g (1,000 The supernatant is poured off into a new times centrifuge tube and then returned to the gravity) centrifuge and subjected to greater centrifugal for 10 min force to obtain the next pellet The pellet (purified nuclei) is used for biochemical Post nuclear studies or electron microscopy supernatant Pellet (Nuclei) Mitochondria, peroxisomes, lysosomes and Golgi are organelles with intermediate sizes and densities These four organelles sediment during centrifugation for 20 min at 20,000 x g Microsomes (ER) and plasma membrane vesicles (the 20,000 x g (20,000 smallest and the least dense vesicles) remain in the times supernatant gravity) for 20 min The supernatant is poured off into a new centrifuge tube and then returned to the Supernatant centrifuge and subjected to greater centrifugal force to obtain the next pellet The pellet is resuspended in a buffer and subjected to equilibrium density- gradient Pellet (Mitochondria, centrifugation to separate mitochondria, peroxisomes, lysosomes peroxisomes, lysosomes and Golgi from each and Golgi ) other Microsomes (ER) and plasma membrane vesicles are the smallest and the least dense vesicles These vesicles sediment during centrifugation for 1 hour at 100,000 x g 100,000 x g (100,000 times Only the cytosol – the soluble aqueous portion of gravity) for the cytoplasm – remains undeposited after 1 hour centrifugation for 1 hour at 100,000 x g Supernatant The pellet is resuspended in a buffer and (the cytosol) subjected to equilibrium density- gradient centrifugation to separate microsomes (ER) and plasma membrane vesicles from each other Pellet (Microsomes (ER) and plasma membrane vesicles) Pour out: Pour out: Pour out: 1,000g 20,000g 100,000g 300,000g 10 min 20 min 60 min 120 min Differential centrifugation does not yield totally pure organelles One method for further purifying organelles is equilibrium density-gradient centrifugation The pellet is resuspended in a buffer and subjected to equilibrium density- gradient centrifugation to separate mitochondria, peroxisomes, lysosomes and Golgi from each other A 20,000-g pellet (Mitochondria, peroxisomes, lysosomes and Golgi) The pellet is resuspended in a buffer and subjected to equilibrium density- gradient centrifugation to separate microsomes (ER) and plasma membrane vesicles from each other A 100,000-g pellet (Microsomes (ER) and plasma membrane vesicles) Equilibrium density-gradient centrifugation is used to separate organelles on the basis of their density, independently of their size and shape Equilibrium density-gradient centrifugation involves a gradient of solute that increases in concentration and density from the top of the tube to the bottom The density of solute gradient spans the range of densities of the organelles to be separated For organelles, a gradient of sucrose is used The density range of sucrose is 10 % sucrose 1.10 – 1.30 g/cm3 (= 0.75 – 2.3 M (1.10 g/cm3) or 10 – 50 % [w/w] sucrose) 50 % sucrose (1.30 g/cm3) Densities of organelles: Peroxisomes = 1.23 g/cm3 Microsomes of the rough ER = 1.20 g/cm3 Mitochondria = 1.18 g/cm3 Golgi = 1.14 g/cm3 Plasma membrane vesicles = 1.12 g/cm3 Lysosomes (Vacuoles) = 1.10 g/cm3 10 % sucrose (1.10 g/cm3) 50 % sucrose (1.30 g/cm3) First step of equilibrium density- gradient centrifugation: Supernatant Pellet (Mitochondria, peroxisomes, lysosomes and Golgi ) The pellet is resuspended in a buffer 10 % sucrose and layered on a density (1.10 g/cm3) gradient composed of layers of increasingly more dense sucrose solutions in a centrifuge tube 50 % sucrose (1.30 g/cm3) Second step of equilibrium density-gradient centrifugation The tube is centrifuged for 6-16 hours at 100,000 x g During centrifugation, each organelle migrates to an equilibrium position where the density of the surrounding sucrose is exactly equal to the density of the organelle All the organelles reach their characteristic equilibrium position in the gradient and remain there Lysosomes (Vacuoles) = 1.10 g/cm3 Golgi = 1.14 g/cm3 Centrifugation for 6-16 hours Mitochondria = 1.18 g/cm3 at 100,000 x g Peroxisomes = 1.23 g/cm3 Second step of equilibrium density-gradient centrifugation When the organelles are at their equilibrium positions, the centrifuge is stopped The bottom of the tube is punctured, and the fractions are collected. Because of their different densities, the four types of organelles are collected in different fractions bottom top Fraction #1 Fraction #2 Fraction #3 Fraction #4 Since each organelle has unique