Refrigeration Basics PDF
Document Details
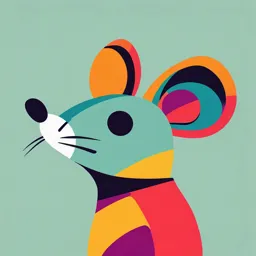
Uploaded by WellBredDerivative9571
Lakeland College
Tags
Summary
This document is a chapter on refrigeration basics. It introduces the fundamental concepts of refrigeration and the principles of a vapor compression refrigeration system. The chapter also covers details on calculating refrigeration system performance.
Full Transcript
# 4th Class Edition 3 • Part B • Unit 9 ## CHAPTER 1: Refrigeration Basics ### **Learning Outcome** When you complete this chapter you should be able to: - Explain the basic concept of refrigeration and refrigerants. ### **Learning Objectives** Here is what you should be able to do when you com...
# 4th Class Edition 3 • Part B • Unit 9 ## CHAPTER 1: Refrigeration Basics ### **Learning Outcome** When you complete this chapter you should be able to: - Explain the basic concept of refrigeration and refrigerants. ### **Learning Objectives** Here is what you should be able to do when you complete each objective: 1. Explain the fundamentals of refrigeration. 2. Describe the cycle of operations in a vapour compression refrigeration system. 3. Explain how the operating temperatures and pressures are selected and related for a vapour compression refrigeration system. 4. State how the capacity of a refrigeration system is described and how refrigeration tables are used to calculate system performance. 5. Describe how refrigerants are classified. 6. Describe the thermodynamic properties of refrigerants. 7. Describe the properties of refrigerants relating to miscibility, leakage tendency, odour, moisture reaction, toxicity, and flammability. ## Chapter Introduction One source describes refrigeration as "The branch of science that deals with the process of reducing and maintaining the temperature of a space or material below the temperature of the surroundings". Another source describes refrigeration as "The process of moving heat from one location to another by use of refrigerant in a closed cycle". Combining these two results in a more comprehensive definition: Refrigeration is the thermodynamic process of moving heat from one location to another, for the purpose of reducing and maintaining the temperature of a material below the temperature of its surroundings, through the circulation of refrigerant in a closed cycle. This material will deal with the science and theory of refrigeration, by introducing the principle components of a refrigeration system, and describing the role and interaction of these components. Prior to studying this material, it is advisable to review Part B Unit 2 Introduction to Pumps and Compressors, especially Chapter 3 Introduction to Compressors. ## Objective 1: Explain the fundamentals of refrigeration. ### **Evaporation** From thermodynamics, recall that one kilogram of pure boiling water, at 101.3 kPa absolute pressure, absorbs 2257 kJ of latent heat. For water, this is the amount of energy needed, per kg, to change from its liquid to gaseous state. For water to evaporate, heat must be transferred to it. The heat source, when giving off heat energy, may: - Undergo a temperature drop (sensible heat), or - Undergo a change of state (latent heat). Another word for "state" is "phase". Change of state is also called "phase change". This chapter uses the terms "phase change" and "change of state" interchangeably. Consider the first point. Most everyone has stepped out of a shower, pool, or bathtub, and shivered vigorously until dry. While shivering, every kilogram of surface water that evaporated withdrew 2257 kJ of heat, resulting in a cold feeling. The evaporative process that occurred reduced the body temperature. Therefore, the first fundamental principle of refrigeration is that a substance must absorb or reject latent heat in order to change state. When latent heat is added to a liquid, it evaporates. When latent heat is removed from a gas, it condenses. The evaporative process removes heat, and causes a cooling effect. Consider the second point. It is possible for a liquid refrigerant to boil below the freezing point of water. If a body of water has its heat continually drawn away by this refrigerant, the water will first drop in temperature, and then change in state from liquid to solid. First, the refrigerant removes sensible heat from the water, and then it removes latent heat of fusion. This occurs each time an ice-cube tray is placed into a freezer. Therefore, the evaporation of a liquid can lower a body's temperature by the extraction of sensible heat, and the evaporation process can cause a change in state (if the liquid evaporation occurs at a low enough temperature). Let us now look at the effect of pressure on a boiling liquid. Pure water, at 101.3 kPa absolute pressure (zero gauge pressure), will boil at 100°C. In a boiler though, due to the higher pressure exerted on the surface of the water, boiling occurs at a higher temperature. For example, at 200 kPa absolute, pure water boils at 120.21°C. At higher altitudes, where the atmospheric pressure is lower, pure water boils at temperatures below 100°C. For example, in Lake Louise, Alberta, the elevation is 1731 metres above sea level. At this elevation, the air pressure is around 83 kPa absolute, and water boils at 94.48°C. Therefore, the second fundamental principle of refrigeration is that the pressure exerted on the surface of a boiling liquid affects the temperature at which the liquid boils. ## Objective 2: Describe the cycle of operations in a vapour compression refrigeration system. ### **Actual Refrigeration Systems** Actual refrigeration systems are not like those described in Objective 1. Additional equipment must be added to address the significant shortcomings of the simple systems of the previous objective. First, in order to run continuously, the vaporized refrigerant must be continuously fed to replace the refrigerant boiled in the evaporator. The rate of replacement must be the same as the rate of evaporation. To do this, larger refrigeration systems have a reservoir of refrigerant (the liquid receiver) to supply liquid to the evaporator. A metering device (such as an adjustable valve, or non-adjustable restriction) must be installed between the liquid receiver and the evaporator, to control the refrigerant flow. If too much refrigerant enters the evaporator, both vapour and un-boiled liquid will leave the evaporator. This liquid will cause damage to the compressor (which will be discussed later). If fed too little refrigerant, the evaporator will "starve" or "run dry". With insufficient boiling liquid, heat flow to the evaporator will be reduced, thus reducing the refrigerating effect. Secondly, refrigerant vapour must be recovered and re-used. In actual refrigeration systems, the refrigerant vapour that leaves the evaporator is compressed, and then cooled in a heat exchanger. In this exchanger, called the condenser, the vapour gives off latent heat to a coolant (or cooling medium) and returns to its liquid state. The liquefied refrigerant then flows from the condenser to the liquid receiver where it resides until it is re-used in the evaporator. Finally, a refrigeration system needs a compressor. To get refrigerant vapour to release its latent heat, the vapour must be hotter than the cooling medium. Consider Figure 1. The refrigerant vapour leaving the evaporator is at its saturation temperature (-33.3°C). To condense the vapour, it would need to be exposed to a cooling medium colder than -33.3°C. However, most condensers use relatively warm cooling media (between 0 and 40°C, depending on the source). Therefore: - The temperature of the refrigerant vapour must be made higher than the temperature of the cooling media (typically, higher than 40°C); and - The pressure of the refrigerant vapour must be raised to a point where the condensed liquid refrigerant - now at high temperature - will remain a liquid. In other words, the saturation pressure in the condenser must correspond with the high saturation temperature of the condensed liquid. The compressor accomplishes both of these tasks. The compressor in a refrigeration system takes the place of the vacuum pump shown in Figure 3. A compressor draws refrigerant vapour at a low pressure and discharges it at a higher pressure. Compression is work, and requires the expenditure of mechanical energy (W = F x d). The mechanical energy of compression is converted to potential energy (pressure) and internal energy (vapour temperature). Therefore, in compressing the low-pressure refrigerant vapour, the compressor also raises the refrigerant vapour temperature. The pressurized, high temperature vapour then enters the condenser, transfers heat to the surrounding coolant, and condenses. The refrigerant liquid drains to the receiver, and eventually returns to the evaporator, where the cycle repeats. Figure 4 shows an actual refrigeration system with the following mandatory components: - Refrigerant - An evaporator - A refrigerant metering device (control valve, orifice or capillary tube) - A condenser - A compressor Optional components shown