morphological features, the purity of organelle preparations can be assessed by examination in an electron microscope or biochemically for enzymatic activity. electron microscopy, biochemistry Proteins in a cell extract (cytosolic proteins) or from purified organelles can be examined by enzymatic assays or a technique called western blot analysis. Enzymatic assays catalase, uricase – peroxisomes cytochrome c oxidase, succinate dehydrogenase – mitochondria acid phosphatase – lysosomes glucose-6-phosphatase – endoplasmic reticulum UDP-galactosyl transferase – Golgi 5’ nucleotidase - plasma membrane Some of these assays were used by Christian de Duve in his pioneering work on cell fractionation (Nobel Prize in Physiology or Medicine, 1974) Western analysis Western analysis (western blotting) is a method used to detect a specific protein in a sample that contains many proteins. It can be used, for example, to assess the purity of an organelle following purification. 1. Separate proteins on a polyacrylamide gel 2. Transfer the proteins onto a thin membrane 3. Probe the membrane with a specific antibody 4. Detect the antibody Polyacrylamide gel electrophoresis Without denaturing detergent – native gel electrophoresis, keeps protein complexes intact, migration in an electric field is dependent upon size, shape and charge (determined by amino acid composition) With denaturing detergent (sodium dodecyl sulfate (SDS) – denatures proteins so that they are no longer part of a protein complex. The negatively-charged SDS binds uniformly to proteins (1.4g SDS/g protein). Therefore, all proteins have a similar charge density and migration in an electric field is strictly dependent upon size. Larger proteins migrate through the polyacrylamide gel more slowly while smaller proteins migrate more quickly. A reducing agent (b-mercaptoethanol or dithiothreitol (DTT)) is included to break disulfide bonds. 1 Proteins are transferred 2 An antibody (primary from the SDS- antibody) to a specific polyacrylamide gel onto a protein is added to a thin membrane solution bathing the (nitrocellulose, PVDF). membrane. 3 An antibody (secondary antibody) 4 A solution containing a that binds to the primary substrate for the enzyme antibody is added to the solution linked to the secondary bathing the membrane. The antibody is added to the secondary antibody is linked to an solution bathing the enzyme that produces colour or membrane. If light is light. Like fluorescence produced it can be microscopy, this amplifies the detected using X-ray film. signal. Proteins can be separated from other cellular proteins using chromatography procedures such as ion-exchange, size exclusion (gel filtration) and affinity chromatography. All three chromatography procedures use western analysis to detect specific proteins of interest. 1. Ion exchange chromatography – separates proteins based on charge. The overall charge of a protein is the sum of all of the charges on the amino acids at a particular pH. When these charges are equal this is called the isoelectric point. At pH > isoelectric point proteins have a net negative charge, at pH < isoelectric point they have a net positive charge. Proteins in the mixture interact with a charged bead and are eluted using a salt gradient of increasing concentration. 2. Size exclusion (gel filtration) chromatography – separates proteins based on molecular size. Can distinguish between monomeric and higher- ordered structures. If the protein (e.g. 80kDa) is part of a large complex, it will fractionate at a size greater than 80kDa (on a denaturing SDS-gel it will fractionate at 80kDa). Proteins in the mixture pass through porous beads. Smaller proteins can access the pores more readily than larger proteins. Thus, large proteins pass through the entire column length faster and emerge first, the smaller proteins emerge last. 3. Affinity chromatography – separates proteins based on a specific sequence within the protein. The column has a ligand (e.g. substrate for a specific enzyme, antibody to a specific antigen, metal such as Ni2+ that binds to a stretch of histidine residues) bound to it that interacts with this sequence. Proteins in the mixture pass through the column. Most flow right through. But the specific protein is attracted to the ligand and is retained. The specific protein is then eluted by adding an excess of soluble ligand or some other molecule to break the interaction. Near total purification in a single step. Protein interactions can be studies