in Figure 4 include: - A liquid receiver - A condenser cooling fan - An evaporator fan This simple but complete system is typical of a household refrigerator, freezer, or air-conditioning system. Such a system transfers heat from a low temperature region (such as the inside of a refrigerator) to higher temperature condensing medium, such as air or water. This system is called a vapour compression refrigeration system. The evaporator and condenser pressures, maintained by the compressor and metering device, are essential for achieving proper system temperatures. ## Objective 3: Explain how the operating temperatures and pressures are selected and related for a vapour compression refrigeration system. Figure 10 shows a compression refrigeration system using R-134a as the refrigerant. This system chills water to cool the air in a central air conditioning system. The evaporator is a shell-and-tube heat exchanger. The chilled water flows through the tubes and the liquid refrigerant surrounds the tubes. The condenser is water-cooled. The pressures and temperatures in this refrigeration system are determined by the temperature required for the air leaving the cooling coil in the air duct - in this case 12°C. The average temperature of the air passing through the coil is approximately 15°C. Assume that the cooling coil is designed for a temperature difference of 10°C between the average air temperature and the average chilled water temperature. The average temperature of the chilled water must be 5°C. Since the water leaves the cooling coil at 10°C, it should be therefore be supplied at 6°C to provide an average temperature of 5°C. From the above, it follows that the water enters the evaporator at 10°C and is cooled to 6°C. Again, assume that the evaporator is designed for a temperature difference of 10°C between the average chilled water temperature (5°C) and the temperature of the boiling refrigerant. It is not practical to operate the evaporator below freezing, so 0°C is the lowest temperature at which the evaporator can operate. This requires an evaporator pressure of 292.5 kPa absolute pressure (394.1 kPa_g_). The cooling water enters the condenser at 15°C and leaves at 26°C, giving an average cooling water temperature of 22°C. Assuming the condenser is designed for a temperature drop of 5°C, the condensing temperature of the refrigerant vapour must be 5°C higher than the average cooling water temperature. Therefore, the condensing temperature will be 30°C. This requires a condenser pressure of 770 kPa absolute (871.3 kPa_g_). ## Objective 4: State how the capacity of a refrigeration system is described and how refrigeration tables are used to calculate system performance. ### **Refrigerant Tables** Certain properties of refrigerants such as the saturation temperatures and pressures, volume, density and enthalpy are called thermodynamic properties of refrigerants. These values must be known in order to solve capacity and performance problems. These thermodynamic properties have been obtained by careful experiments. The resulting values have been tabulated in the refrigerant tables. Refer to Table I- Refrigerant R-717 (Ammonia): Saturation Properties (Temperature) in the PanGlobal Academic Supplement. This table lists the thermodynamic properties of ammonia at various saturation temperatures. Part of this table is shown below in Table 3. | Saturation Temperature (°C) | Saturation Pressure (kPa) | Volume Vapour (m3/kg) | Density Liquid (kg/m3) | Enthalpy, kJ/kg | Entropy, kJ | | -- | -- | -- | -- | -- | -- | | -5 | 354.76 | 0.34664 | 645.37 | 157.77 | 1279.73 | 1437.5 | 0.62848 | | -4 | 368.8 | 0.33414 | 644.02 | 162.37 | 1276.23 | 1438.6 | 0.64553 | | -3 | 383.27 | 0.32218 | 642.66 | 166.98 | 1272.82 | 1439.8 | 0.66253 | | -2 | 398.19 | 0.31074 | 641.3 | 171.59 | 1269.31 | 1440.9 | 0.67949 | | -1 | 413.56 | 0.29979 | 639.94 | 176.21 | 1265.79 | 1442 | 0.6964 | Consider the columns as numbered from left to right. Column 1 lists the saturation temperature in °C for each absolute pressure. Column 2 lists the corresponding saturation pressure in kPa (absolute). It is obvious, by examining the values in columns 1 and 2 that the temperature increases as the pressure increases. Column 3 lists the specific volume (Vg) in m3/kg of refrigerant vapour. Note that the specific volume of the vapour decreases as the pressure increases. Column 4 lists the density (1!Vf) in kg/m3 of refrigerant liquid. Column 5 lists the enthalpy (hf) in kJ/kg of saturated liquid refrigerant, for each temperature. Column 6 lists the enthalpy of evaporation (hfg) in kJ/kg of refrigerant. Column 7 lists the enthalpy (hg) in kJ/kg of saturated refrigerant gas, for each temperature. Columns 8 and 9 list values of entropy, which are used for calculations beyond the scope of this chapter. ### **Refrigeration System Capacity** Internal combustion engines are compared according to kilowatt output. Boilers are compared according to boiler horsepower. Refrigeration plants are compared according to tonnes of refrigeration (or in United States Customary System (USCS) units, tons of refrigeration). This text abbreviates the SI unit "tonne of refrigeration" with the letters "TR". This must not be confused with the USCS "ton of refrigeration," which, if used in the learning materials, will not be abbreviated. A boiler horsepower is the amount of heat required to produce 15.68 kg (34.5 lbs.) of dry and saturated steam at 100°C (212°F) from pure feedwater at 100°C (212°F), over a period of one hour. This calculates to a heat output rate of 35 394 kJ per hour (33 475 Btu/hr). However, no boilers actually produce steam under such conditions. Despite this, the concept of boiler horsepower remains a useful way to compare the heat output of various boilers. A similar situation applies to the concept of "tonnes of refrigeration:' A tonne of refrigeration is the amount of heat required to produce 1 tonne of ice at 0°C from pure water at 0°C, over a 24-hour period. In other words, one tonne of refrigeration is the amount of latent heat of fusion that must be extracted from 1 tonne of water at 0°C to turn it into ice at 0°C. No refrigeration systems operate under such conditions; however, the concept of tonnes of refrigeration is a useful way to compare the heat transfer rate of various refrigeration plants. ### **SI Tonne of Refrigeration (TR)** A tonne of refrigeration is a heat rate based on the latent heat of fusion of pure water, which is 335 kJ/kg. Consequently, to produce 1 tonne of ice at 0°C from water at 0°C would require: ```text 1 TR = 1 tonne x 1000 kg x 335 kJ/kg day tonne kg = 335 000 kJ/day ``` As a heat rate, a tonne of refrigeration can be expressed over different time spans. So, one TR can also be expressed as a heat rate per hour, a heat rate per minute, or a heat rate per second. ```text 1 TR = 335 000 kJ x 1 day = 13 958 kJ/hour day 24 hours 1 TR = 335 000 kJ x 1 day x 1 hour = 232.6 kJ/min day 24 hours 60 min 1 TR = 335 000 kJ x 1 day x 1 hour x 1 min = 3.877 kJ/s day 24 hours 60 min 60 s ``` Note that the last conversion to kJ/s can be expressed as 1 eW So, one TR is equal to 3.877 kW. ## Objective 5: Describe how refrigerants are classified. ### **Refrigerant Identification and Classification** Of the hundreds of refrigerants available, only a few are widely used in modern residential, commercial, industrial, and institutional refrigerating and air conditioning systems. It is important to know the chemical and physical properties of refrigerants to permit their comparison. As well, the proper refrigerant must be selected, ordered, and used for particular applications. Therefore, it is important to be able to refer to refrigerants consistently, so they may be properly identified. These named refrigerants are categorized according to flammability and toxicity, and then placed in a safety group. Refrigerants are also classified according to their impact on the environment, their chemical origins, and their operating temperature suitability. ### **ASHRAE Designation** Table 4 shows the various ways to identify refrigerants, including: - ASHRAE designation - Chemical formula - Chemical name - Trade name Table 4 lists common refrigerants designated by ASHRAE. ASHRAE denotes refrigerants with the capital letter "R" followed by a dash and a number. There are well over 300 ASHRAE designated refrigerants and refrigerant blends. Many of these refrigerants are used only in very small amounts, in special laboratory equipment. ## Objective 6: Describe the thermodynamic properties of refrigerants. ### **Thermodynamic Properties of Refrigerants** Thermodynamic properties are the physical properties that directly affect the movement of heat. These properties are pressure, temperature, volume, density, enthalpy, and entropy. The PanGlobal Academic Supplement has tables and charts for the thermodynamic properties of R-717, R-134a, R-123 and R-744. Refer to the Academic Supplement while studying this objective. A discussion of the various properties of refrigerants follows. As each property is discussed and a comparison is made between the