by a number of methods including: 1. Immunoprecipitation 2. Yeast two-hybrid 3. FRET 1. Immunoprecipitation – variation of affinity chromatography using antibodies. Instead of packing a column, small amounts of beads with antibodies are incubated with a cellular extract under non-denaturing conditions. The antibody binds to its antigen (protein X) and this immune complex is collected by centrifugation (the relatively large beads pellet, the supernatant contains the cellular extract). The only protein expected to pellet with the beads is protein X. If other proteins are detected, this indicates they interact with protein X. Western analysis or mass spectrometry can be used to detect co-immunoprecipitating proteins. 2. Yeast two-hybrid – reconstitution of a transcription factor by the interacting proteins leads to expression of a reporter gene (e.g. lacZ = b-galactosidase) whose activity can be easily measured. (A) Transcription factor from yeast has two domains: DNA binding domain and transcriptional activation domain. Using molecular biological techniques, the DNA encoding each domain is linked to the DNA encoding two different proteins (bait and fish). The bait is linked to the DNA binding domain (B) and the fish to the transcriptional activation domain (C). A B C 2. Yeast two-hybrid – reconstitution of a transcription factor by the interacting proteins leads to expression of a reporter gene (e.g. lacZ = b-galactosidase) whose activity can be easily measured. If the two hybrid proteins interact (D), the transcription factor activity will be reconstituted and transcription of lacZ will take place. The lacZ protein (b-galactosidase) will be produced and will function (in the presence of the appropriate substrate it will generate a blue pigment). If the two hybrid proteins do not interact, transcription of lacZ will not occur (E). D E 3. FRET (fluorescence resonance energy transfer) – can be used to examine protein-protein interactions within a cell. Protein Z is fused to CFP and protein Y is fused to YFP. lex and lem of CFP: 440 nm and 480 nm lex and lem of YFP: 514 nm and 530 nm (A) If the proteins do not interact, CFP absorbs the light at 440 nm and emits strongly 480 nm. Little emission at 530 nm is observed. (B) If the proteins do interact, CFP transfers some of the emitted energy to YFP which then emits strongly at 530 nm. Therefore, the cells are irradiated at 440 nm and the detector is set to detect light at 530 nm, indicating an interaction between the proteins. The two proteins must be within 5 nm for FRET to occur. A B 480 nm 530 nm 440 nm 440 nm 530 nm central dogma of molecular biology DNA mRNA protein degraded protein DNA X mRNA protein degraded protein Gene silencing – RNA interference Assessing the function of a protein is facilitated by examining the effects of removing that protein from the cell. One such method is called RNA interference (RNAi). Double-stranded RNA related to a double stranded RNA specific mRNA encoding the protein dicer of interest is cleaved into small (~21bp) fragments by an enzyme siRNA called dicer. This RNA can be generated from a DNA plasmid RISC after it is transfected into a cell. Can be propagated in the laboratory. Antisense Complementary strand/RISC The resulting pieces of RNA are strand degradation called small interfering RNA mRNA cleavage (siRNA). This type of RNA can be mRNA purchased from a company and transfected into a cell. Cannot be propagated in the laboratory. Gene silencing – RNA interference Assessing the function of a protein is facilitated by examining the effects of removing that protein from the cell. One such method is called RNA interference (RNAi). The antisense strand associates double stranded RNA with a protein complex called RISC. dicer The complementary strand is degraded. siRNA RISC Antisense Complementary strand/RISC The antisense strand/RISC complex strand degradation then anneals with the mRNA mRNA cleavage encoding the protein of interest and mRNA cleavage of the mRNA ensues. Argonaute, a component of RISC, facilitates the annealing of the small RNA fragment to the mRNA. central dogma of molecular biology DNA mRNA protein degraded protein DNA X mRNA protein degraded protein After hours or days (depending on protein stability), the protein is depleted and there is no mRNA present to replenish it. One can monitor the degree of depletion (knockdown) by western analysis. siRNA - + TrappC2 16 kDa tubulin 50 kDa The effect of an Aurora B knockdown on mitotic cells