properties of different refrigerants, it will become obvious how much refrigerants differ. Because of these differences, no single refrigerant is ideal. The differences in properties, however, make one refrigerant more suitable for an application than another. ### **Refrigerant Properties at Standard Operating Conditions** Table 6 shows the boiling point at atmospheric pressure (101.3 kPa) of the refrigerants listed in Table 1. Note that some refrigerants boil well below 0°C, the freezing point of water, while others boil above 0°C. Table 6 also compares the thermodynamic properties of these common refrigerants, at standard operating conditions of -15°C evaporator temperature and +30°C condenser temperature. | | R-134a | Suva 134a | R-123 | Suva 123 | R-717 (Ammonia) | |---------------|--------|-----------|-------|----------|----------------------| | Boiling point at 101.3 kPa absolute | -26.1 | 27.7 | 15.7 | 110 | 2 | | Evaporating pressure (kPa abs.) at -15°C | 163.9 | 15.7 | 2 | 2.3 | - | | Condensing Pressure (kPa abs.) at +30°C | 770 | 110 | - | - | - | | NRE at standard conditions (kJ/kg) | 147.9 | 142.2 | 1102 | - | - | | Specific volume of vapour (m3/kg) at -15°C | 0.1207 | 0.8848 | 0.50 | - | - | | Density of liquid (kg/m3) at +30°C | 1187.5 | 1451 | 595 | - | - | Careful review of this table reveals significant differences in the properties of these refrigerants. ### **Pressure-Temperature Relationship** Note that R-123 boils at 27.7°C at atmospheric pressure. An R-123 chiller, then, must operate with its evaporator well below atmospheric pressure. In fact, at -15°C, the evaporator is at 15.7 kPa absolute pressure. This is the reason R-123 is termed a high temperature refrigerant: it is suitable for HVAC use, but not well suited for industrial cold storage freezers or ice malting. Because of the low evaporator pressure, air may leak into the refrigeration circuit; therefore, air purgers must be installed to continuously remove air from R-123 systems. Also, note the condensing pressures. R-123 has a low pressure at +30°C, so that the refrigeration equipment is considered low pressure by many jurisdictions, and may not require operation by Power Engineers. R-717 and R-134a both have high condensing pressures at 30°C: therefore, large plants using these refrigerants generally require full-time operation by Power Engineers. Note also that R-717 has the highest condensing pressure of the three listed. The high side equipment of R-717 systems (and R-134a systems) must be rugged and built according to ASME BPVC and ASMEB31.5. Table 6 compares how different refrigerants' operating pressures vary with temperature. This is important since it determines the strength of the equipment required, cost of construction, and operator staffing requirements, for a particular refrigerant. One application consideration when choosing a refrigerant is that it should have a low condensing pressure. As well, evaporators should preferably operate above atmospheric pressure to prevent air and moisture infiltration, whi<:;h causes operational problems. ### **Specific Volume** Table 6 gives the specific volumes (in m3/kg) of refrigerant vapour at -15°C. R-717 vapour has the lowest specific volume. This means that a refrigeration compressor in an R-717 system can have a smaller displacement than a compressor for R-123 or R-134a, in a system of similar capacity. As well, suction lines for an R-717 system can be made smaller in diameter, for a given mass of refrigerant circulated, than an R-123 or R-134a system. ### **Density** Refrigerant liquid density (in kg/m3) informs designers about how heavy liquid lines will be. The liquid refrigerant density must also be known to calculate of the size of control valves and piping. It can be seen from Table 5 that R-717 is not a very dense liquid in comparison to R-123 and R-134a. In fact, liquid ammonia is less dense than water. ### **Refrigerating Effect** Finally, examine the NRE of each refrigerant listed on Table 6. The NRE is the heat absorbed in the evaporator, under standard operating conditions. R-717 is the most effective at transferring heat in the evaporator. In fact, R-717 removes over 7 times the heat, per kg of refrigerant, than R-123 or R-134a. | Temp. (°C) | Pressure (kPa absolute) | Temp. (°F) | Pressure (psia) | |---|---|---|---| | -15 | 163.9 | -236.2 | 5 | 23.8 | | -10 | 200.6 | -290.7 | 15 | 29.7 | | -5 | 243.3 | -354.8 | 25 | 36.8 | | 0 | 292.8 | -429.4 | 35 | 45.1 | | 5 | 349.7 | -515.8 | 45 | 54.7 | | 10 | 414.6 | -615.1 | 55 | 65.9 | | 15 | 488.4 | -728.5 | 65 | 78.7 | | 20 | 571.7 | -857.5 | 75 | 93.4 | | 25 | 665.4 | -1003.2 | 85 | 109.9 | | 30 | 770.2 | -1167.2 | 95 | 128.7 | | | R-134a | R-123 | R-717 | |---------------|--------|-------|-------| | -15 | 20.2 | 2.3 | 34.3 | | -10 | 3.0 | 3.0 | 43.1 | | -5 | 4.0 | 4.0 | 53.7 | | 0 | 5.1 | 5.1 | 66.3 | | 5 | 6.5 | 6.5 | 81.0 | | 10 | 8.2 | 8.2 | 98.1 | | 15 | 10.3 | 10.3 | 117.9 | | 20 | 12.7 | 12.7 | 140.6 | | 25 | 15.6 | 15.6 | 166.5 | | 30 | 18.9 | 18.9 | 195.9 | The PanGlobal Academic Supplement refrigeration tables use the same abbreviations for enthalpy at various states as the Academic Supplement Steam Tables. "hf" is the enthalpy of saturated liquid. "hg" is the enthalpy of saturated vapour. "hfg" is the latent heat of evaporation of the refrigerant, which is equal to hg minus hf. Remember that these three properties are thermodynamic characteristics specific to every refrigerant, and vary with the refrigerant's pressure and temperature. The values in the refrigeration tables are used to determine net refrigerating effect, pressure ratio, and coefficient of performance. As well, the refrigeration tables provide the information necessary to calculate the work done in compression, heat rejected by the condenser, mass of refrigerant circulated per tonne of refrigeration, compressor displacement and others. Many more advanced calculations may be performed. These, however, are beyond the scope of Fourth Class Power Engineering. ## Objective 7: Describe the properties of refrigerants relating to miscibility, leakage tendency, odour, moisture reaction, toxicity, and flammability. ### **Physical Properties of Refrigerants** The important physical properties of a refrigerant are as follows: - Miscibility - Leakagetendency - Odour - Toxicity - Flammability/explosiveness - Moisture reaction | | R-134a (Suva 134a) | R-123 (Suva 123) | R-717 (Ammonia) | |-----------------|---------------------|---------------------|---------------------| | Flammable | no | no | slightly | | Explosive when mixed with air | no | yes | yes | | Toxic | no | yes | yes | | Corrosive to metals in the presence of water | no | no | Corrosive to copper and copper alloys | | Effect of contact with food | none | none | Spoils taste, may cause toxicity | | Effect on lubricating oil | Incompatible with mineral oil. Miscible with Polyolester oil. | Moderately miscible with mineral oil. | Non-miscible with mineral and synthetic oil. | | Odour | Slightly sweet odour | Slight, ether-like odour | Strong and offensive | ### **Miscibility** Miscibility refers to when two or more liquids are soluble in all proportions. Some refrigerants are entirely miscible with lube oil. Some are partially miscible. Some are not miscible at all. Refrigerants come in contact with lube oil in the compressor crankcase, cylinder walls, and screws. Refrigerants carry some of this oil into other parts of the system. Miscibility is dependent on the type of refrigerant and the type of lube oil. Lube oils are categorized as conventional mineral-based oils and synthetic polyolester oils. Most halocarbon refrigerants are miscible with one or the other. Ammonia, however, is not miscible with lube oil. Oil-miscible refrigerants dilute compressor crankcase oil, lowering its viscosity and lubricating ability. Allowance should be made for this when selecting a lubricating oil. Oil carried into the condenser and evaporator coats the heat transfer surfaces. This tends to be an insulating coating, reducing evaporator and condenser capacity. When oil-miscible refrigerants are used, the refrigerant will dissolve most of the oil in the system components and return it to the compressor, thus avoiding problems with heat exchange or oil blockage. However, when non-miscible refrigerants are used, the oil tends to build up in the evaporator and the condenser. This reduces system capacity, and eventually blocks the evaporator tubing. Therefore, ammonia systems require oil separators and oil return systems. These remove oil from the piping and other low points in the system, and return the oil to the compressor. Figure 11 shows oil separators installed on the compressor discharge piping in an ammonia refrigeration plant. The miscibility of the refrigerant used in the refrigerating system plays an important role in system design, regarding the need for oil separators, and oil return lines. As well, miscibility affects the sizing of piping. Pipes are sized to maintain sufficiently high refrigerant velocity to prevent oil from settling in evaporator tubing. ### **Odour** In large concentrations, the refrigerants in the halocarbon group have a slight odour resembling ether. They are practically odourless in low concentrations. Other refrigerants such as ammonia have a strong, pungent smell. A slight odour may be advantageous since even small leaks are easily and quicldy detected, so that repairs can be made before all refrigerant is lost. A strong, pungent odour, even when escaping from a minor leak, will also be noticed quicldy. However, when even a small leak of ammonia is noticed in a place of public assembly, such as an arena, its smell may induce panic-like behaviour. ### **Flammability/Explosiveness** Flammable and explosive refrigerants require special consideration as to how and where they are employed. Typically, flammable and explosive refrigerants, such as R-290 (propane), are limited by CSA B52 code to industrial sites. These locations would likely employ full-time system supervision, and would have specialized explosion-proof electrical equipment (including explosion-proof light switches, lamps, heaters, transformers and motors). Of the refrigerants listed in Table 8, only ammonia supports combustion. It is explosive in concentrations between 15% and 28% by volume in air. ### **Toxicity** As previously mentioned, refrigerants are divided into groups according to toxicity. Although refrigerants in the chlorofluorocarbon group are not toxic except in high concentrations, it should be mentioned that, when exposed to a flame, they will react and decompose, forming highly toxic products. When a halide torch is used for leak detection, ample ventilation should be provided. **CAUTION**: - Even non-toxic refrigerants can kill. If a substantial leak occurs, the refrigerant vapour displaces air. Cold refrigerant vapour is usually denser than air. Pockets of refrigerant gas accumulate in low-lying areas. If these areas are not properly ventilated, persons entering these areas could die of asphyxiation. Therefore, all refrigeration plants must have appropriate leak detection systems, ventilation systems, and breathing apparatus. ### **Leakage Tendency** Many factors determine the leakage tendency of a refrigerant. These factors include operating pressure, viscosity, density, and the chemical effects on seals and gaskets. The leakage tendency also depends on the molecular mass of the refrigerant. The greater the molecular mass, the larger the molecule and the less able it is to escape through tiny openings. ### **Moisture Reaction** Moisture will combine in varying degrees with most of the commonly used refrigerants. CFCs, HFCs, and HCFCs absorb only small amounts of moisture. Ammonia, however, readily absorbs moisture. At high temperatures, refrigerants are able to absorb a greater percentage of moisture than at low temperatures. This means that when a warm moisture-saturated refrigerant is cooled to a lower temperature (for instance, in the evaporator), it will produce free water. Moisture in the refrigerant should be avoided for three reasons: 1. When present in the liquid refrigerant, moisture may cause ice to form between the valve and valve seat of the metering device when the liquid is reduced in pressure and temperature as it enters the evaporator. This causes improper operation of the metering device. 2. When present in the system, moisture may also cause acid formation, resulting in corrosion, sludge forming in the compressor crankcase, and deterioration of the electric motor insulation in hermetic compressors. 3. In ammonia refrigeration systems, ammonia combines with water to form ammonium hydroxide, which is highly corrosive to copper and its alloys. For this reason, ammonia systems must NEVER use components made of copper or copper alloys. ## Chapter Summary This chapter introduced basic refrigeration terminology and discussed mechanical refrigeration, from its fundamental principles. From these principles, an understanding of the vapour compression refrigeration process was developed. Refrigeration processes - both theoretical and actual - were illustrated with simple schematics and pressure-enthalpy diagrams. As well, refrigeration system capacity was both discussed and calculated, using SI and USCS units. The refrigeration tables and p-h diagrams were used to find information for calculations, including NRE. The concepts of"coefficient of performance" and "pressure ratio" were also introduced. The last three objectives examined refrigerants in details: their identification, their thermal properties, their physical properties, and their behaviour.